Tennant Creek - Gecko, Warrego, White Devil, Nobles Nob, Juno, Peko, Argo, Orlando, TC8 |
|
Northern Territory, NT, Australia |
Main commodities:
Au Cu Bi
|
|
 |
|
 |
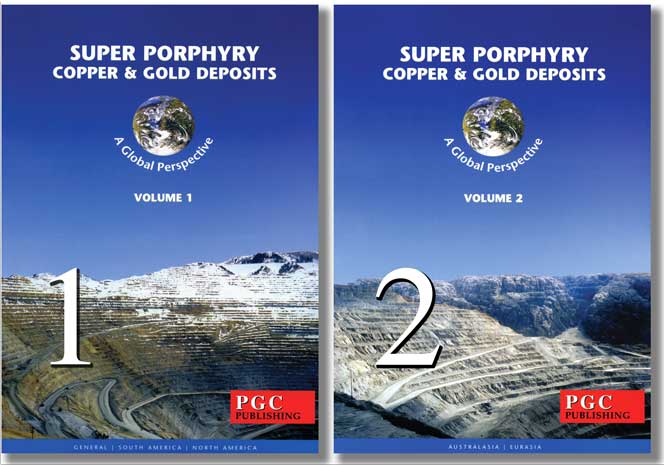 |
Super Porphyry Cu and Au

|
IOCG Deposits - 70 papers
|
All papers now Open Access.
Available as Full Text for direct download or on request. |
|
 |
The Tennant Creek gold field is located around the town of Tennenat Creek, some 900 km SSE of Darwin in the Northern Territory of Australia. It falls within the central province of the Tennant Creek Inlier, surrounded by a flat lying Lower Palaeozoic carbonate sequence.
The Inlier hosts a cluster of small, but high grade gold-copper-bismuth deposits, associated with magnetite and/ or hematite ironstones, that are distributed over an area of ~70 x 50 km. Examples of the largest and highest grade deposits include Peko (3.2 Mt @ 4% Cu, 3.5 g/t Au, 0.2% Bi, 14 g/t Ag) and Juno (0.45 Mt @ 0.4% Cu, 57 g/t Au, 0.6% Bi, 7 g/t Ag). The total production to 2000 was in excess of 156 tonnes of gold and 345 000 tonnes of copper since mining commenced in the 1930s.
Other key deposits include Gecko, Warrego, White Devil, Nobles Nob, Juno, Peko, Argo, Orlando and TC8
Geological Setting
The Palaeoproterozoic Tennant Creek Inlier is elongated in a northnorthwest to northwest direction, covering an area of ~44 000 km2, ~500 km west of the Mount Isa Inlier (Le Messurier et al., 1990). It is composed of three elements, the central of which, the Warramunga Province, hosts all of the mineralisation. The mineralised province is bounded to the north and south by overlying sequences of the coeval Ashburton and Davenport provinces respectively, while the inlier as a whole is surrounded by flat lying, mainly carbonate facies Cambrian rocks of the Wiso and Georgina basins to the west and east respectively, and by Mesozoic and younger cover (Shaw et al., 1996).
The exposed Warramunga Province is the central portion of a largely concealed block of Palaeoproterozoic rocks that extends over an area of ~350 x 60 km, elongated in a eastsoutheast direction (Shaw et al., 1996), almost perpendicular to the trend of the inlier, but parallel to, and approximately 200 km north of the southern boundary of the North Australia craton. Assembly of this craton commenced prior to 1.9 Ga, to be consolidated and cratonised by ~1.83 Ga, although repeated terrane accretion and orogenic activity continued along its southern margin to ~1.40 Ga (Plumb 1979, Myers et al., 1996).
Deposition within the Warramunga Province commenced with a thick turbidite succession, the Warramunga Formation, which is unconformably overlain by sedimentary and felsic volcanic rocks of the Ooradidgee Group. Pre-Warramunga Formation basement is not exposed, although drill holes 30 km to the south west of the exposed Warramunga Province have intersected almandine-amphibolite facies gneisses with a Rb-Sr age of 1920±60 Ma, and Sm-Nd and Rb-Sr modal ages of crystallisation of at least 2.4 Ga .
The Warramunga Formation, which is ~3000 m thick, was deposited in a rift basin at ~1.86 to 1.85 Ga, and hosts the bulk of the known mineralisation of the Tennant Creek Inlier (Donnellan et al., 1994; Compston 1995). It is composed of turbiditic greywacke, siltstone and shale, which are in part tuffaceous, and notably iron-rich. The sequence contains abundant disseminated detrital and metamorphic magnetite, and numerous thin hematitic carbonate-bearing siltstones and shales, including an argillaceous banded ironstone that is known locally as the 'hematite shale'. An interbedded dacite lava is dated at 1862±5 Ma (Smith, 2001).
