Andean Cu-Au-base metals province - Peruvian Andes |
|
Peru |
Main commodities:
Cu Au Mo Ag Zn Pb
|
|
 |
|
 |
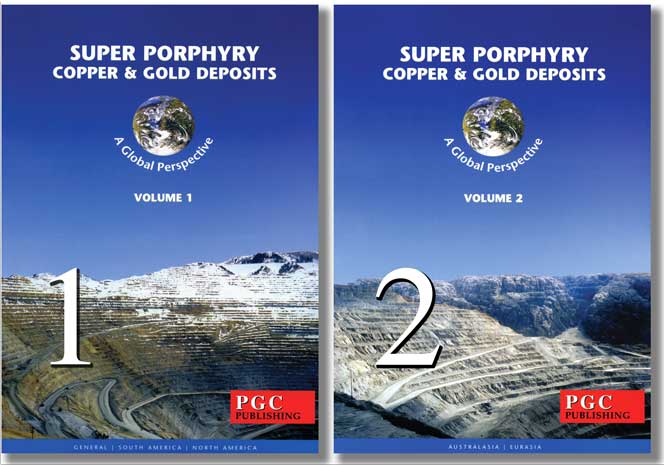 |
Super Porphyry Cu and Au

|
IOCG Deposits - 70 papers
|
All papers now Open Access.
Available as Full Text for direct download or on request. |
|
 |
The Peruvian Andes host a string of porphyry Cu±Mo±Au and Au±Cu, porphyry/skarn Cu±Au±Mo±Zn, epithermal Au±Ag, and Cordilleran epithermal polymetallic replacement and vein deposits over an ~1000 km long, NNW-SSE trending segment of the Andean Orogen.
This segment extends from the 'Huancabamba Deflection' in far northern Peru, marking the southern limit of the Northern Andes, to the 'Abancay (or Pisco) Deflection' in the southern part of the country. For details of the changes and geology to the south of this deflection see the Central Andes and Bolivian Orocline record.
These two 'deflections' correspond to major zones of east-west to ENE-WSW shearing that extend westward from the Archaean to Proterozoic Amazonian craton. In that craton, these structures controlled or parallel the margins of the Neoproterozoic to Recent Solimoes/Amazon rift basin that divides the craton into the northern Guiana and southern Central Brazil (or Guaporé) shields.
The deposits within the Peruvian Andes are almost exclusively associated with Miocene aged intrusions, and due to the dominant Mesozoic carbonate sequences intruded by the magmatic arc, many are characterised by skarn alteration.
The Huancabamba Deflection marks an abrupt change in the trend of the Andean orogen, from NNE-SSW in the Northern Andes, to NNW-SSE in Peru. It is interpreted (e.g., Klein et al., 2011) to coincide with the east-west, sinistral Amazon Megashear that extends eastward into the Amazonian Craton. An accompanying sub-parallel, generally east-west, sinistral structure the Tumbes-Guyana Megashear, occurs ~200 km to the north. To the east, these two structures coincide with the northern margin of the Solimoes Basin, the broader western extension of the Neoproterozoic to Recent Amazon rift basin. To the west they appear to be truncated by the major NNE-SSW Cauca-Pujilí and Romeral-Peltetec-Huancabamba fault complexes that define the tectonic framework, and separate the oceanic and cratonic domains, of the Northern Andes and the adjoining segment in Ecuador. However, over the interval of the intersection of these structures, the Cauca-Pujilí and Romeral-Peltetec-Huancabamba fault complexes, are disrupted by cross features and deflected (e.g., the Dolores-Guayaquil Megashear), and host a much greater density of porphyry and epithermal deposits in southern Ecuador. Both of these generally east-west structures are of Precambrian age and experienced multiple reactivation events since then, and represent major zones of crustal weakness (Jacques, 2003).
The Abancay (or Pisco) Deflection is a ENE-WSW continental trench-normal structure that marks the northern limit of the central volcanic zone of southern Peru, and offsets/deflects the Cordillera Oriental by ~150 km and coincides with the northern limit of the Precambrian Arequipa basement block along the Pacific coast in the SW. It also marks a change in structural trend, from NNW to the north, to NW-SE in southern Peru as well as coinciding with the southern limit of current flat slab subduction. Projected to the east, it follows the southern margin of the Solimoes Basin.
