San Cristobal |
|
Bolivia |
Main commodities:
Ag Zn Pb
|
|
 |
|
 |
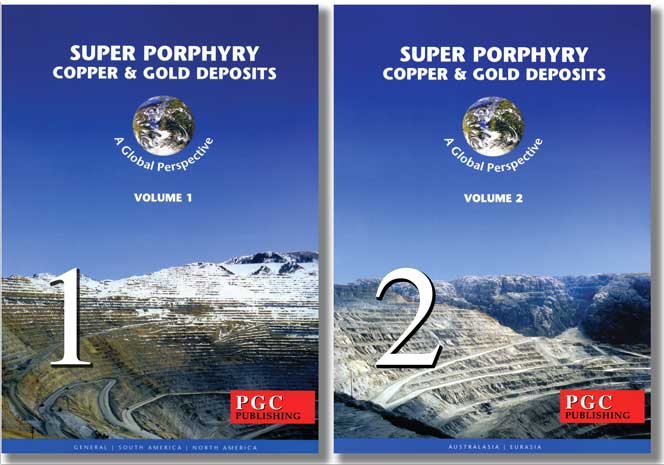 |
Super Porphyry Cu and Au

|
IOCG Deposits - 70 papers
|
All available as eBOOKS
Remaining HARD COPIES on
sale. No hard copy book more than AUD $44.00 (incl. GST) |
|
 |
The San Cristobal silver-zinc-lead deposit is located in the Altiplano of southern Bolivia, 235 km SW of Potosi, 115 km ENE and 210 km NNW of the borders with Chile and Argentina respectively (#Location: 21° 05' 19"S, 67° 12' 31"W).
Regional Setting
The Altiplano province of western Bolivia contains a series of high intermontane basins located between the Cordilleras Occidental and Oriental to the west and east respectively.
The Cordillera Oriental is a fold and thrust belt largely composed of deep marine, platform and delta facies rocks of Palaeozoic and Mesozoic age, mostly deposited on Proterozoic basement (Richter et al., 1992).
The Cordillera Occidental, includes the present volcanic crest of the Andes, with an eastern boundary with the Altiplano, that coincide with the eastern and northern limit of late Miocene-Holocene stratovolcanoes (Richter et al., 1992).
The Altiplano was formed between the Cordillera Occidental and Cordillera Oriental uplifts. Folding was initiated at ~25 Ma, which resulted in the dominant structural grain of the Altiplano (Lamb and Hoke, 1997).
Geology
The San Cristobal deposit occurs in a volcanic centre overlying subvertical beds of the Paleocene-Eocene Potoco Formation. This volcanic centre has produced an extensive pile of volcanic rocks that conformably overlie an unnamed unit of red silty sandstone derived from erosion of the Potoco Formation.
The volcanic pile includes a 4 km diameter cauldron that contains mineralised volcano-sedimentary sedimentary rocks. A series of barren dacitic domes are distributed around the periphery of the cauldron.
A linear NNE oriented trend of strongly altered and mineralised extrusive to shallowly intrusive rhyodacite domes bisect the cauldron.
The individual units of the district may be summarised as follows (from Phillipson and Romberger 2002):
• Potoco Formation - of Paleocene to Eocene age, composed very fine- to fine-grained quartz sandstones, composed of rounded to sub-rounded grains, that are thinly bedded, with cross-bedding, scour structures and low amplitude ripple marks. Beds dip at 60 to 75°NW to 60 to 75°SE, striking at 30 to 40°.
• Un-named unit - fine-grained reddish-brown sandstone and siltstone, which is up to 50 m thick proximal to the volcanic centre, but normally forms a 2 to 5 m thick resistent layer directly below poorly welded, feldspar-rich volcaniclastic rocks.
• Porphyritic vitric hornblende-biotite andesite sills - dated at 22.82±0.16 Ma to 21.68±0.26 Ma (Ar40/Ar39 on biotite), containing aligned biotite and hornblende phenocrysts defining a flow foliation, parallel to the bedding in the Potoco Formation that occur along trend, and define a 750 m wide zone that cuts the San Cristobal volcanic centre.
• Miocene Volcanic Pile - up to 200 m thick, extending over an area with a diameter of 15 km, unconformably overlying the Potoco Formation, but conformably above the red sandstone, composed of:
- Lower Crystal-Lithic Tuff, that is lightly welded, and contains sparse dacite fragments,
- Middle Crystal-Lithic Tuff, containing ~75% wdlded ash matrix, 20% dacite-lithic clasts, 5% red silty-sandstone clasts and 1% biotite crystals, and randomly oriented, unsorted and angular 10 to 250 mm diameter clasts of dacite that increase in size and frequency upwards.