The angular unconformity at the top of the Warramunga Formation corresponds to the Tennant Event, when the sequence was metamorphosed to greenschist facies and the ~1850 Ma (Page 1995) Tennant Creek Super-suite was emplaced, comprising syn- to post-tectonic I-type granitoids, quartz-feldspar porphyry and minor mafic intrusions. This magmatism and tectonic activity represents the early stages of the Barramundi Orogeny, that is recognised across northern Australia (Donnellan 2005; Donnellan et al., 1994; Compston 1995; Skirrow, 2000; Stolz and Morrison, 1994; Etheridge et al., 1987).
The up to 5000 m thick Ooradidgee Group immediately overlies the Tennant Event unconformity and comprises a suite of felsic volcanic rocks, predominantly dacitic to rhyolitic ignimbrites, (but with broadly coeval basalts, representing bimodal volcanism) with associated felsic sills and a possible monzodiorite, emplaced between 1845±4 and 1827±9 Ma (Compston, 1995). The sequence was emplaced within a continental rift setting (Hussey et al., 1994), associated with continuing, localised extension belonging to the late stages of the Barramundi orogenic cycle. The sequence also includes sandstones, siltstones, shales, cherts and tuff. Deposition ceased soon after the emplacement of the ~1820 Ma Treasure Suite granites, granophyre, and monzodiorite-diorite (and comagmatic volcanic rocks) and the variably developed 1810 Ma Murchison Event unconformity which separates the Ooradidgee and the Tomkinson Creek and Hatches Creek groups. In places this contact appears to be transitional, with volcanism and any break in deposition diachronously migrating southward. This event also marks the transition from rift to sag phase sedimentation in the Davenport geosyncline (Smith, 2001, and sources cited therein).
The temporally equivalent Tomkinson Creek and Hatches Creek groups are composed of thick, well stratified sequences, respectively comprising ~6 km of silicified marine sandstone in the Ashburton Province to the north, and in the Davenport Province to the south, up 10 000 m of sandstone, siltstone and shale, with felsic volcanic rocks and basaltic lava towards the base. Both of these groups were terminated by the ~1720 Ma Davenport Event which was accompanied by a suite of post-mineralisation 1720 to 1710 Ma S-type granites (Page, 1995; Smith, 2001, and sources cited therein).
Alteration and Mineralisation
Mineralisation within the Tennant Creek field is associated with ironstone bodies, characterised by ellipsoidal, lens, cigar-like and irregular geometries, that range in size from a few tonnes to several tens of millions of tonnes. In excess of 650 ironstone bodies are recorded in the Inlier, although only 25% contained any ore grade Cu, Au or Bi, many of which were only very minor in size, and only 100 of these have been mined in any way. Barren ironstones are dominantly composed of magnetite and/or hematite with variable amounts of quartz, chlorite, dolomite and talc, whereas those that are mineralised, also contain, sulphides, sulphosalts, and minor muscovite. The ironstones display a range of textural variations, including iron oxides that occur as: i). massive fine to medium grained intergrowths; ii). diffusely-banded to mottled with quartz and chlorite; iii). fine-grained, colloform-banded varieties with quartz; and iv). skeletal and growth-zoned magnetite. Both replacement and infill textures are evident (Wall and Valenta, 1990; Davidson and Large, 1998). Large (1975) observed microtextures suggesting the earliest assemblages involved hematite or hydrated iron-oxides, which were overprinted by magnetite.
Both the barren and mineralised ironstones are zoned, grading downward from massive accumulations, into root zones of replacement and networks of magnetitequartz± hematite stringer veins, together forming pipe-like geometries that may persist in some cases for up to several hundred metres vertically, all of which are enveloped by chloritic alteration extending for centimetres to metres out from the iron oxides. Talc and dolomite alteration are also observed, and both host sediments and ironstones are frequently variably brecciated on a deposit- to local-scale (Skirrow, 2000; Skirrow & Walshe, 2002).
Ironstones generally vary from transgressive to bedding localised in a variety of structural settings including antiformal fold closures, faults, shears and contacts of felsic porphyry intrusions (Ivanac, 1954), particularly in conjunction with contacts between rheologically contrasting lithologies. A strong structural control is also indicated by several east-west lines or trends of ironstones. Some ironstones are also spatially related to locally calcite-bearing 'hematite shale' units which may have acted as chemical triggers to ironstone precipitation where they were encountered by conduits carrying magnetite-stable fluids (Large, 1975; Wedekind et al., 1989).
