Mantoverde - Manto Verde, Manto Ruso, Manto Monstruo, Monte Cristo |
|
Chile |
Main commodities:
Cu Au
|
|
 |
|
 |
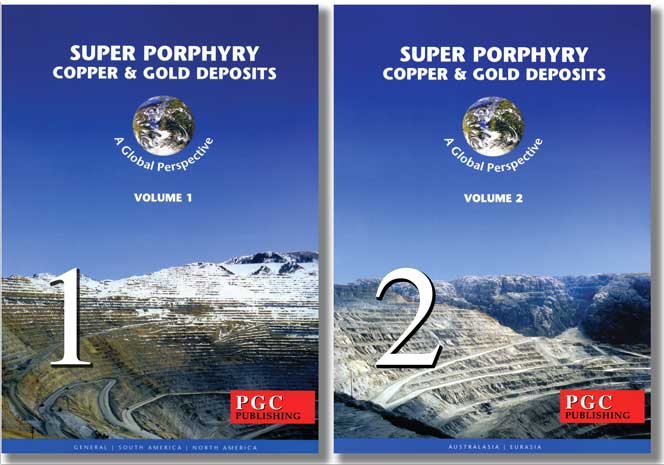 |
Super Porphyry Cu and Au

|
IOCG Deposits - 70 papers
|
All available as eBOOKS
Remaining HARD COPIES on
sale. No hard copy book more than AUD $44.00 (incl. GST) |
|
 |
The Mantoverde IOCG deposit cluster is located in the Los Pozos district of the Chilean Coastal Cordillera, some 400 km south of Antofagasta and 100 km north of the town of Copiapo in northern Chile. Individual deposits include Manto Verde, Manto Ruso, Manto Monstruo and Monte Cristo (#Location: 26° 33' 57"S, 70° 18' 45"W).
The Los Pozos district falls within a structural segment bound to the east and west by two branches of the north-south trending Atacama Fault Zone (AFZ), an arc-parallel wrench fault system that extends for more than 1000 km along the Chilean coast. The prominent 340 to 345° trending and 40 to 50°E-dipping brittle Manto Verde fault cuts this segment of the AFZ and controls the main Cu-Au ore zone.
The district is predominantly composed of Jurassic and/or possibly Early Cretaceous andesitic flows and breccias which are interpreted to correlate with either the Jurassic La Negra Formation or the Early Cretaceous Bandurrias Group. These cataclased andesitic volcanics host rocks are intruded by coeval (?) early Cretaceous granitoids of the Chilean Coastal Batholith. Granodiorites and monzonites of the ~130 Ma Las Tazas Plutonic Complex are found in the western part of the Manto Verde deposit, while diorites, monzodiorites, granodiorites and tonalites of the ~127 Ma Remolino Plutonic Complex lie to the east.
For detail of the regional setting of the Chilean Coastal Cordillera in the Central Andes, see the Central Andes and Bolivian Orocline record.
The Cu-Au ores of the Los Pozos district are hosted mainly in specularite-dominated tabular breccia bodies (Mantoverde), breccia pipes (Manto Ruso and Manto Monstruo) and stockwork bodies (Monte Cristo). All are associated brittle faults belonging to the AFZ, which were emplaced during an extensional phase of sinistral strike-slip and dip-slip tectonism of that complex Jurassic to early Cretaceous major regional fault system. The Mantoverde deposit consists of three breccia units paralleling the 12 km long Mantoverde Fault for at least 1500 m. The Mantoverde Fault which strikes NNW and dips at 40 to 50° E, connects two major branches of the AFZ.
The hangingwall Manto-Atacama breccia is a ±100 m wide, matrix supported, specularite rich, hydrothermal breccia that follows the Mantoverde Fault along strike and down dip although it thins with depth. The degree of brecciation and mineralisation decreases gradually outwards from the fault into the andesite wall rocks.
In contrast the footwall Manto Verde Breccia is a ±20 m wide cataclasite with relatively sharp mineralisation boundaries, with sulphides being hosted by andesitic volcanic and deformed volcaniclastic rocks where hypogene grades locally exceed 1% Cu with around 0.25 g/t Au and Elevated REE concentrations.
