Sunnyside |
|
Arizona, USA |
Main commodities:
Cu Mo Ag
|
|
 |
|
 |
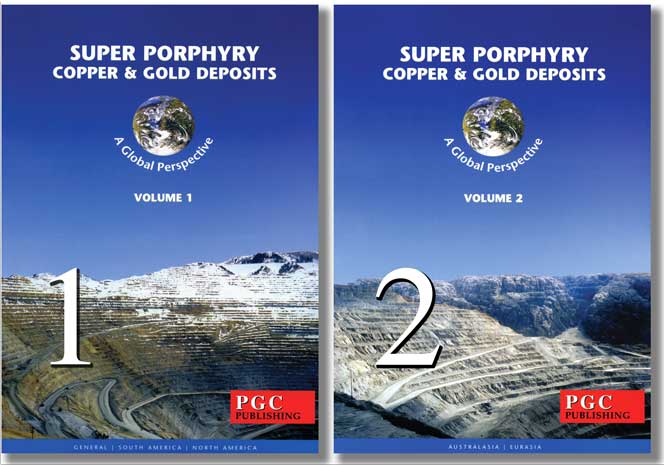 |
Super Porphyry Cu and Au

|
IOCG Deposits - 70 papers
|
All papers now Open Access.
Available as Full Text for direct download or on request. |
|
 |
The deep, sub-economic Sunnyside Cu-Mo deposit, is located ~4 km south of the similar Red Mountain Cu-Mo deposit and 2 km west of the centre of the Hermosa Zn-Ag-Pb deposits in the Patagonia Mountains of Santa Cruz County, ~8.5 km south of the town of Patagonia and 90 km SSE of Tucson in Arizona, USA (#Location: 31° 27' 47"N, 110° 44' 45"W).
Mining in the Patagonia Mountains dates back to the Spanish missionaries in the 1600’s (Chaffe et al. 1981). Subsequent to Arizona being ceded to the United States in 1848, portions of the district were mined as early as the 1850's. Modern exploration commenced in 1912, when the Magma Copper Company explored and mined the high-grade chalcocite ores at the 3R Mine, 2 km to the NW (Chatman, 1994). Some 190 t of ore with 9% Cu was shipped between 1912 and 1914.
In the early 1900s, the American Smelting and Refining Company, ASARCO, erected a custom smelter at the Trench Claims, 1.75 km east of the Sunnyside deposit. Mining is recorded at this mine from as early as 1858, with ASARCO operations undertaken from 1906 to 1962, with the bulk of the mining between 1939 and 1949.
The Red Hill (or Four Metals) Cu-Mo breccia mineralisation ~8 km to the south of Sunnyside was drill tested by a number of companies between 1929 and 1965. The Flux Canyon deposit, 2.5 km north of Sunnyside was drilled and mined by ASARCO to feed their smelter at Trench, initially in oxides but by 1944, at depths of ~130 m, Pb-Zn-Ag sulphide ore was becoming the primary economic target (Kartchner, 1944). Approximately 1.5 million tonnes of ore was
mined from the deposit between 1950 and 1962 at a combined grade of 7% Pb+Zn and 140 g/t Ag. During the 1950s to the early 1970s, major companies including Kennecott, Anaconda, Inspiration Mining Co., Phelps Dodge, Kerr McGee, West Range, Superior Oil. Utah Construction, Continental Copper, Duval Sulfur and Potash, Getty Oil, all held titles around and over the Sunnyside deposit and conducted drilling. A number had drilled moderate to deep holes, but none had explored the central portion over the deposit because of a confluence of claim boundaries. From 1971 to 1974, ASARCO consolidated all mineral rights in and around the
Sunnyside Project through various JV agreements (Graybeal, 1972). ASARCO undertook a drill program exploring both the shallow and deep portions of the Sunnyside porphyry copper-molybdenum system from 1974 to 1988. The last known exploration to 2017 occurred in 1993. The drilling by ASARCO essentially outlined the deep porphyry Cu-Mo and shallow high sulphidation lithocap mineralisation.
Geological Setting
The Sunnyside mineralised system is part of the Late Mesozoic to Tertiary Cananea-Mission Porphyry Trend, a broad, northwest trending, corridor of porphyry copper deposits in Mexico and the US. It also lies within the Basin and Range Province that covers most of the southwest United States and northwestern Mexico, characterised by linear, faulted mountain chains separated by broad flat valleys, the result of extension and thinning that began ~17 m.y. ago in the Miocene.
