Pea Ridge |
|
Missouri, USA |
Main commodities:
Fe REE Au
|
|
 |
|
 |
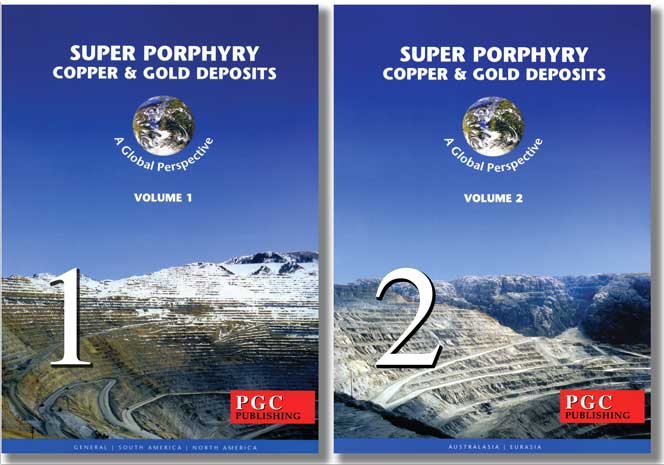 |
Super Porphyry Cu and Au

|
IOCG Deposits - 70 papers
|
All available as eBOOKS
Remaining HARD COPIES on
sale. No hard copy book more than AUD $44.00 (incl. GST) |
|
 |
Pea Ridge is a Proterozoic volcanic hosted, breccia related magnetite deposit, with associated REE and some gold, in the Saint Francois district of South-east Missouri, located ~90 km SW of Saint Louis and ~14 km SE of the town of Sullivan (#Location: 37° 7' 28"N, 91° 2' 45"W).
For details of the regional to local scale setting and geology of the Saint Francois Mountains district see the separate Southeast Missouri Iron District record.
The deposit was discovered following airborne and ground magnetic surveys in 1948 and follow-up drilling. Shaft sinking commenced in 1957 and production in 1964 by the Meramec Mining Company, a joint venture between the St Joseph Lead Company and Bethlehem Steel Company. From 1981 to 1987 the mine was operated by Fluor and was subsequently acquired by Woodridge Resources until its closure in 2001. The mine was closed and flooded in 2001.
The Pea Ridge deposit is hosted by Mesoproterozoic felsic volcanic rocks of the St. Francois terrane, comprising ash-flow tuffs and lavas, interpreted to have been formed in the root of a volcanic caldera (Nuelle et al., 1991). The deposit has been dated at 1.465 Ga by a U-Pb determination on xenotime from a mineralisation related quartz vein (Van Schmus, 1988). Elsewhere the host volcanic rocks have been dated in the range 1.5 to 1.4 Ga (Bickford and Morse, 1975). Kisvarsanyi (1981) inferred the presence of an underlying central granite pluton, with the deposit hosted within volcanic rocks in its roof zone. This interpretation was based on aeromagnetic data, although drilling had not intersected the pluton by 1989 (Marikos et al., 1989). However, the high Y, Th, U, F and Sn content of late mineralisation and the presence of alaskite dykes are consistent with proximity to such a pluton, which is bounded by magnetite-rich trachyte intrusions that form an anomalous magnetic signal on its southern and western margins, and outcrop to the south (Kisvarsanyi,1981;Marikos et al., 1989). Marikos et al. (1989) further argue that the steep dip of the host volcanic rocks suggest they are part of a foundered roof block or pendant above the pluton. The steep dip of the deposit suggests it was emplaced after the tilting of the host rocks. Seeger (2000) suggests zones of weakness in the rhyolite host rocks, caused by ring complex development or caldera collapse and resurgence, controlled ore emplacement. The geometry of the deposit also suggest it was not controlled by the bedding and flow lineation of the volcanic hosts, but by fracturing (Marikos et al., 1989).
The magnetite orebody is tabular, striking at 60° over a length of ~850 m, and dipping at 70°W, from the base of the unconformity with the overlying Palaeozoic sedimentary rocks at ~400 m to a depth of more than 975 m. There is a prominent bulge on its eastern end where it ~180 m thick, tapering to less than 50 m in the upper levels to the west, although with depth this section thickens to >120 m in width, while on the eastern end the body thins with depth. The eastern, thicker end was eroded at the unconformity, forming a basement high, while the western extremity is 50 m below the palaeo-surface. This geometry suggests the lensoid-tabular body plunges to the SW (Marikos et al., 1989).