- Volcanic Breccia, with 0.1 to 4 m diameter clasts of vitric dacite porphyry, dated at 8.102±0.07 to 7.88±0.12 Ma (Ar40/Ar39 on biotite).
• Dacite Flow Domes, which occur around the periphery of the cauldron, and are characterised by fine- to medium-grained, subhedral to euhedral plagioclase phenocrysts in a glassy matrix that makes up 30 to 60% of the rock. These dacites also contain 3 to 15% fine-grained euhedral to subhedral hornblende phenocrysts, 15% fine-grained biotite, 1 to 5% fine-grained, euhedral magnetite, <5% fine- to medium-grained, euhedral quartz and <3% fine- to medium-grained, euhedral to subhedral sanidine phenocrysts. Dacite on the western margin of the cauldron contains plagioclase that is significantly more calcitic than that to the north, which are, in turn, more calcic than domes that bisect the cauldron along the 30 to 40° trend. Whole rock analyses suggest that the dome in the western edge of the cauldron represents the least differentiated magma in the volcanic centre, containing 60.57 to 63.99 wt.% Si02. Other peripheral dacitic domes are characterised by 61.62 to 65.12 wt.% Si02, with the northern-edge dome representing the most differentiated peripheral dacite at 68.82 to 69.65 wt.% Si02.
Magmatic foliation, defined by aligned biotite and hornblende phenocrysts, are discordant to the regional structural orientation defined by the Potoco Formation. Flow foliation in individual domes have axes that strike at from 90 to 145°, in contrast to the consistant 030° striking Potoco Formation.
• Finely laminated mudstones and siltstones, at least 300 m thick, interbedded with tuffaceous clastic sedimentary rocks, fill the 4 km wide cauldron. Units dominated by angular, commonly moderately sorted and imbricated, 10 to 300 mm dacite and rhyodacite clasts, are found within this sequence, interpreted to be autobreccias, spalled from resurgent domes. Such lenses of breccia extend from flow domes toward the basin centre, and thin within a few tens of metres from domes. Some 100 mm diameter dacite clasts appear to represent bombs and produce sag textures in fine-grained tuffaceous units that contain small cross-beds.
• Rhyodacite domes, dated at 7.897±0.071 to 7.49±0.18 Ma (Ar40/Ar39 on biotite) bisect the cauldron along a 40° trend. They may be distinguished from the peripheral dacitic domes, as they contain 10 to 30 mm, concentrically zoned, sanidine phenocrysts, a greater abundance of quartz phenocrysts, sodic plagioclase, no magnetite and a higher silica content (69.65 to 70.46 wt.% Si02). Some 20% of the rock is composed of euhedral to anhedral, commonly resorbed and 'sericitized', or potassically altered, 0.5 to 2.5 mm plagioclase phenocrysts. These rocks also contain up to 5% fine-grained resorbed, anhedral sanidine phenocrysts; <5% fine-grained angular and anhedral, resorbed quartz phenocrysts; and up to 10% relict and euhedral biotite phenocrysts that are as much as 1.5 mm in diameter. Between 40 and 60% of the rock is represented by the partially devitrified groundmass, which commonly contains compositional and flow banding. Euhedral 1 to 3 mm biotite phenocrysts are frequently aligned in a magmatic flow foliation. The flow foliation and bands are parallel to the 40° strike of the underlying Potoco Formation.
Several regional lineaments intersect at the San Cristobal volcanic center. The most prominent is defined by the upturned edge of the subvertical Potoco Formation, which regionally strikes at 30° but is defracted to 40° beneath the cauldron. The Potoco Formation hosts hornblende-andesite sills for several kilometres along strike to the SW of San Cristobal. The flow foliations in hornblende-andesite sills and rhyodacitic domes are parallel to the regional structural grain defined by the Potoco Formation. Dacites that occupying the southwestern and western ring fractures, which are roughly aligned with the underlying Potoco Formation, contain faults and joints subparallel to Potoco Formation bedding.
A second major north-trending lineament is seen as a linear erosional trend through the volcanic pile, by localised hot spring deposits, and by dense parallel fracture swarms in domes.