Other Cu-Au deposits also occur on more northwest-trending bends of this fault or the eastern branch of the Atacama fault zone, or at the intersection of the Manto Verde fault and related second order structures.
Unlike at Candelaria, early district-wide sodic-calcic alteration is absent in the Los Pozos district (Rieger et al., 2010), although widespread sporadic, weak selective albitisation of plagioclase is taken to represent sub-ocean floor spilitisation (Benevides et al., 2007).
Benevides et al. (2007) interprets the following sequence of regional- to district-scale alteration: (1) Early, sporadic, widespread, but poorly exposed potassium-iron metasomatism which converted both granitoid and volcanic rocks to orthoclase (with subordinate biotite), accompanied by the deposition of magnetite (at 130 to 126 Ma; Chen, 2010), with associated fluorapatite, and minor pyrite, but no chalcopyrite. Homogenisation temperatures of 550 to 460°C are estimated for this phase (Benevides et al., 2007). (2) Subsequent regional scapolitisation in the area between the Atacama fault system and the marginal basin, characterised by marialitic scapolite. Later in this second stage, much of the scapolite was replaced by chlorite as part of an episode of chlorite and sericite (hydrolytic) alteration and veining (at 350 to 300°C), with minor development of hematite, pyrite and trace chalcopyrite (Benevides et al., 2007). This style of alteration grades from a slightly chloritised country rock, to chlorite-quartz veinlets, into chlorite-quartz-cemented hydrothermal breccias with mostly silicified, and occasionally K-altered, fragments, the Breccia Verde as detailed below (Rieger et al., 2010).
The Mantoverde IOCG sensu stricto deposits, were subsequently emplaced along the main and, more commonly, subsidiary segments of the major Atacama fault system, together with chalcopyrite-bearing, but sub-economic metasomatic magnetite, and copper-barren magnetite-fluorapatite-pyrite bodies. All are hosted by Middle to Upper Jurassic andesites of the La Negra Formation, and by diorite and monzodiorite assigned to the Lower Cretaceous (126 to 120 Ma) Sierra Dieciocho plutonic complex (Benevides et al., 2007). In the immediate Mantoverde district, mineralisation is developed within an intensely fractured structural block, delimited by the subvertical central and eastern branches of the northsouth Atacama fault system, connected obliquely by the northnorthwest trending, brittle, Mantoverde fault (MVF). The MVF is a releasing strike-slip duplex, representing a transfer zone between the central and eastern branches of the Atacama fault system. Most of the Mantoverde deposits are closely associated with a 12 km interval of the MVF and sub-parallel minor faults (Benevides et al., 2007; Rieger et al., 2010; Rieger et al., 2010; Rieger et al., 2009).
A loosely constrained Early Cretaceous age of mineralisation is based on K-Ar dating of two samples of hydrothermal sericite from Mantoverde Norte, with minimum ages of 117±3 Ma from an andesite and 121±3 Ma from a granite dyke (Vila et al., 1996). A two-point Re-Os isochron age derived from magnetite yielded 116 Ma, consistent with the alteration ages (Mathur et al., 2002).
Five main ore-bearing geological groupings or mineralisation zones/styles are recognised at Mantoverde:
1) Manto Atacama, a specularite-cemented, hydrothermal breccia, averaging 80 m in thickness (locally up to 200 m), in the hanging wall (east) of the 40 to 50° east-dipping MVF. It is composed of sub-angular to sub-rounded clasts, a few centimetres across, of mainly andesitic or granitoid igneous rocks in a mineralised, calcite-bearing, coarse-grained specularite matrix, altered to varying degrees by pervasive K feldspar, with more or less intense chlorite, sericite, silica and/or carbonate.
2) Transition zone, an adjacent specularite stockwork zone, immediately to the east and above the Manto Atacama, containing supergene copper oxide and hypogene sulphides, with essentially the same alteration as the Manto Atacama zone.
3) Mantoverde Breccia, a tectonic breccia in the footwall (west) of the main fault plane of the MVF, commonly 20 to 40 m thick. It comprises a rock flour matrix cataclastite, enclosing angular andesite and/or diorite clasts that are commonly a few millimetres to about 10 cm in diameter, with silica, chlorite and minor to moderate K feldspar alteration.