The Peruvian Andes are composed of two parallel ranges the Cordillera Occidental (Western cordillera), corresponding to the Cainozoic magmatic arc, and the Cordillera Oriental (Eastern cordillera), a belt of post-Permian uplift (or lesser subsidence) that exhumed Precambrian crystalline and Palaeozoic sedimentary and igneous rocks. The two ranges are separated by an upland of relatively subdued relief, and narrow intermontaine depressions, which during the Cainozoic have been the site of continental deposition.
Between the Pacific flank of the western magmatic arc and the oceanic trench, there is a system of fore-arc, partly submerged basins along the narrow continental shelf and coastal plain (Benavides-Cáceres, 1999).
The western flank of the Cordillera Occidental is occupied by the Cretaceous to Paleocene Coastal batholith, carbonate-rich Mesozoic country rocks, and the overlying Cainozoic volcanic arc and other localised plutons (e.g., the Cordillera Blanca Batholith). The Incaic Fold and Thrust belt is located to the east of the Coastal batholith, extending to the Cordillera Oriental, and is the locus of several compressive pulses, the more significant of which were in the late Paleocene (59 to 55 Ma); middle Eocene (43 to 42 Ma); Oligocene (30 to 27 Ma); Earliest Miocene (~22 Ma); Mid Miocene (~17 to 15 Ma); and several others in the late Miocene (Benavides-Cáceres, 1999).
The sub-Andean Fold and Thrust belt, which was formed in the late Miocene, is located to the east of the Cordillera Oriental horst, overlapping the western margin of the Eastern Foreland Basin, which hosts an eastward tapering sedimentary section that laps onto the Amazonian craton to the NE and SE, and interfingers with the Solimoes Basin sequence to the east (Benavides-Cáceres, 1999).
Tectonics and Geology
The development of the Peruvian Andes took place as three major cycles, during the i). Precambrian, ii). Palaeozoic to Early Triassic and iii). from the Late Triassic to present. The geologic and tectonic history of the orogen may be summarised as follows:
• Proterozoic and Palaeozoic - While Palaeo- to Mesoproterozoic crust was progressively accreted to the Archaean core of the Amazonia Craton, the Precambrian cycle to the immediate west of the Andes commenced with the amalgamation of the Rodinia supercontinent between 1200 and 1000 Ma during the late Mesoproterozoic. This is reflected in the Sunsás Belt on the margin of the Amazonian craton in Bolivia, eastern Peru (largely under cover) and western Brazil, where a suite of passive margin quartzitic and conglomeratic units, deposited at ~1200 Ma, were intruded by 1105±21 Ma tonalite (U-Pb zircon; Matos in Teixeira et al., 2010), a 1014±6 Ma orthogneiss (U-Pb zircon; Isla-Moreno, 2009) and 1092 to 1047 Ma plutons (Vargas-Mattos et al, 2009), and deformed and subjected to low- to medium-grade metamorphism during the 1120 to 1000 Ma Sunsás orogenesis. Ramos (2010) and other authors present evidence that as part of the amalgamation of Rodinia, the eastern North American side of Laurentia collided with what is now the west coast of South America. Rocks of the Sunsás orogen are exposed in Paraguay and eastern Bolivia and along the Bolivia-Brazil border, and have been proposed to extend further to the NW (e.g., Ramos, 2008; Chew et al., 2011), but it is uncertain if they have been encountered much to the NW of the Abancay Deflection.
The early stages of break-up of Rodinia produced an extensional regime, referred to as the Pampean Tectonic Cycle, resulting in the development of a broad shallow basin filled with intracratonic platformal clastic sedimentary rocks. Subsequent to the Rodinia break-up and the opening of the intervening Iapetus ocean (Dalziel, 1997), some terranes remained attached to South America/Gondwana, while others were rifted away with Laurentian. Others that were detached during the breakup, were returned and amalgamated to Gondwana in the Early Cambrian to Middle Ordovician as part of the Terra Australis Orogen (Ramos, 2010; Cawood, 2005).
