Wodgina, Mount Cassiterite |
|
Western Australia, WA, Australia |
Main commodities:
Ta Li Sn
|
|
 |
|
 |
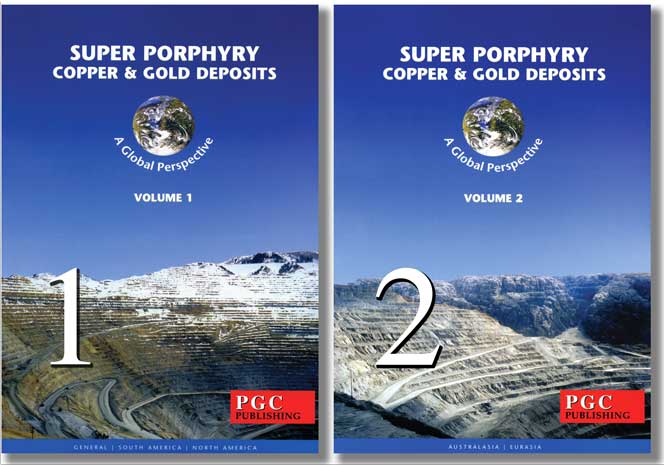 |
Super Porphyry Cu and Au

|
IOCG Deposits - 70 papers
|
All papers now Open Access.
Available as Full Text for direct download or on request. |
|
 |
The Wodgina and Mount Cassiterite tantalum-lithium-tin pegmatite deposits are hosted within the Palaeoarchaean East Strelley Greenstone Belt in the central Pilbara craton of Western Australia, located ~30 km SW of the Pilgangoora deposits and ~100 km SSE of Port Hedland in Western Australia (#Location: Wodgina - 21° 10' 42"S, 118° 40' 33"E; Mount Cassiterite - 21° 11' 25"S, 118° 40' 25"E).
The Wodgina mine was the world's major supplier of tantalite during much of the twentieth century. Tantalite had been discovered at Wodgina in 1901 by brothers Francis and William Michell, who subsequently extracted 70 tonnes of the ore between 1905 and 1909. Tantalite Ltd was subsequently incorporated, which undertook mining between 1925 and 1943, exporting tantalite ore concentrate mainly to the United States. In 1943, the mine was nationalised by the Australian Government as part of its wartime effort. Tantalite concentrate continued to be exported to the United States, and in addition during this period beryl was also exported. In 1927, geologist E.S Simpson had identified large masses of cesium bearing white beryl at the northern end of the pegmatite. It is thought this was used as part of the Manhattan Project, which was researching the development of an atomic bomb. Following World War II, Tantalite Ltd, continued to operate the mine, until 1953 when the mine was acquired by Northwest Tantalum Ltd. The deposit was subsequently deemed uneconomic, and the lease was relinquished in 1957. Between 1957 to 1963 the mine was operated on a small scale by prospectors, until purchased by Avela in 1967, and then by Goldrim Mining in 1968. Goldrim formed a partnership with Goldfield Corp. (New York) and Chemalloy Minerals Ltd. (Toronto). The investigations into the pegmatite by this last firm discovered the new species Wodginite. Mining occurred sporadically until Goldrim formed a new partnership with Pan West Tantalum Pty Ltd., who commenced open pit mining at the site in 1989 and progressively expanded during the 1990s. However, by 1994 most of the resources of the Main Tantalite Dyke, the principal deposit at the mine, had been exhausted and mining ceased. From 2001 to 2005, the deposit fell witjin title held by Sons of Gwalia, and then from 2005 to 2009 by Talison Minerals. Following the cessation of operatons at the Main Tantalite Dyke, mining was switched to the Mount Cassiterite deposit, ~2 km to the SSW. A major expansion in 2002 increased the mine's capacity to 635 t of tantalum pentoxide (Ta2O5) per annum. Active mining at the Mt. Cassiterite pit has been started and regularly stopped since 2008. Following a restructure in 2009, the tantalum interests were passed to a new division, Talison Tantalum, which then became Global Advanced Metals. The mine was placed on care and maintenance in 2008, 2012, and most recently in 2019 until it restarted in May 2022. In 2016, Mineral Resources Limited had acquired the mine and upgraded the processing facilities and site infrastructure to 750 ktpa spodumene plant producing 6% spodumene concentrate, completed in 2019. On October 31, 2019, Albemarle Corp. acquired a 60% interest in this hard rock lithium mine project and formed an unincorporated joint venture with Mineral Resources known as MARBL for the exploration, development, mining, processing and production of lithium and other minerals (other than iron ore and tantalum) from Wodgina. The tantalum resources remained with Global Advanced Metals. Production of spodumene concentrate from the first and second trains at the Wodgina mine was achieved in May and July of 2022, respectively, after it had been idled following its acquisition in 2019. On October 18, 2023, a restructuring of the MARBL JV, which resulted in the reduction of Albemarle's ownership interest in Wodgina to 50%.