4) Breccia Verde, which is developed on both sides of the MVF, and has a gradational boundary with both the Transition zone and Mantoverde breccia. It is largely barren, and is composed of silicified and pervasively K feldspar altered volcanic and dioritic clasts within a matrix of chlorite-quartz, containing calcite and subordinate sericite, grading outwards into a chlorite-quartz stockwork. It is cut by sparse to moderately frequent sets of K feldspar±quartz, calcite±siderite, specularite,±quartz and sericite veinlets.
5) Magnetite Zone, developed between the Breccia Verde, an intrusion of the 120 to 127 Ma Sierra Dieciocho pluton to the west, and the Mantoverde Breccia to the east, and mainly at depth. It comprises magnetite-chlorite-sericite-K feldspar-cemented breccias, with clasts of igneous rock that are predominantly altered to magnetite (including mushketovite), K feldspar and quartz, cut by veinlets of K feldspar ±quartz, calcite, sericite and late specularite-calcite. Pyrite and chalcopyrite occur as small patches, disseminations, or in discontinuous veinlets. This zone is representative of the other magnetite-sulphide bodies in the south of the district (Rieger et al., 2010).
The bulk of the mining to 2010 exploited supergene mineralisation which occurs within the zone of oxidation that consistently persists to a depth of 150 to 250 m below the surface. Mineralisation comprises chrysocolla, brochantite, atacamite, almagre, malachite and copper-bearing hematite, jarosite, and goethite, disseminated in the breccia matrix, as filling of veinlets, and as a patina on, or patches within rock fragments (Rieger et al., 2010; Benevides et al., 2007). Below the base of supergene oxidation, in the Manto Atacama breccia, the specularite-rich matrix contains pyrite-chalcopyrite, with the chalcopyrite being locally replaced by digenite and bornite. The breccia is cut by veinlets of K feldspar±quartz, tourmaline, or sericite, with late stage calcite and specularite veinlets (Rieger et al., 2010). Neither native gold, nor electrum have been reported from the Mantoverde district, although there is a close correlation between gold and copper grades, and gold has been detected in both, chalcopyrite and pyrite (Rieger et al., 2010). In the northern half of the district, the mineralised tectonic breccias (e.g., Mantoverde Breccia), specularite cemented, hydrothermal breccias (e.g., Manto Atacama), and outer halo of mineralogically identical veins (Transition zone) are characterised by primary specularite and largely developed in the hanging wall of the MVF. In contrast, in the southern half of the district, there are hypogene, crudely tabular, massive bodies of magnetite-pyrite and magnetite-chalcopyrite (e.g., the Magnetite zone), predominantly in the footwall of the Mantoverde fault, while massive and irregular bodies of magnetite-apatite±pyrite are developed along the eastern branch of the Atacama fault system (Benevides et al., 2007).
The hypogene minerals of the Magnetite zone and the magnetite rich bodies in the south of the district comprise (1) magnetite stockworks and disseminations; (2) elongate magnetite-chlorite-sericite-K feldspar-cemented breccias, with igneous rock fragments that are mainly altered to an assemblage of magnetite, K feldspar and quartz; and (3) massive magnetite bodies. Both primary magnetite and mushketovite are present. These magnetite-rich rocks may be either barren, or contain chalcopyrite (and/ or pyrite), mainly as small patches, disseminations and/or discontinuous veinlets (Rieger et al., 2010). Rieger et al. (2010), notes a vertical zonation of mineralisation-associated iron oxides, with a downward transition from upper hematite to magnetite at depth. Tilting and differential rotation across the MVF has resulted in the magnetite mineralisation being exposed to the south, while drilling confirms the continuity of similar magnetite dominant mineralisation at depth immediately below the Mantoverde Breccia ores in the footwall of the MVF in the north. A lateral transition between specularite breccias and magnetite is not evident to the north, although the two are juxtaposed across the MVF, again due to the differential rotation accommodated by that structure. However, in the south, the Manto Atacama is only poorly developed and gives way to magnetite-rich rocks in the hanging wall, footwall and at depth.