The Terra Australis Orogen developed along the palaeo-Pacific/Iapetas margin of Gondwana, and extended over a continuous pre-dispersal length of ~18 000 km and up to 1600 km width, from eastern Australia and New Zealand, the periphery of Antarctica, and along the entire western fringe of what is now the South American plate. The Pacific/Iapetas margin was the result of rifting associated with the Neoproterozoic break-up of Rodinia. Despite a long history of plate convergence, reorganisation and terrane accretion, the Pacific ocean has never subsequently closed, and the Terra Australia Orogen has been both a passive and active margin at different times and localities since the Lower Palaeozoic (Cawood, 2005).
The oldest exposed rocks within the Peruvian Andes are confined to the Cordillera Oriental (Eastern Cordillera) where they have been exposed by uplift of the Marañón Arch/horst over a width of up to 75 km and much of the length of the Peruvian Andes. These are part of the Marañón Complex that originally formed as the Marañón Magmatic Arc during the late Cambrian to Devonian compressive Famatinian Tectonic Cycle, and includes metasedimentary schists and gneisses that have been intruded by Ordovician to Silurian, and subsequent Carboniferous and Permo-Triassic plutons. Chew et al. (2007) demonstrated that the Marañón Complex has been affected by two major orogenic events. The post-tectonic Pacococha adamellite constrains all polyphase deformation within the Complex to be prior to 474.2±3.4 Ma, whilst 25 km to the SE, the migmatitic Huacapistana granite and granite migmatic paragneisses of the Marañón Complex, both of which are syn-D2, yielded ages of 310.1±2.3 Ma and 312.9±3.0 Ma, respectively (Chew et al., 2007). The latter ~312 Ma tectonothermal event is confirmed by the deformed 325.43±0.57 Ma Hualluniyocc adamellite and the undeformed 307.05±0.65 Ma Utcuyacu granite plutons. Detrital zircons from meta-sandstone nearby that had been affected by the ~312 Ma event were dated at 474 Ma, suggesting deposition following the Ordovician event, whilst some of the rocks affected by the 474 Ma event, do not contain detrital zircons that are <750 Ma.
A third, loosely constrained event in indicated by i). the Sitabamba orthogneiss, crystallised between 446 and 442 Ma, but overprinted by high-grade metamorphic assemblages; and ii). a strongly deformed psammite which contains an abundant 470 Ma detrital zircon population, but is overlain by Lower Carboniferous sedimentary rocks. These imply a Siluro-Devonian deformation age of between 440 and 340 Ma (Chew et al., 2007).
A study of detrital zircons (Chew et al., 2007) from the sequence, indicates large populations in the ranges, in decreasing order of abundance, 0.45 to 0.5; 0.9 to 1.3; 0.7 to 0.9; and 0.5 to 0.65 Ga. This is taken to indicate both the older and younger unit sedimentary protoliths were both derived (directly or indirectly) from late Cambrian to Devonian Famatinian and late Mesoproterozoic Sunsás belt rocks to the east, on the margin of the Amazonian craton (Chew et al., 2007; Ramos, 2010).
These data collectively imply the Marañón Complex includes the following (Chew et al., 2007, 2010; Ramos, 2010):
- an older, at least partly Proterozoic suite of metasedimentary schists and gneisses that underwent metamorphism prior to 474 Ma,
- a Famatinian Ordovician magmatic and metamorphic event at 480 to 470 Ma,
- a younger, Palaeozoic succession that was derived in part from the ~480 to 470 Ma magmatic belt, and includes Devonian quartzites,
- a Siluro-Devonian deformation event between 440 and 340 Ma,
- a magmatic and metamorphic event at 325 to 302 Ma in the upper Carboniferous.
The relationship between these sequences is uncertain, due to later Mesozoic and Tertiary thrust stacking.