Sunnyside lies within one of these mountain chains, the Patagonia Mountain Range, which is composed of Proterozoic, Palaeozoic and Mesozoic sedimentary, granitic, and volcanic rocks. Latest Cretaceous to Palaeogene Laramide volcanic rocks, and a core of Laramide intrusions that comprise the Patagonia Mountains batholiths form the backbone of the range.
The oldest rocks in the range are Proterozoic granodiorite unconformably overlain by the Cambrian Bolsa quartzite and Abrigo Formation. These are unconformably overlain by the Upper Devonian Martin Formation carbonates, followed by the Lower Carboniferous Escabrosa Limestone and Upper Carboniferous to Lower Permian principally carbonate successions. These are all overlain by Triassic and/or Jurassic silicic volcanics rocks composed of rhyolitic lava, welded tuff, and interlayered tuff. In the central region of the range, the Cretaceous aged Bisbee Formation comprises siltstone and mudstone with thin limestone beds, resting discomformably on Triassic and Jurassic silicic volcanic rocks. The youngest rocks are late Cretaceous or early Tertiary in age and comprise silicic tuff, tuff breccia, and lavas (Simons, 1972).
See the Geological Setting section and geological map in the Hermosa record for a more detailed stratigraphy.
The ~74 to 58 Ma Laramide multi-phase intrusive complex that forms the core of the Patagonian Range comprises quartz monzonite to granodiorite and lesser quartz-feldspar porphyry. It extends for ~19 km north from the Mexican border, before it thins and terminates against Mesozoic granitic and volcanic intrusion breccias just to the north of Sunnyside. Younger Cenozoic-aged volcanics were deposited over the northern portion of the range and are likely related to the extension that produced the Basin and Range province. Normal faulting that juxtaposes rocks of different ages is typical of the Basin and Range province. The major such fault zone along the Patagonia Range is the NW trending Harshaw Creek Fault that represents >3000 m of stratigraphic displacement, with Proterozoic crystalline rocks along the western front, whilst the eastern part of the range exposes complexly faulted Palaeozoic to Mesozoic sedimentary and volcanic rocks. This structure projects into rocks that comprise the Sunnyside hydrothermal system. A second structure, the Guajolote Fault, may have controlled the emplacement of the NW trending batholiths, and is well exposed in prominent shears at the 3R Mine, 2 km to the northwest. These structures are part of the 3R Shear Zone, a 6.5 km long by up to 1 km wide corridor of brittle shear trending at 345°, passing through the 3R mine on its western margin, whilst its eastern edge passes through the Sunnyside deposit. It deforms all Mesozoic rocks, with the oldest being most affected, decreasing in intensity with age to being almost absent in Laramide intrusions. A third major structural feature, a normal fault is evident in the stratigraphic displacement of Quaternary colluvial deposits and volcanic rocks on the north-western side of Red Mountain. The alignment of the mountain range, the elongation of the Laramide age batholiths, as well as the strike of the main structural features and mineral deposits of the Cananea-Mission trend in the Patagonia Mountains have been interpreted to imply the presence of a deep crustal structure within the Laramide magmatic arc (Vikre et al., 2014).
Geology, Alteration and Mineralisation
The oldest rocks overprinted by the Sunnyside mineralised system are to the west, and include a package of Proterozoic crystalline rocks that comprise both meta-intrusive and meta-sedimentary rocks, intruded by batholithic Jurassic granitoids. To the east, Cretaceous sedimentary and volcanic rocks overlie Triassic to Jurassic volcano-sedimentary sequences that, in turn, overlie Palaeozoic limestone and shale units that have been complexly folded and faulted, likely related to Laramide tectonics. A little to the south these Palaeozoic rocks overlie Proterozoic basement. The central section, between these two suites, comprises a composite, multiphase Laramide intrusive complex largely intruding a Triassic to Jurassic volcano-sedimentary sequence. This intrusive complex is composed of granodiorite to quartz monzonite with late quartz-feldspar-porphyry intrusions (Graybeal, 1996; Vikre et al., 2014; Turner, 2017).