Benevides et al. (2007) conclude that on the basis of textural relationships in both breccias and veins, the crystallisation of specularite and chalcopyrite was coincident. However, Rieger et al. (2010) interpret them to have been emplaced at different times, with the main mineralising events being (1) an early high-temperature iron oxide stage, comprising the bulk of the specularite and magnetite in the district; (2) a sulphide stage responsible for the main copper-gold mineralisation; and (3) a late stage, represented mainly by calcite±specularite and specularite veining, and pervasive carbonatisation. They suggest the iron oxide stage comprises early hematite (hm-I), followed by early magnetite (mt-I) which wholly or partially replaced hm-I (to form mushketovite), accompanied by pervasive K feldspar alteration, minor tourmaline and weak scapolite. Subsequent, variably intense silicification, pervasive sericitisation and minor pyrite (py-I) was followed by a second phase of magnetite (mt-II) and chloritisation, with specularite locally developing rather than mt-II. Rieger et al. (2010) see the sulphide phase as occurring in isolation, after the development of iron oxides (including the specularite breccia matrix), K feldspar, sericite, silica and chlorite. The iron oxide stage of Rieger et al. (2010) corresponds to both the potassium-iron metasomatism, scapolitisation and sericite-chlorite phases of Benevides et al. (2007), described above. Both agree on a terminal phase of alteration, which comprises largely barren calcite veining, variably accompanied by quartz.
Rieger et al. (2010) suggest the specularite associated with ore in the north of the district is their hm-I, on the periphery of the mineralised system, while the magnetite in the mineralisation at depth and to the south is both mt-I mushketovite after hm-I, and mt-II, which is deeper and hotter, proximal to the centre of mineralisation.
Rieger et al. (2010), noted a similar zonation in sulphur isotope signatures. Chalcopyrite in the Mantoverde district shows a wide range in sulphur isotope composition, with δ34SVCDT of between -6.6 and +10.0‰ (Rieger et al., 2010; Benavides et al., 2007). Systematic variation of these data reflect the spatial distribution of the sulphides in the orebodies and their position relative to the MVF. Sulphur isotopic compositions around 0‰ δ34SVCDT, which are compatible with a magmatic-derived sulphur component, are characteristic of chalcopyrite in orebodies with a close spatial relationship with the MVF in the southern and deeper, or proximal part of the Mantoverde district, representing the more internal parts of the hydrothermal system, while higher δ34SVCDT values of around +6‰, are typical of the northern part of the district, or the shallower, levels, suggesting sulphur contribution from non-magmatic sources in the peripheral portions of the hydrothermal system (Rieger et al. (2010) report the δ34S values for pyrite (0.2 to 9.4‰) to similarly indicate a dominantly magmatic source, although also showing systematic variations across the district, interpreted to reflect both the relative distance from inferred fluid conduits and the level of deposition within the hydrothermal system, and possible non-magmatic components on the peripheries of the system.
Homogenisation temperatures of between 550 and 460°C have been reported from hypersaline fluid inclusions (32 to 56 wt.% NaCl equiv.) in quartz coexisting with magnetite (Vila et al.,1996; Benavides et al., 2007 and unpublished sources quoted therein), while the main hypogene specularite breccia sulphide mineralisation is calculated to have formed at between 250 and 180°C (Benevides et al., 2007). Homogenisation temperatures of a number of determinations for late calcite-chalcopyrite and calcite veins from two and three phase fluid inclusions with salinities of from 32 to 40 wt.% NaCl equiv. and 1 to 10 wt.% NaCl equiv. returned values of 360 to 160°C and 260 and 112°C respectively (Vila et al., 1996 and unpublished sources quoted therein).
The majority of initial 87Sr/86Sr values of altered volcanic rocks and hydrothermal calcite from the district (0.7031 and 0.7060) are similar to those of the igneous rocks of the region. Lead isotope ratios of chalcopyrite are consistent with lead (and by inference copper) derived from Early Cretaceous magmatism. The sulphur, strontium and lead isotope data of chalcopyrite, calcite gangue and altered host rocks respectively, are compatible with the cooling of metal and sulphur-bearing magmatic hydrothermal fluids (with deposition at <350°C) that mix with meteoric waters or seawater at relatively shallow crustal levels. They suggest, input of additional exotic sulphur is likely, though not essential, for the deposition of copper mineralisation, occurring mainly in the shallow, and distal parts of the ore system.