The geological and geochronological character of the Marañón Complex are very similar to those of a belt of rocks that extend along the southwestern coastal margin of the 1198 to 970 Ma Proterozoic gneisses of the Arequipa Terrane in coastal southern Peru, south of the Abancay Deflection (Chew et al., 2007). This led Chew et al. (2007) to suggest an Early Ordovician magmatic and metamorphic belt that runs along the western margin of the Arequipa basement and is offset northeastward into the Eastern Cordillera. They further suggest this offset followed an original embayment on the western Gondwanan margin during the early Palaeozoic, and that this embayment was then filled by subsequent accretion of oceanic material that became the basement to the Western Cordillera (e.g., Polliand et al., 2005). However, Ramos (2008) suggests this arc of the Marañón Complex is subduction related, caused by 'continent-continent' collision and that the continent that collided with the Arequipa Terrane would have been ~675 km from the Marañón Complex (allowing for subsequent shortening), too far to have caused the degree of deformation and metamorphism. Ramos (2008) suggests that the Paracas terrane, an established sialic basement immediately offshore of the Peruvian Andes, north of the Arequipa Terrane (seen in offshore petroleum drilling and in geophysical data; e.g., Thornburg and Kulm 1981), collided with the western margin of Gondwana within this embayment to form the Marañón orogen during the Famatinian cycle. Subsequent rifting of the intervening Huarmey basin during the Cretaceous, when it was filled with >9 km of mafic pillow lavas and pyroclastic rocks, has separated the bulk of the Paracas terrane from the Marañón Arch, with remnants possibly below the Cordillera Occidental (Ramos, 2008).
To the east of the metamorphic suite of the Marañón Complex, a back-arc extensional setting is suggested by the marine shales and sandstones of the Ordovician Contaya Formation (Jaillard et al., 2002). The strongly metamorphosed Marañón Complex, which was uplifted during Palaeozoic to Tertiary extension to form the core of the Marañón Arch (see below), is structurally bounded in south-central Peru by a less metamorphosed sequence. This latter sequence comprises 'thousands of metres' of generally unfossiliferous, dark grey or black shales and quartzites, mapped as the Excelsior and Cabanillas Groups. This succession is known to include middle Ordovician and lower to middle Devonian rocks, and disconformably underlies Lower Carboniferous rocks. The Excelsior Group is usually regarded as being largely of Devonian age (e.g., Newell et al., 1953).
• Permo-Carboniferous and Lower Triassic - The Peruvian Andes are underlain by a dissected and deformed platformal sequence of Permo-Carboniferous and Lower Triassic rocks, lapping onto the Amazonian craton to the east, and unconformably overlying the lower to middle Palaeozoic basement. This succession belongs to the Mid-Devonian to Late Permian/early Triassic, predominantly extensional Gondwana Tectonic Cycle, and commenced with the Carboniferous Tarma-Copacabana sequence, largely composed of sandstones, mudstones and limestones, deposited in a mosaic of intense intracontinental rift basins and horsts controlled and linked by strike-slip fault zones that have 'Andean trends' (Rosas et al., 2007).
According to Rosas et al. (2007), Permo-Carboniferous fault-controlled subsidence diminished and ceased by the Late Permian, followed by widespread regional subsidence and a broad epeiric sea into which the argillaceous, organic-rich Ene Formation was deposited as a regional blanket.
This was followed by a hiatus related to the late Permian to early Triassic Juruá orogeny, which was accompanied by synkinematic calc-alkaline granodiorites and granites, and alkaline to peralkaline comendites, basalts and syenites in the Cordillera Oriental, with an age range of 255 to 236 Ma (Rb/Sr, K/Ar; Lancelot et al., 1978; Dalmayrac et al., 1980; Gunnesch et al., 1990; Soler, 1991).