The mining operation has extracted tantalum bearing pegmatite ores from the Mount Cassiterite and Tinstone open pits. The ores are crushed, milled and fed into the Wodgina plant's advanced gravity separation plant. A primary tantalum concentrate is produced at Wodgina, and then sent to the Greenbushes mining operation for secondary processing to produce on-specification, saleable tantalum products.
The North Pilbara Craton consists of large, domal, multiphase granitoid-gneiss complexes bordered by sinuous synformal to monoclinal greenstone belts (Hickman, 1983; Griffin, 1990; Barley, 1997). The greenstone belts range in age from ~3.56 to ~2.94 Ga, with the granitoids emplaced over a similar but slightly younger time span (e.g., Champion and Smithies, 1998). Although the supracrustal rocks are structurally complex, the primary stratigraphic units may be correlated between greenstone belts (Hickman, 1983, 1990). The granitoid-greenstone terrane of the North Pilbara Craton has been subdivided into tectonostratigraphic domains with boundaries defined by NNE-SSW to NE-SW trending structural lineaments that regionally have a sinistral shear sense. The lithotectonic units identified are the i). East Pilbara granite-greenstone terrane, ii). Central Pilbara tectonic zone, and iii). West Pilbara granite-greenstone terrane. At least seven episodes of granitic magmatism have been identified between 3.47 and 2.80 Ga. During this period, granitic magmatism became more potassic and large ion lithophile element enriched, with increased compositional variability from tonalite-trondhjemite granodiorite to calc-alkaline and alkaline granite compositions due to cyclic crustal reworking and growth (Champion and Smithies, 1998). Most of the granitoid-gneiss complexes have tectonic margins, with little evidence of contact metamorphism of adjacent supracrustal sequences (Hickman, 1983, 1990). Granitic magmatism culminated with emplacement of a suite of 2.89 to 2.83 Ga granite plutons at (e.g., Blockley, 1980; Pidgeon, 1984; Bickle et al., 1989; Smithies and Champion, 1998, 2001) and a 2.76 Ga suite of small A-type granites and stocks of tourmaline rich S-type peraluminous granites (Smithies and Champion, 1998). There is good spatial, geochemical, and geochronological evidence to link rare metal pegmatites in the North Pilbara Craton with emplacement of the younger granite suite (e.g., Blockley, 1980; Kennedy, 1998; Kinny, 2000; Sweetapple et al., 2000, 2001).
Exposed pegmatites in the Pilbara province are generally <5 km from outcropping plutons of the younger granite suite, and almost all pegmatite localities can be tied spatially, paragenetically and chronologically to those younger granites, which were emplaced between 2.89 and 2.83 Ga. These younger granite suites are composed of highly fractionated 'I' and 'A' type granites and monzogranites with elevated lithophile element contents, representing the culmination of magmatic evolution due to protracted continental growth and recycling of continental crust.
The pegmatites are predominantly developed within mafic-ultramafic rocks of relatively low metamorphic grade, mostly greenschist facies, whilst the most highly fractionated rare element-class pegmatites are hosted by tongues or inliers of greenstone belts protruding into or within the larger granitoid complexes. In addition, most of the rare metal pegmatites of the Pilbara pegmatite province are within the East Pilbara granite-greenstone terrane, aligned along, or close to, craton-scale lineaments that may coincide with, or are parallel to, tectonostratigraphic domain boundaries.