A third lineament, 1 km south of the cauldron, trends at 65° and is reflected by 10 m wide fracture zones in dacite, sheared red silty sandstone, by several manganese fracture zones in rhyodacite and by a ridge of fractured and sheared dacite. TRhis trend parallels the dacite domes in the southeastern ring fracture.
The deposit is interpreted to have been emplaced at the intersection of regional structural weaknesses. The subvertical Potoco Formation redbeds, which controlled the intrusion of Early Miocene hornblende-andesite sills and Late Miocene resurgent rhyodacites, may have also allowed dilatent zones to form in response to regional oblique compression that occurred during Early and Late Miocene phases of the Andean Orogeny (Lamb and Hoke, 1997).
Mineralisation
The earliest mineralisation was veins of barite and coeval hematite, magnetite or pyrite, light coloured sphalerite and manganite. Galena and dark sphalerite were subsequently introduced into veins, accompanied by silver, occurring as argentiferous galena, jamesonite, pyrargyrite, polybasite and native silver.
The mineralisation hosted by tuffaceous sedimentary rocks of the cauldron differs from those of the veins. The lowest of these sequences contain stratabound sphalerite, galena, marcasite and pyrite, whereas argillically altered sedimentary rocks proximal to rhyodacites contain very fine-grained native silver, smithite, argentopyrite and argentite. The characteristics of these styles of mineralisation are as follows (from Phillipson and Romberger 2002):
• Ring fracture vein mineralisation - occurs as 30 to 500 mm thick barite veins cutting crystal-lithic tuffs, dacite and rhyodacite. Up to 85% of individual veins is occupied by tabular, euhedral to subhedral, 2 to 15 mm long barite crystals that form a skeletal framenwork. Approximately 15% of the veins are interstitial 0.5 to 2.5 mm void spaces. Cleavages and crystal boundaries are covered by very fine grained hematite and manganiferous hematite, with submicroscopic Pb and Zn minerals. Sparse 2 to 3 mm acicular jamesonite are oblique to barite growth, while 1 mm pyrite crystals are found within individual barite crystals. Interstitial crystals include 5 to 10 mm octahedral argentiferous galena, 1 to 3 mm cubic galena, 1 to 2 mm euhedral sphalerite and anhedral to subhedral quartz. Silver-bearing pyrite veins that included native silver and stromeyerite are also reported, with local chalcopyrite, arsenopyrite, pyrargyrite, tetrahedrite, galena, sphalerite and polybasite. Crustiform chalcedony occurs with 1 to 4 mm bands of hematite, galena, pyrite and sphalerite in fractured barite veins. Disseminated pyrite and anglesite coated galena are found in argillically altered rhyodacite.
Mineralisation is also found in hydrothermally altered hydrothermal- and auto-breccias near intrusive contacts between dacite and volcano-sedimentary rocks. Pre-sulphide silicification occurs as 1 mm thick chalcedony coatings on 'sandstone' breccia clasts, coated in turn by dark sphalerite, pyrite, cubic galena, chalcopyrite and euhedral quartz. Disseminated pyrite, sparse chalcopyrite and galena occur throughout the breccia matrix. In the interior of brecciated dacite domes, large (up to 500 mm), rotated, subrounded, dacite clasts occur within a very fine matrix that hosts sphalerite, chalcopyrite and pyrite.
• Tuffaceous sedimentary rock-hosted mineralisation - which occurs within the cauldron, and comprises around half of the San Cristobal orebody. Stratabound sphalerite, marcasite and pyrite are hosted by black shale, while wurtzite is also present in tuffaceous sedimentary rocks. Veinlets containing sphalerite, marcasite and pyrite truncate bedding planes in tuffaceous sedimentary rocks, suggesting mineralisation along bedding planes might also be replacive or vein related. Veins within cauldron-hosted sedimentary rocks are characterised by a framework of intergrown tabular barite crystals with interstitial arsenopyrite, chalcopyrite and pyrite that are commonly intergrown with light green sphalerite and euhedral quartz. Tabular barite crystals occur in vugs in argillically altered tuffaceous rocks that also host 1 to 2 mm disseminated pyrite, marcasite, galena, sphalerite, anglesite, sub-microscopic wurtzite, smithite and argentopyrite.
Sphalerite and galena increase to a depth of ~220 m, then decreases below 260 m where copper-dominated veins are found in microbrecciated dacite, characterised by an assemblage that includes pyrite, chalcopyrite, sphalerite, tennantite, famatinite and digenite.