Magnetites from the Mantoverde deposit have Os and Re concentrations of 11 to 17 ppt and 4 to 6 ppb, respectively. An initial 187Os/188Os ratio calculated from these is ~0.20, compared to ~0.36 to 0.33 at Candelaria, and from 0.20 to 0.41 for magmatic magnetite from Early Cretaceous batholithic intrusions in the district. These relatively radiogenic ratios are taken to represent a mixture of mantle and crustal components in the ores and batholitic rocks (Mathur et al., 2002).
Benevides et al. (2007) interpret isotopic and temperature data they quote from regional and shallow hematitic mineralisation to indicate their potassium-iron metasomatism stage was caused by magmatic fluids, possibly products of the second boiling of granitoid magmas, such as the nearby 126 to 120 Ma Sierra Dieciocho complex. However, they suggest the data indicates a change, probably during the scapolitisation and sericite-chlorite phases, possibly due to evaporite derived brines mobilised by marginal basin inversion reflected in the regional sodic alteration event, but even more likely during the deposition of chalcopyrite ore.
The copper resource at Mantoverde to the 800 m level was estimated prior to mining at 120 Mt @ 0.72% Cu (0.2% Cu cutoff) including a mineable reserve of 85 Mt @ 0.82% Cu.
In the mid to late 1990s, exploration identified:
- a Cu oxide resources of the order of 180 Mt @ 0.5% Cu overlying
- a hypogene sulphide resource of 440 Mt @ 0.56 % Cu, 0.12 g/t Au at a 0.20 % Cu cut-off.
Remaining mineral resources at 31 December, 2014 at a 0.2% Cu Total cutoff (Anglo American Annual Report, 2015) were:
Oxide ore (Heap Leach)
Measured + indicated resource - 67.4 Mt @ 0.35% Cu Acid Sol.,
Inferred resource - 2.6 Mt @ 0.29% Cu Acid Sol.,
Oxide ore (Dump Leach)
Measured + indicated resource - 25.3 Mt @ 0.16% Cu Acid Sol.,
Inferred resource - 2.3 Mt @ 0.16% Cu Acid Sol..
Remaining Ore Reserves and Mineral Resources at 31 December, 2022 (Capstone Copper Reserve and Resources online, viewed March 2024) were:
Sulphide + Mixed ore (Flotation)
Proved + Probable Reserve - 236.276 Mt @ 0.59% Cu, 0.10 g/t Au,
Measured + indicated Resource - 599.563 Mt @ 0.46% Cu, 0.11 g/t Au, (Inclusive of Ore Reserves)
Inferred Resource - 605.434 Mt @ 0.37% Cu, 0.08 g/t Au,
Oxide + Mixed ore (Dump + Heap Leach)
Proved + Probable Reserve - 221.115 Mt @ 0.22% Cu Acid Sol.,
Measured + Indicated Resource - 476.588 Mt @ 0.20% Cu Acid Sol., (Inclusive of Ore Reserves),
Inferred Resource - 70.721 Mt @ 0.15% Cu Acid Sol..
The mine is operated and controlled by Minera Mantos Blancos S.A., which was initially an Anglo American Group company, but was sold to be part of Mantos Copper S.A. in 2015. In 2021, Mantos Copper merged with Capstone Mining to become Capstone Copper which took control of Mantoverde.
The most recent source geological information used to prepare this decription was dated: 2006.
This description is a summary from published sources, the chief of which are listed below. © Copyright Porter GeoConsultancy Pty Ltd. Unauthorised copying, reproduction, storage or dissemination prohibited.