In the immediate deposit area, separate quartz-feldspar-porphyry and quartz monzonite to quartz monzonite porphyry occurs as a series of elongated dyke-like bodies and irregular plugs. To the south, the 64 to 60 Ma quartz monzonite to quartz monzonite porphyry bodies coalesce at surface into a larger, NNW-SSE elongated 9 x 1 to 2 km intrusion. Within the Sunnyside porphyry Cu-Mo system, these quartz-monzonitic intrusions have been pervasively altered to quartz-sericite-alunite-kaolinite. Drilling has shown that the younger, and physically separated quartz-feldspar-porphyry intrusions exposed at the surface, coalesce at a depth of ~1.5 km into a single, roughly circular plug that is ~1.6 km in diameter (Graybeal, 1996). This latter porphyry was intruded as multiple phases in the age range 59.94 ±0.85 to 60.0 ±1.4 Ma (U-Pb zircon). At the surface, the quartz-feldspar-porphyry contains up to 20 vol.% anhedral-resorbed to euhedral, clear, unbroken quartz phenocrysts and up to 50 vol.% euhedral feldspar phenocrysts, all set in a matrix altered to a fine-grained sericite-illite assemblage. Drilling in sections of the quartz-feldspar-porphyry reveal the resorbtion textures in quartz phenocrysts die out rapidly with depth, and by 1.5 km below the surface, quartz occurs as well rounded crystals accompanied by tabular feldspar phenocrysts in a matrix of locally granophyric quartz and orthoclase (Graybeal, 1996; Vikre et al., 2014; Turner, 2017).
At surface, a roughly circular, ~1.6 km diameter mass of Paleocene volcaniclastic rocks, also referred to as 'the lapilli tuff', is exposed. It is interpreted to be coeval with the later phases of the quartz-feldspar-porphyry which crops out on its northern and western margins and centre, while the deep coalesced porphyry body is centred below the western rim of the volcaniclastic rocks. The volcaniclastic rocks are well consolidated and poorly sorted lapilli tuff and tuff breccia. Lithic clasts in the lapilli tuff are generally angular, <2.5 cm in diameter, and largely comprise Mesozoic volcanic and sedimentary rocks. These are accompanied by 35%, mostly broken, clear quartz crystals with strongly resorbed boundaries on preserved crystal faces. Minor fragmented feldspar phenocrysts are also present. The lapilli are matrix supported and form ~30% by volume of the lapilli tuff, and with the crystals and lithic clasts, are set in a fine-grained, illite-alunite-kaolinite-altered matrix (Graybeal et al., 2015), interpreted to be after ash. It also contains numerous silicified zones, both transgressive and conformable. The bedded volcaniclastic sequences have a concentric strike and inward dips. On the outer margins, dips are shallow, but toward the centre of the exposed mass dip at up to 70°. Drilling shows it persist to depths of as much as 1 km below the surface with steeply dipping walls and at depth may become a xenolithic-rich intrusion to explosive breccia. It is interpreted to represent tephra that occupies a >0.7 km diameter vent formed as a result of explosive fragmentation of rising volatile-rich magma during a phreatomagmatic eruption. The flat lying outer margins are interpreted represent the area where ejecta overflowed the crater edge. Intrusive breccia masses to the southwest of these volcaniclastic rocks are texturally similar to the volcaniclastic rocks and contain locally abundant quartz monzonlte porphyry clasts up to 30 cm across (Graybeal 1996; Graybeal et al., 2015).
Drilling has indicated the deep coalesced quartz-feldspar-porphyry stock hosts porphyry-style chalcopyrite-molybdenite mineralisation at a depth of >1100 m, associated with potassic alteration. Mineralisation occurs as an inverted shell (convex up) ~250 to 300 m thick, with a diameter of ~900 m and vertical extent of ~800 m. Remnants of a potassic alteration assemblage become common at depths of 1.2 to 1.5 km and include K feldspar in veins and shreddy biotite, apatite, magnetite, hematite and chalcopyrite in replacement envelopes peripheral to quartz-pyrite-chalcopyrite veins. The potassic alteration assemblage and associated chalcopyrite mineralisation are largely confined to the quartz-feldspar porphyry, but are strongly overprinted by a younger phyllic assemblage. The mineralised zone is mostly restricted to the quartz feldspar porphyry and to a lesser extent adjacent earlier quartz monzonite porphyry. Chalcopyrite has undergone local hypogene oxidation to magnetite and hematite. Minor chalcopyrite extends up into the overlying phyllic zone, but does not overlap into the enargite zone above (Graybeal, 1996; Vikre et al., 2014).