The Pilbara pegmatite province hosts >120 pegmatite deposits in over 25 pegmatite clusters, including the unusual albite-type pegmatite, the Wodgina main lode, and the giant albite-spodumene-type pegmatites of the Mount Cassiterite group, both with high-grade tantalum mineralisation.
Wodgina and Mount Cassiterite (<1 km to the south) are two of a series of major tantalum deposits, including Pilgangoora, Tabba Tabba and Strelley that are distributed along a NNE trending corridor, the Wodgina-Strelley lineament, within the same tectonostratigraphic domain.
The group of pegmatites that define the Wodgina district are hosted within the Wodgina greenstone belt, near the southern end of the regional Wodgina-Strelley pegmatite belt. The greenstone belt is exposed as a NNE plunging synformal structure folding a greenstone sequence that separates granitoid complexes. This structure forms a SW tapering wedge-shape that is ~25 km long and 10 km across at its widest point in the NE (Sweetapple et al., 2001). The greenstone sequence is mostly composed of mafic-ultramafic volcanic rocks, including komatiitic and clastic sedimentary rocks, banded iron-formation and chert, all of which have been subjected to greenschist to lower amphibolite facies metamorphism at relatively low pressures (Sweetapple et al., 2001; Blewett et al., 2001).
The greenstone belt wedge is bounded to the SE by a medium grained granite to monzogranite of the 2.89 to 2.83 Ga Numbana Monzogranite, a member of the younger granite suite, with a sliver of marginal leucogranite sporadically developed along the contact with the greenstone sequence. A large mass of older migmatite and gneissic granodiorite is found to the west, intruded by porphyritic granite to monzogranite, also of the Numbana Monzogranite (Sweetapple and Collins, 2002).
The principal tantalum bearing pegmatites (i.e., Wodgina and Mount Cassiterite) occur on the eastern limb of the synform, close to both the axis of the synform and a major NNE trending regional sinistral shear zone. They are separated by an east-west dextral shear zone, and are adjacent to the narrow marginal leucocratic phase of the Numbana Monzogranite to the SE. The Wodgina pegmatite district exhibits a regional zonation of pegmatite groups away from the parental Numbana granite of the Yule granitoid complex. This zonation progresses from a beryl-columbite sub-type (Beryl pegmatite group), then to an albite-spodumene type (Mount Cassiterite pegmatite group), and finally to the albite type (Wodgina pegmatite group). Each of these pegmatite types are almost invariably hosted by a different rock type, and reflect different structural controls (Sweetapple et al., 2001). Sweetapple and Collins (2002) suggest changes in pegmatite type are a function of host rock rheology, previous deformation and possibly thermal histories.
Both the Wodgina and Mount Cassiterite pegmatite groups have been emplaced syntectonically into fault/shear zones, with a predominantly reverse sense of movement. This emplacement has been related to a semi-concordant control of pegmatite distribution in both areas by F2 fold hinges and limbs (Sweetapple and Collins, 1998). The Wodgina main lode pegmatite appears to related to a major inclined fold hinge, while the pegmatites of the Mt. Cassiterite group appear to be sheets joined by a number of parasitic fold hinges (Sweetapple, 2000).
Other pegmatites in the Wodgina district include Stannum, West Wodgina and Mills Find.
Wodgina pegmatite group
This group includes the Wodgina main lode pegmatite, plus several smaller pegmatites that are mostly subparallel to the main structure. They are characterised by an abundance of cleavlandite and/or sugary albite, and are almost entirely hosted by variably foliated metakomatiite.
The Wodgina main lode pegmatite is a sheeted dyke that trends north-south, dips at 20 to 50°E, and cuts across the foliation of the host rock, which strikes north-south to NE-SW, and dips to the west. This pegmatite has a total strike length of ~1 km, and varies from 5 to 40 m in thickness. However, most mining has been confined to its northern 500 m, where the pegmatite segments are thickest. Small offshoots of the pegmatite are subparallel to the foliation of the host rocks.