Mantoverde
|
Selected References:
|
Benavides J, Kyser T K, Clark A H, Oates C J, Zamora R, Tarnovschi R and Castillo B, 2007 - The Mantoverde Iron Oxide-Copper-Gold District, III Región, Chile: The Role of Regionally Derived, Non-magmatic Fluids in Chalcopyrite Mineralization: in Econ. Geol. v102 pp 415-440
|
Chen H, 2010 - Mesozoic IOCG Mineralisation in the Central Andes: an Updated Review: in Porter T M, (Ed), 2010 Hydrothermal Iron Oxide Copper-Gold and Related Deposits: A Global Perspective PGC Publishing, Adelaide v.3 pp. 259-272
|
Chen H, Cooke D R and Baker M J, 2013 - Mesozoic Iron Oxide Copper-Gold Mineralization in the Central Andes and the Gondwana Supercontinent Breakup: in Econ. Geol. v.108 pp. 37-44
|
Childress, T.M., Simon, A.C., Reich, M., Barra, F., Arce, M., Lundstrom, C.C. and Bindeman, I.N., 2020 - Formation of the Mantoverde iron oxide-copper-gold (IOCG) deposit, Chile: insights from Fe and O stable isotopes and comparisons with iron oxide-apatite (IOA) deposits: in Mineralium Deposita v.55, pp. 1489-1504.
|
Marschik, R, and Kendrick, M.A., 2015 - Noble gas and halogen constraints on fluid sources in iron oxide-copper-gold mineralization: Mantoverde and La Candelaria, Northern Chile: in Mineralium Deposita v.50 pp. 357-371
|
Rieger A A, Marschik R and Diaz M, 2012 - The evolution of the hydrothermal IOCG system in the Mantoverde district, northern Chile: new evidence from microthermometry and stable isotope geochemistry: in Mineralium Deposita v.47 pp. 359-369
|
Rieger A A, Marschik R and Díaz M, 2010 - The Mantoverde District, Northern Chile: an Example of Distal Portions of Zoned IOCG Systems: in Porter T M, (Ed), 2010 Hydrothermal Iron Oxide Copper-Gold and Related Deposits: A Global Perspective PGC Publishing, Adelaide v.3 pp. 273-284
|
Rieger AA, Marschik R, Diaz M, Holzl S, Chiaradia M, Akker B and Spangenberg JE, 2010 - The Hypogene Iron Oxide Copper-Gold Mineralization in the Mantoverde District, Northern Chile: in Econ. Geol. v105 pp 1271-1299
|
Verdugo-Ihl, M.R., Ciobanu, C.L., Courtney-Davies, L., Cook, N.J., Slattery, A., Ehrig, K., Tornos, F. and Hanchar, J.M., 2022 - U-Pb Geochronology and Mineralogy of Hematite from Mantoverde and Carmen de Cobre, Northern Chile: Constraints on Andean IOCG Mineralization: in Econ. Geol. v.117, pp. 943-960.
|
Vila T, Lindsay N, Zamora R 1996 - Geology of the Manto Verde copper deposit, Northern Chile: A specularite-rich, hydrothermal-tectonic breccia related to the Atacama Fault Zone: in Camus E, Sillitoe R H, Peterson R (Eds), 1996 Andean Copper Deposits: New Discoveries, Mineralisation, Styles and Metallogeny Soc. Econ. Geol. Spec Pub no. 5 pp 157-170
|
Williams P J, Barton M D, Johnson D A, Fontbote L, de Haller A, Mark G, Oliver N H S and Marschik R, 2005 - Iron oxide copper-gold deposits: Geology, space-time distribution and possible modes of origin: in Hedenquist J W, Thompson J F H, Goldfarb R J and Richards J P (Eds.), 2005 Economic Geology 100th Anniversary Volume, Society of Economic Geologists, Denver, pp 371-405
|
Zhu, Z., 2016 - Gold in iron oxide copper-gold deposits: in Ore Geology Reviews v.72, pp. 37-42.
|
Porter GeoConsultancy Pty Ltd (PorterGeo) provides access to this database at no charge. It is largely based on scientific papers and reports in the public domain, and was current when the sources consulted were published. While PorterGeo endeavour to ensure the information was accurate at the time of compilation and subsequent updating, PorterGeo, its employees and servants: i). do not warrant, or make any representation regarding the use, or results of the use of the information contained herein as to its correctness, accuracy, currency, or otherwise; and ii). expressly disclaim all liability or responsibility to any person using the information or conclusions contained herein.
|
Top | Search Again | PGC Home | Terms & Conditions
|
|