• Rhyodacite associated mineralisation - which comprises near 50% of the San Cristobal orebody. Large rhyodacite domes contain finely disseminated pyrite, galena, sphalerite and native silver at the contact with tuffaceous sedimentary rocks within the cauldron. Sphalerite is frequently found in leached feldspar phenocrysts, while very fine-grained euhedral pyrite crystals are common along flow bands and form rings around sericitised plagioclase phenocrysts. Sulphides are common within the matrices of those breccias containing randomly oriented, subangular clasts of rhyodacite and siltstone in a matrix of alternating bands of galena, sphalerite, marcasite and brown sphalerite. These are interpreted to be the product of hydrothermal solution collapse, while matrix supported breccias composed of angular rhyodacite clasts in a glassy silica matrix are taken to be autobreccias. Matrix supported breccias with angular rhyodacite clasts in a matrix of tuffaceous pyrite, sphalerite and galena is interpreted to be a quench fragmental breccia.
Phillipson and Romberger (2002) suggest mineralisation may have begun with sulphide precipitation from hot springs in the cauldron lake. As the cauldron filled with sediments, and the hot springs were buried, hydrothermal fluids may have circulated through basin fill material to produce a vein and replacement style of mineralisation.
Alteration zones are centred around rhyodacite domes, with zones of silicification in the rhyodacite carapace breccias, which grade outward into avalanche breccia. These silicified breccia are surrounded by an extensive mixed argillic and advanced argillic shell that covers an area of several square kilometres. Alunite, kaolinite and illite are dominant proximal to domes and to depths of as much as 235 m below the surface in tuffaceous sedimentary rocks, while montmorillonite, illite, halloysite and dickite are more prominent distal to the dome. The advanced argillic alteration is underlain by a green propylitic suite (chiefly chlorite after biotite and hornblende, and epidote after plagioclase) within the basal tuffaceous sedimentary rocks of the cauldron and parts of the rhyodacites, grading outwards into unaltered rocks. No carbonate minerals are observed in the propylitic assemblage, although calcite may be found in sericitised plagioclase.
The cores of plagioclase phenocrysts in rhyodacite are selectively replaced by pink K feldspar and fine grained alunite and adularia. Adularia is also found as linings of vugs and as matrix replacement.
The mineralising system is predominantly a low sulphidation type, suggested by sphalerite as the dominant metallic mineral, widespread barite veins and adularia as an important alteration mineral (Hayba et al., 1985). The presence of alunite, with resurgent rhyodacite domes and late stage copper sulphosalts in veins suggest that the system evolved over time into a short-lived high sulphidation system by the end of the main mineralising phase (Phillipson and Romberger 2002).
Phillipson and Romberger (2002) indicate that fluid inclusion data indicate silver, lead and zinc were transported as chloride complexes and precipitated by cooling in veins, from <5 wt.% NaCl eq. fluids at 170 to 215°C. Silver spatially and perhaps temporally associated with resurgent rhyodacite was transported as a chloride comp1ex and precipitated by increased H2S activity or increased fluid pH.
Proved + probable reserves at the San Cristobal mine as at June 30, 2010 (Minera San Cristobal website, 2014) were:
258.823 Mt @ 1.41% Zn, 0.48% Pb and 53.0 g/t Ag.
This summary is taken from Phillipson, S.E. and Romberger, S., 2002, Emplacement and mineralization of the San Cristobal Ag-Zn-Pb deposit in southern Bolivia; SME Transactions, v. 310, pp. 80-88.
The most recent source geological information used to prepare this decription was dated: 2002.
This description is a summary from published sources, the chief of which are listed below. © Copyright Porter GeoConsultancy Pty Ltd. Unauthorised copying, reproduction, storage or dissemination prohibited.
|
Porter GeoConsultancy Pty Ltd (PorterGeo) provides access to this database at no charge. It is largely based on scientific papers and reports in the public domain, and was current when the sources consulted were published. While PorterGeo endeavour to ensure the information was accurate at the time of compilation and subsequent updating, PorterGeo, its employees and servants: i). do not warrant, or make any representation regarding the use, or results of the use of the information contained herein as to its correctness, accuracy, currency, or otherwise; and ii). expressly disclaim all liability or responsibility to any person using the information or conclusions contained herein.
|
Top | Search Again | PGC Home | Terms & Conditions
|
|