The pegmatite postdates most of the deformation of the Wodgina greenstone belt and was apparently partially controlled by pre-existing folding and faulting. It is cut by late steeply dipping normal faults, whilst the northern end was disaggregated by later dip-slip faulting and late strike-slip shearing (Sweetapple et al., 2001). The northern portion of the pegmatite body has also been observed to be intruded around the hinge zone of an asymmetric fold, and its associated long limb (Sweetapple, 2000). While the pegmatite has a general easterly dip, it varies from a dyke in the south, to a large bulbous mass with a saddle shape that pinches out rapidly into thin angular sheets in the north.
Although pegmatite zonation typical of highly fractionated pegmatites is not well developed in the Wodgina main lode, the zonation that is developed, takes the form of two main compositional assemblages in primary layers that subparallel contacts, as: i). layers of massive cleavlandite (a variety of albite) with rare quartz, spessartine and muscovite on both the footwall and hanging wall of the dyke, and ii). a broad central unit of banded aplitic to granitic-textured medium to coarse grained albite-quartz-muscovite ±megacrystic perthitic microcline.
These assemblages are less regularly distributed on the northern end of the dyke. The central bulbous section of the main dyke contained a large irregular segregation of massive quartz up to 50 m wide and 60 m long. Ellis (1950) noted that the cleavlandite units appear to locally intrude the aplitic/granitic textured unit.
The marginal cleavlandite assemblage zones in the hanging wall and footwall are partially overprinted by a secondary fine-grained sugar-textured albite ±muscovite assemblage, and by lepidolite ±albite alteration of the micaceous aplitic-granitic textured unit. An intense 5 to 30 cm thick concentration of exomorphic mica was developed on the contacts with the wall rocks (Sweetapple, 2000). Hall (1988) notes discrete units rich in beryl, spodumene and fluorite associated with these secondary overprints.
In the main lode, the principal tantalum mineral is manganotantalite [Mn Ta2O6] with minor manganocolumbite [(Mn,Fe)(Nb,Ta)2O6] and wodginite [Mn2+(Sn,Ta)Ta2O8], with microlite [(Ca,Na)2Ta2O6(O,OH,F)] and fersmite [(Ca,Ce,Na)(Nb,Ta,Ti)2(O,OH,F)6] alteration (Miles et al., 1945; Sweetapple and Collins, 1998; Sweetapple et al., 2001).
This tantalum mineralisation is almost always within the massive cleavlandite units, commonly occurring in the core of radial aggregates of cleavlandite, and frequently as coarse skeletal-textured manganotantalite crystals clustered at the base of the hanging wall massive cleavlandite unit, suggesting precipitation and gravitational settling from that massive unit (Sweetapple, 2000).
These textural locations suggest some of the primary tantalum minerals were among the first minerals to crystallise, and co-crystallised with cleavlandite during undercooling and quenching within these units. The secondary albite and lepidolite replacement units also contain dispersed tantalum mineralisation, most likely indicating remobilisation of some of the tantalum. Masses of cesian beryl have been described in the north end of the pegmatite, in addition to the tantalum minerals (Ellis, 1950). Thorogummite, thorite, and massive lithiophylite and its alteration products have also been recorded (Simpson, 1928, 1951, 1952; Finucane and Telford, 1939).
The Wodgina main lode pegmatite is characterised by high grade tantalum mineralisation, and the presence of secondary lepidolite, or lithian muscovite, consistent with it being an extremely fractionated pegmatite (Sweetapple et al., 2001). The primary cleavlandite and secondary albite units constitute ~78 vol.% of the pegmatite. The bulk composition of the pegmatite is more sodic and less silicic than most other rare metal pegmatites, consistent with the albite type of Cerny (1993). Other albite-enriched pegmatites close to the Wodgina main lode pegmatite are also considered to be of the albite type, as they have similar internal structure and modal mineralogy. Sweetapple and Collins (2002) suggest the low contents of rare alkali elements and volatile elements such as B, P, F, and higher Mg and Fe values than other rare metal pegmatites are most likely to be the result of extensive ion exchange with the metakomatiite wall rock. The same authors suggest it provide field evidence for the separation of a residual sodic melt enriched in lithophile elements escaping from crystallising semi-consolidated albite-spodumene pegmatite sheets at Mount Cassiterite, and appear to represent a regional zonation relationship.