Hematite forms a cap to the upper section of the deposit in the basement high on the eastern quarter of the orebody, where has overprinted the magnetite to a depth of ~100 m below the unconformity and locally persists as a downward tapering zone for another 50 to 100 m flanking the massive magnetite to the north, east and south (Marikos et al., 1989).
The massive magnetite ore averages 53 to 55% Fe, but may be as rich as 65% Fe (90 wt.% magnetite). It generally occurs as fine-grained magnetite, but may locally form octahedra >1 cm long in cavities and fractures. From the core of the deposit outwards the mineralisation grades from massive magnetite enclosing occasional angular to rounded wall rock clasts, through breccias composed of rounded to angular boulders with a magnetite matrix, to crackle breccia and pseudobreccia in the wall rocks. The narrow, tapering lateral eastern and western extremities of the magnetite body are composed of angular- to crackle breccias. The selvage between fresh rock and magnetite in the breccias varies from 0 to 1.5 cm thick and is typically characterised by bleaching and reddening of the country rock, reflecting depletion of magnetite and mafic minerals adjacent to fractures. At least two generations of apatite are found throughout the magnetite, occurring as veins and broken crystals. Both generations are cloudy grey-brown with abundant inclusions, and some yellow and transparent varieties (Marikos et al., 1989). Apatite in massive magnetite is associated with monazite gangue (Sidder et al., 1993). Pyrite is present as disseminated grains, lacey veinlets and stockworks that form the breccia matrix, as well as distinct veinlets within and post-dating the main magnetite mineralisation (Marikos et al., 1989).
The massive magnetite body is discontinuously fringed by zones of amphibole, specifically ferro-actinolite, in direct contact on both the hanging wall and footwall margins. The amphibole rock includes rounded boulders, which grade out from their generally siliceous cores into crackle breccia, commonly rimmed by inwardly pointing amphibole crystals which were open-space grown (Marikos et al., 1989). The amphibole crystals are uralite pseudomorphs after pre-existing clinopyroxene (Kisvarsanyi, 1981). Garnet is also present within the amphibole rock (Kisvarsanyi, 1981; Marikos et al., 1989).
The amphibole zone passes outwards into country rocks across a gradational contact from massive amphibole replaced, to altered volcanic rocks with glomerocrysts of amphibole and chlorite rimmed biotite crystals
Aphanitic grey-green tholeiitic basalt dykes from a few cm to 4 m thick cut both the magnetite orebody and country rocks along steeply dipping east-west fracture zones and persist for hundreds of metres. Fragments of these dykes are found within the breccia pipes.
Silicification is notable both within and adjacent to the deposit, particularly on the northern margin, covering a volume larger than the iron oxide ore body (Seeger et al., 1989). Silicified rock is characterised by massive, white to light grey quartz that replaced rhyolite to varying intensities, with areas of greater than 75% quartz being common. Accessory minerals include potassium feldspar, fluorite, muscovite, biotite, tourmaline, epidote, calcite, barite, rutile, pyrite, chalcopyrite, and chlorite. Sericite is common on fracture and fragment surfaces. Silicification
Potassium metasomatism, ranges from sporadic to pervasive, is associated with silicification, occurring as the addition of potassium feldspar to silicified rock and surrounding wall rock (Seeger et al., 1989). It also forms potassium feldspar pegmatite pods, again associated with silicification. Epidote is a minor alteration product at Pea Ridge, where it is associated with silicification.