In other references (e.g., Newell et al., 1953; INGEMMET, 2013) the upper Palaeozoic succession is divided into the Lower Carboniferous Ambo Group, which disconformably overlies the Excelsior Group, and is dominantly composed of sandstone with thin black shales and coal, and exceeds 850 m in thickness. In far northwestern Peru, in the Amotape Mountains sequence that continues across the border into Ecuador, the equivalent succession is the Chaleco de Paño Formation, composed of 1200 m of greenish-grey quartzites, sandstones, siltstones and slates (Martinez, 1970). These sequences are respectively disconformably overlain by the Upper Carboniferous Tarma Group thin limestones and black shale that is up to 2100 m thick in central Peru, and the coarser Cerro Prieto Formation in NW Peru, comprising >1600 m of interbedded dark grey, partly marly shale, siltstone, crossbedded and graded sandstones, quartzites and rare limestones (Martinez, 1970). These sequences are, in turn, disconformably to discordantly overlain by the ~1900 m thick Lower Permian Copacabana Group massive limestone and black shales in central Peru. These rocks are discordantly followed by the Permo-Triassic Mitu Group as described below, which is variously regarded as Late Permian (e.g., INGEMMET, 2013) or Lower Triassic (e.g., Rosas et al., 2007).
• Middle Triassic to Earliest Cretaceous - Following and during the Juruá orogeny, renewed deposition commenced as a prelude to the main extensional regime, resulting in the latest Permian to middle Triassic Mitu Group, which was not involved in the Juruá orogeny, and unconformably overlies deformed Ene Formation (Jaillard et al., 1990; Rosas et al., 2007). It was deposited in a complex of rift basins, the most significant of which was the Mitu graben, largely coinciding with the core of the current Cordillera Oriental. This main graben was separated from a secondary belt of grabens largely along the present coastal plain and narrow continental shelf by the major Divisoria horst. The Mitu Group comprises as much as 3000 m of continental clastic molassic sediments/red beds, volcaniclastic rocks and alkaline volcanic rocks. Master faults along both the basin axis and margins, were the pathways for mantle-derived continental Mitu volcanism, mainly tholeiitic or alkaline basalts accounting for up to 20% of the sequence, that were accompanied and followed by the intrusion of a voluminous belt of crustally derived Permo-Triassic granodioritic to monzogranitic plutons, largely along the present Eastern Cordillera (Rosas et al., 2007; Panca, 2009).
During the Early Jurassic, Mariana-style subduction of the Farallon plate is interpreted to have commenced (Benavides-Caceres, 1999), well to the west of the continent, producing intra-oceanic island-arc magmatism in the west and back-arc extension on the continental margin (Longo, 2005 and references cited therein). Initiation of this subduction marked the commencement of the Andean Tectonic Cycle in Peru (Cobbing et al., 1981; Megard, 1984), which has persisted for ~200 m.y. (Petford and Atherton, 1995), punctuated by the split of the Farallon plate into the Nazca and Cocos plates in the late Oligocene to early Miocene. During much of the Mesozoic, the broad back-arc region between the inferred offshore subduction system and the Amazonian craton, was dominated by extensional tectonics that resulted in belts of differential subsidence and uplift bounded by major longitudinal crustal faults, for the most part inherited from the Palaeozoic. In general, the Mesozoic marine sequences deposited during this period included rift and shelf sequences, with subduction-related volcanic facies along their western extremeties (Benavides-Caceres, 1999). Crustal thickening in the overriding plate only started in the Upper Cretaceous, when the magmatic arc migrated onshore, whereas Jurassic and Lower Cretaceous times have essentially been marked by back-arc extension (e.g., Mpodozis and Ramos, 1989; Jaillard and Soler, 1996).