At a similar depth of between 1.2 and 1.6 km, skarns in Palaeozoic carbonate rocks intruded by the quartz-feldspar porphyry contain intervals of ~1% Cu, 3% Pb, 10% Zn and 300 g/t Ag over widths of 15 to 30 m. These intersections are below the Triassic to Jurassic Older Volcanic sequence and the Tertiary volcaniclastic rocks, and are >1 km down dip from the Taylor Deeps deposit of the Hermosa deposits.
Phyllic alteration overlies and is superimposed upon the deep porphyry mineralisation. This zone covers an extensive area of many square kilometres outward and upward from the deep quartz-feldspar-porphyry stock, overlain by a more restricted, up to 450 m thick, keel of advanced argillic alteration, separated by a broad transition. Both alteration zones are centred on or above the deep, mineralised quartz feldspar porphyry. Phyllic alteration extends to depths of 1.6 km or more within and below the western side of the volcaniclastic/lapilli tuff unit. At a depth of 900 m, the phyllic zone is ~1.6 km wide, but expands rapidly upwards in all directions to >3 km from west to east at surface. It is distributed asymmetrically around the 0.3% Cu shell of the deep porphyry mineralisation. It broadly corresponds to the zone of pyritisation shown on the accompanying geological map, and is dominated by a pervasive sericite-pyrite assemblage with locally abundant quartz-tourmaline. Beyond the margins of the pervasive pyrite-sericite zone, 6 to 10 mm thick veins of quartz-sericite-pyrite are common, generally oriented at 55 to 70° forming two wings, one to the west and the second to the east, encompassing the Trench mine and sections of the Hermosa deposits. Identical quartz-sericilc-pyrite veins are also common below the zone of advanced argillic alteration (Graybeal, 1996; Vikre et al., 2014). No significant mineralisation occurs within the phyllic zone, although small amounts of enargite occur its uppermost zones, increasing in intensity upwards into the transition and throughout the advanced argillic alteration, occurring as disseminated grains in silicified zones and in breccias.
An outermost, weak, erratically developed zone of propylitic alteration is found laterally beyond the potassic and phyllic zones, and is of unknown extent.
The advanced argillic alteration zone is a relatively thin cap, not more than 450 m thick. The advanced argillic assemblage comprises alunite-kaolinite-diaspore-zunyite with erratic enargite-tennantite-pyrite and extensive intensely silicified zones. Traces of andalusite can be seen in thin section, as well as rare pyrophyllite only seen by x-ray methods. Sericite is absent, other than in the transition to the phyllic zone. There are no veins of any kind within the advanced argillic zone, and primary rock textures are commonly obliterated. The alteration zone is coincident with strong and continuous anomalies values in rock of F (>I000 ppm) and Mo (>4 ppm), and very erratic As values. Gold is essentially absent, rarely exceeding 0.04 g/t. An ~600 x 300 m area of strong silicification is colllcident with the advanced argillic zone along the west side of the volcaniclastic/lapilli tuff unit. Whilst ghosts of protolith textures are visible, this rock is composed of 95 to 100 vol.% fine grained silica. Similar more extensive zones of silicification are widespread in the Triassic to Jurassic Older Volcanic suite, extending for a radius of as much as 25 km from the Sunnyside deep porphyry. A near-surface hypogene zone of mineralisation is developed within the advanced argillic alteration zone, characterised by an assemblage of enargite-tennantite-covellite resource. This has been subjected in part to supergene chalcocite enrichment to produce a combined high sulphidation sulphide and chalcocite blanket resource, as detailed below in the resources section. This assemblage continues into the surrounding Mesozoic rocks and in part overlies the more weakly mineralised phyllic alteration zone (Graybeal, 1996; Vikre et al., 2014). Oxidation extends to depth of 180 to 200 m within the 3R Shear Zone, but elsewhere within the mineralised system, supergene chalcocite is limited to depths of 3 to 15 m averaging 0.2 to 0.5% Cu (Turner, 2017).