Mount Cassiterite pegmatite group
These pegmatites lies directly to the south of the Wodgina group and cover an area of ~1.1 x 0.8 km (Sweetapple and Collins, 1998). They comprise a series of interlinked pegmatite sheets, dykes and irregular offshoot structures, and in contrast to the Wodgina group, have been emplaced within a thick formation of metasedimentary rocks, mostly composed of fine-grained psammite and thinly interbedded pelite with minor quartzite and chert (Sweetapple et al., 2001).
Two main sets of stacked pegmatite sheets occur, mostly 5 to 12 m thick, but ranging from 2 to 100 m and generally dipping 20 to 25°SE with localised 'roll-overs' dipping at 15 to 20°W to SW. These sheets are interconnected by near-vertical dykes that trend NW-SE and NE-SW, by irregular keel-like structures, and by thin stringers of pegmatite, all of which were emplaced at the same time. A third subparallel, 50 to 200 m thick sheet, has been encountered in drilling, although the base of this sheet is as much as 400 m below surface (Sweetapple et al., 2001). These pegmatite sheets were apparently syntectonically emplaced into a series of thrust faults that postdate at least two earlier deformation events identified by prominent cleavages and folding in the host metasedimentary rocks (Sweetapple and Collins, 2002).
Mount Cassiterite pegmatite appear to have a primary mineralogy and texture characteristic of albite-spodumene-type pegmatites, including an abundance of albite and primary spodumene with subordinate K feldspar and minor muscovite in near homogeneous sheeted bodies. The pegmatite sheets display a massive to comb-textured internal structure which Sweetapple and Collins (2002) regard as also being characteristic of albite-spodumene type pegmatites (Ginzburg and Lugovski, 1977; Cerny, 1992), with minor aplitic and K feldspar-rich layering.
These sheets are mostly unzoned, with a mineralogy dominated by megacrystic spodumene and perthitic microcline in a matrix of fine- to medium-grained quartz, albite and muscovite. Spodumene crystals are mostly aligned approximately perpendicular to the pegmatite contacts, typically exhibiting distinctive 'pull apart' structures. A weak zonation is evident as the development of finer grained border units, and occasional areas rich in microcline crystals.
Secondary assemblages, dominantly composed of fine-grained albite, variably overprint the assemblage outlined above in most areas of the pegmatite sheets. This alteration developed an accompanying pseudo-gneissic textured banding and syn-tectonic deformational textures. Cataclastic and proto-mylonitic textures are evident in places in the pegmatites. However, the micaceous minerals of the banding do not display a true schistosity, suggesting final crystallisation of the pegmatite took place under hydrostatic stress conditions, after the termination of deformation association with emplacement (Sweetapple, 2000).
The primary tantalum mineral assemblage is wodginite with subordinate manganotantalite and manganocolumbite and an alteration assemblage of stanniferous microlite, defect microlite, and traces of fibrous calciotantite [Ca(Ta,Nb)4O11] (Sweetapple et al., 2000). Cassiterite grains contain 5 to 8% Ta2O5 by way of charge balance substitution of Ta and Mn for Sn (Hall, 1994; Sweetapple et al., 2000).
There are distinct differences in whole rock geochemistry between the two different pegmatite suites (Sweetapple and Collins, 1998). The Wodgina pegmatite has a higher niobium:tantalum ratio than Mt. Cassiterite, which has a higher tin:tantalum ratio. These differences are reflected in the two different tantalum mineral suites in each pegmatite group. Significant differences were also noted in the gallium and beryllium contents, which were enriched and depleted respectively, in the Wodgina main lode pegmatite relative to the Mt. Cassiterite pegmatite group. The mineral assemblages in both pegmatite groups have undergone a significant degree of subsolidus recrystallisation (Sweetapple and Collins, 1998).