Four mapped REE-bearing breccia pipes steeply crosscut the magnetite-hematite orebody and its altered rhyolite host rock. They occur at or near contacts between major lithologic zones, dip steeply (>60°) and are elongate to ovoid in plan view. Portions of these breccia pipes are as much as 60 m in horizontal length and up to 15 m in width, and persist to an undetermined depth below the mined levels (Seeger et al., 2001). The pipes comprise variably cemented matrix supported angular, granule to boulder sized breccia clasts that include volcanic rocks, hematite, magnetite, and silicified and altered amphibole rock, mostly reflecting the local wall-rock (Seeger, 2000). The clasts are supported by a matrix of rock-flour (milled rhyolite wall rock and specularite grains) with feldspar, chlorite, barite, apatite, quartz and calcite. Where uncemented, porosity approaches 50%. Layering within the breccias parallel the pipe margin. Late stage silicification, with associated sericite, commenced during the formation of these breccias. Uncemented breccias contain earlier silicified clasts with a relict breccia texture,
These breccia pipes carry rare earth elements-bearing (REE) minerals that include monazite, xenotime, and rare bastnaesite and britholite. Monazite and xenotime occur as 0.5 to 1.0 mm granular crystals, radial crystal aggregates, acicular crystals replacing wall rock microfragments, and irregular crystals filling fractures in barite and feldspar (Nuelle et al., 1991). The REE concentrations in the breccia pipes are consistently high but variable, averaging 20 wt.% total REE oxide (Nuelle et al., 1992), with grab samples ranging from ~2.5 to 37.8 wt.% total REE oxide (Seeger et al., 2001). Bulk sampling by the U.S. Bureau of Mines yielded 7 to 25 wt.% total REE oxide, average of 12 wt.% (Vierrether and Cornell, 1993). Thorium and uranium are also present. Cerium, lanthanum and yttrium are found in recoverable quantities. Gold is erratically distributed and has been reported as electrum and sylvanite, with concentrations seldom exceeding 1 ppm, although individual assays are as high as 371 ppm (Husman, 1989).
Dark brown to black ferrodravite tourmaline occurs as thin films, up to 1 cm thick veins cutting silicified breccia, as well as forming clots in the breccia groundmass, but is only found in late stage brecciated or silicified rock.
Orange alaskite ("aplite") dykes. from ~1 cm to a metre or more in thickness are present in various attitudes, with associated potassic flooding, chloritisation, silicification, pyrite and hematite accompany the dykes which are interpreted to be coeval with the rhyolitic host rocks and and trachytic ring dykes (Day et al., 1989).
Randomly oriented quartz veins are found throughout the deposit, cutting all other lithologies, including silicified rocks. They range from thin veinlets to more than 1.25 m thick and locally contain rutile, xenotime, monazite, apatite, biotite, K feldspar, pyrite an rare molybdenite. Late cement and minerals encrusting clasts and fractures within the breccia include barite, fluorite, chalcopyrite, calcite, chamosite, hematite, REE-phosphate, fluorocarbons, anatase, altaite and galena.
Production from 1964 to 2001 totalled 53.1 Mt of magnetite pellets. Remaining proven reserves have been estimated at 120 million tonnes of 57% Fe with associated rare earth elements (REE) and traces of gold, and potential for up to 500 Mt of a similar or higher grade (Kisvarsanyi and Kisvarsanyi, 1989).
Historic, non NI 43-101 resources quoted on the Pea Ridge Resources website in 2013 were:
Measured resource - 85.4 Mt @ 58.0% Fe Total, including 50.8% Fe Magnetic;
Indicated resource - 85.4 Mt @ 58.9% Fe Total, including 51.9% Fe Magnetic;
Inferred resource - 49.8 Mt @ 55.9%% Fe Total, including 40.6% Fe Magnetic;
Estimated reserves of REE ores in four REE-bearing breccia pipes (Marikos et al., 1989 and sources quoted therein), were:
0.2 Mt @ 12 wt.% total REE oxide and traces of gold
The most recent source geological information used to prepare this decription was dated: 2001.
Record last updated: 19/7/2013
This description is a summary from published sources, the chief of which are listed below. © Copyright Porter GeoConsultancy Pty Ltd. Unauthorised copying, reproduction, storage or dissemination prohibited.
|
Selected References:
|
Aleinikoff, J.N., Selby, D., Slack, J.F., Day, W.C., Pillers, R.M., Cosca, M.A., Seeger, C.M., Fanning, C.M. and Samson, I.M., 2016 - U-Pb, Re-Os, and Ar/Ar Geochronology of Rare Earth Element (REE)-Rich Breccia Pipes and Associated Host Rocks from the Mesoproterozoic Pea Ridge Fe-REE-Au Deposit, St. Francois Mountains, Missouri: in Econ. Geol. v.111, pp. 1883-1914.