Vikre et al. (2014) suggest there was a third late mineralisation pulse that produced deep to shallow veins containing molybdenite and veins containing sphalerite.
The observations above suggest the mineralisation at Sunnyside was introduced during the intrusion of a number of pulses of the Paleocene quartz-feldspar porphyry. The first phase involved the development of the Sunnyside deep porphyry Cu-Mo deposit within the quartz-feldspar porphyry intrusive complex, the result of a number of co-spatial, juxtaposed intrusive and hydrothermal fluid events sourced from a deep parental magma chamber. The last of the intrusions related to this first stage propagated to shallower depths and erupted in a phreatomagmatic explosion, forming a vent complex filled by the volcaniclastic tuff unit. This event led to decompression of the porphyry system, boiling and the separation of the vapour phase, as indicated by fluid inclusions within the quartz-feldspar porphyry and volcaniclastic tuff at depths shallower than 600 m (Graybeal 1996) and a change in pH. These changes and opening of the conduit to shallower levels allowed the widespread distribution through permeable volcanic rocks of differentiated fluids from the apex of the large, deep parental magma chamber. The low pH vapours and following fluids produced the advanced argillic and phyllic lithocap and deposition of the high sulphidation enargite-tennantite-covellite mineralisation. The late magmatic-hydrothermal event evolved into fluids that deposited Zn-Pb-Ag mineralisation both proximal and distal to the quartz-feldspar porphyry, as at the Hermosa Taylor and Clark deposits. Uplift, erosion and weathering during the development of the Basin and Range province produced a weak supergene enriched blanket from the shallow high sulphidation assemblage in the lithocap.
Resources
Non-JORC and non-NI 43-101 resources have been estimated from pre-1993 drilling, as follows:
Deep porphyry Cu-Mo mineralisation:
- 1.5 Gt @ 0.33 wt.% Cu, 0.011 wt.% Mo, 5 g/t Ag at a 0.2% Cu cutoff, Vikre et al. (2014);
- 150 to 200 Mt @ 0.6 wt.% Cu, 0.02 wt.% Mo, 4.5 g/t Ag, (Graybeal, 1996).
Shallow supergene chalcocite and hypogene high sulphidation mineralisation:
- 800 Mt @ 0.175 wt.% Cu, 7 g/t Ag (Graybeal and Vikre, 2010)
This summary is drawn in part from "Turner, A., 2017 - Technical report on the Sunnyside Project, Santa Cruz County, Arizona, USA; An NI 43-101 Technical report, prepared for Regal Resources Inc. and Barksdale Capital Corp. by APEX Geoscience Ltd. Edmonton AB Canada, 95p."
The most recent source geological information used to prepare this decription was dated: 2017.
This description is a summary from published sources, the chief of which are listed below. © Copyright Porter GeoConsultancy Pty Ltd. Unauthorised copying, reproduction, storage or dissemination prohibited.
Sunnyside
|
|
|
Graybeal, F.T., 1996 - Sunnyside A Vertically-Preserved Porphyry Copper System, Patagonia Mountains, Arizona: in SEG Newsletter No.26, p. 1, 10-14.
|
Graybeal, F.T., Moyer, L.A., Vikre, P.G., Dunlap, P. and Wallis, J.C., 2015 - Geologic map of the Patagonia Mountains, Santa Cruz County, Arizona: in U.S. Geological Survey, Open-File Report 2015-1023, 10p.
|
Vikre, P.G., Graybeal, F.T., Fleck, R.J., Batron, M.D. and Seedorff, E., 2014 - Succession of Laramide Magmatic and Magmatic-Hydrothermal Events in the Patagonia Mountains, Santa Cruz County, Arizona: in Econ. Geol. v.109, pp. 1667-1704.
|
Porter GeoConsultancy Pty Ltd (PorterGeo) provides access to this database at no charge. It is largely based on scientific papers and reports in the public domain, and was current when the sources consulted were published. While PorterGeo endeavour to ensure the information was accurate at the time of compilation and subsequent updating, PorterGeo, its employees and servants: i). do not warrant, or make any representation regarding the use, or results of the use of the information contained herein as to its correctness, accuracy, currency, or otherwise; and ii). expressly disclaim all liability or responsibility to any person using the information or conclusions contained herein.
|
Top | Search Again | PGC Home | Terms & Conditions
|
|