Well developed S1 foliation in the chlorite alteration suggests early- to syn- D1 timing for the development of the ironstones. The colloform textures of early hematite and/or hydrated iron oxide deposition (Large, 1975) are consistent with a relatively low temperature of initial formation, supported by fluid inclusion homogenisation temperatures of 120 to 180°C (not pressure-corrected; Khin Zaw et al., 1994; Huston et al., 1993; Skirrow, 1993). However, calculated chlorite formation temperatures, and oxygen isotope geothermometry on quartz-magnetite pairs, in both barren and mineralised ironstones, indicate a temperature of formation of ~300 to 400°C, for magnetite-chlorite replacing the early hematite assemblage (Skirrow, 1993), consistent with formation during lower greenschist facies regional metamorphism (Wall and Valenta, 1990). The ironstone bodies are also calculated to have formed from hot (350 to 400°C) NaCl+CaCl2 brines at pressures of ~2.5 to 5 kb (Wedekind and Love, 1990; Skirrow and Walshe, 2002).
Khin Zaw et al. (1994), Huston et al. (1993), Skirrow (1993) and Skirrow and Walshe (1994) agree that the fluids responsible for ironstone-formation were calcic brines with 10 to 20 wt.% NaClfluid equiv..
Although deposits across the district share many key characteristics, they have widely varying Au:Cu and magnetite:hematite ratios, iron-sulphide mineralogies, and sulphur isotope composition (Wedekind et al., 1989; Wall and Valenta, 1990; Huston et al., 1993; Skirrow, 1993; Davidson and Large, 1998). These variations may be regarded as representing a spectrum of ironstone-related styles between two compositional end-members: i). reduced (magnetite-pyrrhotite), copper rich deposits with narrow ranges of δ34S sulphide values (0 to 4‰) and magnetite-muscovite-Mg chlorite alteration assemblages (e.g., West Peko), and ii). oxidised (hematite-magnetite± pyrite), gold-rich deposits with wide ranges of δ34Ssulphide values (-15 to 7‰) and hematite-chlorite-muscovite±pyrite alteration (e.g., Eldorado; Skirrow & Walshe, 2002). Many of the larger, high grade gold deposits lie between these extremes, characterised by minerals of intermediate oxidation-reduction state (Skirrow, 2000).
At the West Peko deposit, gold-copper-bismuth is interpreted to have formed at 300 to 340°C from pyrrhotite±magnetite-stable, weakly acidic, sulphur- and N2±CH4-rich, low salinity (3 to 10 wt.% NaCl equiv.) hybrid metamorphic and/or formation fluids (Skirrow, 1999; Skirrow and Walshe, 2002). The same authors suggest these fluids flowed along shear and fault zones which trapped reduced rock packages below oxidised Warramunga Formation, with gold and bismuth deposited in response to desulphidation±oxidation of the reduced fluid as it reacted with magnetite±hematite ironstone. They also suggest desulphidation could have been driven by precipitation of chalcopyrite, pyrite, pyrrhotite and bismuth-sulphides or sulphosalts in response to increased pH of the fluids (Skirrow, 1999; Skirrow and Walshe, 2002).
Mineralisation at Eldorado is interpreted to have formed from an intermediate fO2, low- to moderate-salinity fluid, which mixed with hematite-stable Ca-Na-Cl brine in the presence of magnetite-rich ironstone (Skirrow and Walshe, 2002). Fluid mixing likely caused an increase in copper solubility leading to undersaturation of copper minerals and the deposition of copper-deficient gold-bismuth mineralisation.
According to Skirrow (2001), with the exception of several data from West Peko, all oxygen-hydrogen isotopic compositions of fluids lie outside and above the generally accepted field for magmatic waters (δ18Ofluid = 0 to 6‰; δDfluid = -40 to -10‰; Wedekind, 1990; Huston, 1991; Skirrow, 1993). Input of evolved surficial waters (e.g., formation waters or their higher temperature equivalents) appears necessary to generate the oxygen-hydrogen isotopic compositions, but contributions of magmatic or metamorphic waters cannot be precluded. All of the textures and mineral relationships point to the copper and iron sulphides, bismuth minerals (including bismuthinite and a range of seleniferous Bi±Pb sulphosalts; Large, 1975), and gold overprinting the magnetite-rich ironstones.