Beryl pegmatite group
This minor pegmatite group is on the southern periphery of the Mount Cassiterite pegmatite system, and is hosted by a sheared mafic volcanic unit. These pegmatites are up to 140 m long and 15 m wide at surface and display a simple zonation, with pale green beryl in discrete pods on the outer margins of quartz core structures enclosed by a muscovite-albite ±spessartine matrix (Sweetapple et al., 2001). Fine to coarse grained manganocolumbite with very fine microlite inclusions are also present in one of these pegmatites (Sweetapple et al., 2000).
North Hill pegmatite group
The North Hill pegmatite group lies directly to the north of the Cassiterite group, beneath the area known locally as North Hill. Details on the mineralogy, chemistry and association of these pegmatites is poorly understood, but may represent a continuation of pegmatite emplacement between the Cassiterite and the Wodgina pegmatite group to the north. The North Hill pegmatite group is hosted in a primary schist, although logging varies greatly in the area. This pegmatite group generally has lower Li O grades than the Cassiterite group, although mineralisation is developed over thicker drill intervals than observed in the Cassiterite pit. Internal pegmatite banding and mineralogy is largely unknown as evaluation and exploration has largely been by reverse circulation drilling. The trace element geochemistry of the North Hill pegmatite group varies from the Cassiterite deposit located immediately to the south, although it is inferred that the primary Li mineralogy is spodumene. The presence of economic mineralisation has yet to be established (SRK, 2023).
Reserves, Resources and Production
The Wodgina main lode pegmatite was mined out between 1988 and 1994 to produce:
0.402 Mt @ 1283 ppm Ta2O5, ~211 ppm Nb2O5 to yield 269 t of tantalum.
The Mount Cassiterite pegmatite has produced:
4.37 Mt @ 525 ppm Ta2O5, 292 ppm Sn02; to yield ~240 t of Ta.
Published interim global resource in April 1998 (Gwalia Consolidated, quoted in MBM Magazine, April 1999, pp. 52-55) were:
28 Mt @ 415 ppm Ta2O5.
The Wodgina Lithium deposit Ore Reserves and Mineral Resources as at December 31, 2022 (SEC Technical Report, Summary Initial Assessment, Wodgina, Western Australian prepared by SRK Consulting for Albemarle Corporation, 2023) were:
Indicated Mineral Resource - 17.60 Mt @ 1.31% Li2O;
Inferred Mineral Resource - 163.40 Mt @ 1.31% Li2O;
No Ore Reserves have been published.
This summary is partly drawn from "Sweetapple, M.T., 2000 - Characteristics of Sn-Ta-Be-Li-Industrial Mineral Deposits of the Archaean Pilbara Craton, Western Australia; Australian Geological Survey Organisation, Canberra, Record 2000/4, 59p." and
SRK Consulting (U.S.), Inc., 2023 - SEC Technical Report, Summary Initial Assessment, Wodgina, Western Australian prepared by SRK Consulting for Albemarle Corporation, 95p.
The most recent source geological information used to prepare this decription was dated: 2002.
This description is a summary from published sources, the chief of which are listed below. © Copyright Porter GeoConsultancy Pty Ltd. Unauthorised copying, reproduction, storage or dissemination prohibited.
Wodgina Mount Cassiterite
|
|
|
Sweetapple, M.T. and Collins, P.L.F., 2002 - Genetic Framework for the Classification and Distribution of Archean Rare Metal Pegmatites in the North Pilbara Craton, Western Australia: in Econ. Geol. v.97, pp. 873-895.
|
Porter GeoConsultancy Pty Ltd (PorterGeo) provides access to this database at no charge. It is largely based on scientific papers and reports in the public domain, and was current when the sources consulted were published. While PorterGeo endeavour to ensure the information was accurate at the time of compilation and subsequent updating, PorterGeo, its employees and servants: i). do not warrant, or make any representation regarding the use, or results of the use of the information contained herein as to its correctness, accuracy, currency, or otherwise; and ii). expressly disclaim all liability or responsibility to any person using the information or conclusions contained herein.
|
Top | Search Again | PGC Home | Terms & Conditions
|
|