|
Ayuso, R.A., Slack, J.F., Day, W.C. and McCafferty, A.E., 2016 - Geochemistry, Nd-Pb Isotopes, and Pb-Pb Ages of the Mesoproterozoic Pea Ridge Iron Oxide-Apatite–Rare Earth Element Deposit, Southeast Missouri: in Econ. Geol. v.111, pp. 1935-1962.
|
Childress, T.M., Simon, A.C., Day, W.C., Lundstrom, C.C. and Bindeman, I.N., 2016 - Iron Oxygen Isotope Signatures of the Pea Ridge and Pilot Knob Magnetite-Apatite Deposits, Southeast Missouri, USA: in Econ. Geol. v.111, pp. 2033-2044.
|
Harlov, D.E., Meighan, C.J., Kerr, I.D. and Samson, I.M., 2016 - Mineralogy, Chemistry, and Fluid-Aided Evolution of the Pea Ridge Fe Oxide-(Y + REE) Deposit, Southeast Missouri, USA: in Econ. Geol. v.111, pp. 1963-1984.
|
Hofstra, A.H., Meighan, C.J., Song X.-Y., Samson, I., Marsh, E.E., Lowers, H.A., Emsbo, P. and Hunt, A.G., 2016 - Mineral Thermometry and Fluid Inclusion Studies of the Pea Ridge Iron Oxide-Apatite–Rare Earth Element Deposit, Mesoproterozoic St. Francois Mountains Terrane, Southeast Missouri, USA: in Econ. Geol. v.111, pp. 1985-2016
|
Johnson, C.A., Day, W.C. and Rye, R.O., 2016 - Oxygen, Hydrogen, Sulfur, and Carbon Isotopes in the Pea Ridge Magnetite-Apatite Deposit, Southeast Missouri, and Sulfur Isotope Comparisons to Other Iron Deposits in the Region: in Econ. Geol. v.111, pp. 2017-2032.
|
Mercer, C.N., Watts, K.E. and Gross, J., 2020 - Apatite trace element geochemistry and cathodoluminescent textures - A comparison between regional magmatism and the Pea Ridge IOAREE and Boss IOCG deposits, southeastern Missouri iron metallogenic province, USA: in Ore Geology Reviews v.116, 22p. doi.org/10.1016/j.oregeorev.2019.103129
|
Neymark, L.A., Holm-Denoma, C.S., Pietruszka, A.J.,Aleinikoff, J.N., Fanning, C.M., Pillers, R.M. and Moscati, R.J., 2016 - High Spatial Resolution U-Pb Geochronology and Pb Isotope Geochemistry of Magnetite-Apatite Ore from the Pea Ridge Iron Oxide-Apatite Deposit, St. Francois Mountains, Southeast Missouri, USA: in Econ. Geol. v.111, pp. 1915-1933.
|
Nold, J.L., Dudley, M.A. and Davidson, P., 2014 - The Southeast Missouri (USA) Proterozoic iron metallogenic province - Types of deposits and genetic relationships to magnetite-apatite and iron oxide-copper-gold deposits: in Ore Geology Reviews v.57, pp. 154-171.
|
Nuelle L M, Day C M, Sidder G B 1991 - Rare earth element- and gold-bearing breccia pipes of the Pea Ridge iron ore mine, Washington County Missouri: in Soc of Mining, Metallurgy & Exploration, Denver Colorado Preprint pp 91-109
|
References in PGC Publishing Books: | |
|
Seeger C M, 2000 - Southeast Missouri Iron Metallogenic Province: Characteristics and General Chemistry, in Porter T M, (Ed.), Hydrothermal Iron Oxide Copper-Gold & Related Deposits: A Global Perspective, v1 pp 237-248
Abstract
|
Porter GeoConsultancy Pty Ltd (PorterGeo) provides access to this database at no charge. It is largely based on scientific papers and reports in the public domain, and was current when the sources consulted were published. While PorterGeo endeavour to ensure the information was accurate at the time of compilation and subsequent updating, PorterGeo, its employees and servants: i). do not warrant, or make any representation regarding the use, or results of the use of the information contained herein as to its correctness, accuracy, currency, or otherwise; and ii). expressly disclaim all liability or responsibility to any person using the information or conclusions contained herein.
|
Top | Search Again | PGC Home | Terms & Conditions
|
|