Published 40Ar/39Ar ages for gold-copper-bismuthmineralisation in the Tennant Creek region range from 1825 to 1829 Ma, apparently younger than the timing of deformation bracketed by 207Pb/206Pb zircon ages of igneous rocks at ~1850 Ma. Recalculation using revised estimates of the 40K decay constant and age of the 40Ar/39Ar standard shifts the 40Ar/39Ar ages to 1851 to 1847 Ma, in excellent agreement with 207Pb/206Pb zircon ages from Tennant Supersuite igneous rocks and with local geological constraints (Fraser et al., 2008) and the Barramundi orogenic cycle volcanic rocks of the Ooradidgee Group. This implies the Tennant Event metamorphism and continuing magmatism is coeval with mineralisation.
District-wide iron oxide alteration is evident, although no regional sodic alteration has been recognised in the Warramunga Province, possibly refl ecting the apparent lack of evaporites in the sequence. Strongly developed deposit scale magnetite-hematite with chlorite-muscovite and biotite alteration is characteristic of the ores. Ore fl uid temperatures of ~340 to 400°C for the earlier ironstone formation during early D1 and ~300 to 350°C for the late D1 emplacement of sulphide mineralisation are generally lower than in many IOCG sensu stricto deposits.
Historical Tennant Creek Mine Production (after Emmerson Resources, 2014)
Nobles Nob (1947-86) 1.996 Mt @ 17.3 g/t Au | Juno (1967-77) 0.452 Mt @ 56.1 g/t Au |
White Devil (1987-99) 1.618 Mt @ 14.6 g/t Au | Eldorado (1934-93) 0.212 Mt @ 17.9 g/t Au |
Argo (1986-88) 0.257 Mt @ 10.0 g/t Au | Chariot (2003-05) 0.2537 Mt @ 10.9 g/t Au |
Golden Forty (1974-86) 0.104 Mt @ 14.0 g/t Au | TC-8 (1986-88) 0.081 Mt @ 17.6 g/t Au |
Northern Star (1934-88) 0.101 Mt @ 7.3 g/t Au | Malbec West (2005) 0.0359 Mt @ 17.8 g/t Au |
Small Mines (1934-87) 0.127 Mt @ 26.6 g/t Au | Warego Tailings (1994-98) 5.300 Mt @ 0.7 g/t Au |
 |
Warrego (1972-89) 6.750 Mt @ 6.6 g/t Au, 1.9% Cu | Peko (1654-76) 3.676 Mt @ 3.5 g/t Au, 4 % Cu |
Orlando (1962-99) 0.736 Mt @ 7.9 g/t Au, 3.1% Cu | Gecko (1980-99) 2.930 Mt @ 0.6 g/t Au, 3.6% Cu |
Ivanhoe (1965-72) 0.321 Mt @ 3.0 g/t Au, 3.7 % Cu |
 |
TOTAL production 24.951 Mt @ 6.9 g/t Au, 2.8% Cu |
a. Regional geological setting of the Tennant Creek ore deposits (after Geological Map of the Northern Territory, 2006, Northern Territory Geological Survey).
b. Stylised cross section through the Juno orebody showing the geology, alteration and distribution of mineralisation (after Wedekind et al., 1989).
The most recent source geological information used to prepare this decription was dated: 2010.
Record last updated: 21/10/2014
This description is a summary from published sources, the chief of which are listed below. © Copyright Porter GeoConsultancy Pty Ltd. Unauthorised copying, reproduction, storage or dissemination prohibited.
Gecko Orlando Warrego White Devil Peko Juno Nobles Nob
|
|
Anonymous 1998 - Normandy Mining Limited: in Extracts from the Normandy Mining Limited 1997 Annual Report pp 12-14, 17, 26-28.
|
Anonymous 1998 - Tennant Creek Operations: in Register of Australian Mining 1997/98 p 209
|
Anonymous 1998 - Normandy Mining Limited: in Extracts from the Normandy Mining Limited 1997 Annual Report pp 12-15, 26-28
|
Bosel C A, Caia G P 1998 - White Devil gold deposit: in Berkman D A, Mackenzie D H (Eds), Geology of Australian and Papua New Guinean Mineral Deposits The AusIMM, Melbourne pp 439-442
|
Crohn P W 1975 - Tennant Creek-Davenport Proterozoic Basins - regional geology and mineralization: in Knight C L, (Ed.), 1975 Economic Geology of Australia & Papua New Guinea The AusIMM, Melbourne Mono 5 pp 421-424
|
Edwards G C, Booth S A, Cozens G J 1990 - White Devil Gold deposit: in Hughes F E (Ed.), 1990 Geology of the Mineral Deposits of Australia & Papua New Guinea The AusIMM, Melbourne Mono 14, v1 pp 849-855
|
Ferenczi P A, Ahmad M 1998 - Geology and mineral deposits of the Granites-Tanami and Tennant Creek Inliers, Northern Territory: in AGSO J. of Aust. Geol. & Geophys. v17, no. 3 pp 19-33
|
Goulevitch J 1975 - Warrego copper-gold orebody: in Knight C L, (Ed.), 1975 Economic Geology of Australia & Papua New Guinea The AusIMM, Melbourne Mono 5 pp 430-436
|
Hill J H 1990 - TC8 Gold deposit: in Hughes F E (Ed.), 1990 Geology of the Mineral Deposits of Australia & Papua New Guinea The AusIMM, Melbourne Mono 14, v1 pp 857-859
|
Huston D L, Bolger C, Cozens G 1993 - A comparison of mineral deposits at the Gecko and White Devil deposits: implications for ore genesis in the Tennant Creek district, Northern Territory, Australia: in Econ. Geol. v88 pp 1198-1225
|
Large R R 1975 - Juno gold-bismuth mine, Tennant Creek: in Knight C L, (Ed.), 1975 Economic Geology of Australia & Papua New Guinea The AusIMM, Melbourne Mono 5 pp 424-430
|
Le Messurier P, Williams B T, Blake D H 1990 - Tennant Creek Inlier - regional geology and mineralisation: in Hughes F E (Ed.), 1990 Geology of the Mineral Deposits of Australia & Papua New Guinea The AusIMM, Melbourne Mono 14, v1 pp 829-838
|
Main J V, Nicholson P M, O Neill W J 1990 - K44 Ironstone Copper-Gold deposit, Gecko Mine: in Hughes F E (Ed.), 1990 Geology of the Mineral Deposits of Australia & Papua New Guinea The AusIMM, Melbourne Mono 14, v1 pp 845-848
|
Skirrow R G 2000 - Gold-copper-bismuth deposits of the Tennant Creek district, Australia: A reappraisal of diverse high-grade systems: in Porter T M (Ed), 2000 Hydrothermal Iron Oxide Copper-Gold & Related Deposits: A Global Perspective PGC Publishing, Adelaide v1 pp. 149-160
|
Skirrow R G, Walshe J L 2002 - Reduced and oxidized Au-Cu-Bi Iron Oxide deposits of the Tennant Creek Inlier, Australia: an integrated geologic and chemical model: in Econ. Geol. v97 pp 1167-1202
|
Skirrow, R.G., 2021 - Iron oxide copper-gold (IOCG) deposits - a review (part 1): settings, mineralogy, ore geochemistry, and classification within the Cu-Au-Fe (±Co, REE) deposit family: in Preprint accepted Nov 2021, for Ore Geology Reviews, 71p. doi.org/10.1016/j.oregeorev.2021.104569
|
Wedekind M R, Large R R, Williams B T 1995 - Controls on high grade gold mineralisation at Tennant Creek, Northern Territory, Australia: in Econ. Geol. Mono. 6 pp 168-179
|
Wedekind M R, Love R J 1990 - Warrego Gold-Copper-Bismuth deposit: in Hughes F E (Ed.), 1990 Geology of the Mineral Deposits of Australia & Papua New Guinea The AusIMM, Melbourne Mono 14, v1 pp 839-843
|
Williams, P.J. and Pollard, P.J., 2003 - Australian Proterozoic Iron Oxide-Cu-Au Deposits: An Overview with New Metallogenic and Exploration Data from the Cloncurry District, Northwest Queensland: in Exploration & Mining Geology, CIM v.10, No. 3, pp. 191-213.
|
Yates K R, Robinson P 1990 - Nobles Nob Gold deposit: in Hughes F E (Ed.), 1990 Geology of the Mineral Deposits of Australia & Papua New Guinea The AusIMM, Melbourne Mono 14, v1 pp 861-865
|
Porter GeoConsultancy Pty Ltd (PorterGeo) provides access to this database at no charge. It is largely based on scientific papers and reports in the public domain, and was current when the sources consulted were published. While PorterGeo endeavour to ensure the information was accurate at the time of compilation and subsequent updating, PorterGeo, its employees and servants: i). do not warrant, or make any representation regarding the use, or results of the use of the information contained herein as to its correctness, accuracy, currency, or otherwise; and ii). expressly disclaim all liability or responsibility to any person using the information or conclusions contained herein.
|
Top | Search Again | PGC Home | Terms & Conditions
|
|