The Mitu Group sequence is overlain across a contact that varies from a paraconformity to angular unconformity, by the three part sag phase successions of the 1000 km long by >250 km wide, late Triassic and early Jurassic carbonate rich Pucará Basin (Rosas et al., 2004). This basin was related to transtensional subsidence, and comprised: a). the Norian to Rhaetian Chambará Formation, dolomite and subordinate limestone; b). the upper Rhaetian to Sinemurian Aramachay Formation, bituminous calcareous shales, indicating a deepening underfilled basin; and c). the upper Sinemurian to Toarcian Condorsinga Formation that caps the succession, and is again dominated by shallower-water limestones, which are locally sandy, with evaporites. This sequence contains intercalated intraplate basaltic to andesitic volcanic rocks (Rosas et al., 2004). This NNW-SSE basin lapped onto the Amazon Craton, or interfingered with the Solimoes basin to the east, and is divided into four main geotectonic zones:
i). the Eastern Foreland Basin, which areally corresponds to the current sub-Andean fold belt and eastern or Ene-Madre de Dios foreland basin, and was the site of epicontinental evaporite and pelitic carbonate deposition;
ii). the Marañón Arch, a basement horst, or belt of lesser subsidence, generally corresponding to the present Cordillera Oriental, cored by Proterozoic to Palaeozoic basement, as described above. This arch began to form as a horst within the main Mitu graben and provided a base for the subsequent shallow water carbonate platform of the Pucará Group;
iii). the Western Platform, mostly covered by shallow-water marine, carbonate shelf sedimentary rocks of the Pucará Group. The Pucará transgression, which had commenced in northern Peru by the Late Triassic (Middle Norian), had reached central Peru in the Late Norian. Continued extensional conditions are also indicated by the local eruption of within-plate volcanic rocks, particularly near the western boundary of the platform (Rosas, 1994; Benavides-Caceres, 1999);
iv). the Divisoria Arch/horst of coastal Peru, also a belt of lesser subsidence or emergence which forms the western margin of the Western platform (and Pucará Basin), and was inherited from Permian time. It exposes a lower to middle Palaeozoic sequence, with Mesozoic to Cenozoic intrusions, and is separated from the Western platform by a major NW to NNW sinistral shear zone that passes just to the east of Cajamarca and Yanacocha. To the north, as it approaches the Huancabamba deflection, it diminishes, and the Western platform sequence grades directly into the Western volcanic belt (Rosas et al., 2004; Benavides-Caceres, 1999; Jaillard et al., 1990; Longo et al., 2010).
v). the Western Volcanic Belt - characterised by mid to late Jurassic volcanism in northern coastal Peru, where 1000 to 3000 m of subaerial basaltic andesitic to dacitic lava flows and pyroclastic beds, the Colán Formation, were deposited to the west of the Marañón arch and north of the latitude through Cajamarca (Rosas et al., 2004; Jaillard et al., 1990; Longo et al., 2010). This volcanic sequence is interpreted to represent the southern continuation of the Jurassic magmatic suite of the Cordillera Real and Condor/Sub-Andean Cordillera to the north in Ecuador, which includes the Misahualli Volcanic Complex and the composite Zamora batholith. However, there are no significant intrusives associated with the Colán Formation in Peru. The structural grain of the Colán Formation does reflect the trend of the Peruvian Andes south of the Huancabamba Deflection, occurring as a series of en echelon, NNW elongated exposures, distributed along the same projected SSW trend as in Ecuador, continuing to the coastline, and not reappearing again north of the Abancay Deflection in the south (Romeuf et al., 1995).
The early Middle Jurassic Vicusian orogeny, which affected both Ecuador and Peru, resulted in the sub-Andean fault system being particularly active, with the Marañón arch on its immediate western margin experiencing considerable uplift and erosion. This event also caused a regression, interrupting the carbonate sedimentation of the Pucará Group with a major unconformity and stratigraphic break.
As a consequence of this regression, and the extensional tectonics that characterised the Late Jurassic, the Eastern basin, the Marañón arch and the Huancabamba deflection region were emergent, and with inversion of the Divisoria arch, subsidence was limited to the Western Platform. Deposition on the platform passed from deltaic to paralic siliciclastic sedimentary rocks along the Western platform (Yura and Oyón Groups), passing westward through a slope facies into deeper water facies of the 'Western Trough', to subduction related volcanic rocks along the marginal volcanic arc.
Following the Vicusian orogeny, a new northward and eastward progressing shallow transgression commenced in the late Middle Jurassic (Callovian) in western Peru, reaching northern Peru in the Late Jurassic to Lower Cretaceous as the Chicama Group, and is characterised by predominantly siliciclastic rocks, which occur further east as the Sarayaquillo Formation, particularly in the Eastern foreland basin where red-bed clastics dominate. During this transgression, subduction-related volcanism continued along the marginal volcanic arc, with intense crustal attenuation in the western trough.
The Vicusian orogeny had overlapped the deposition of the Colán Formation volcanic sequence which rests unconformably on the Pucará carbonates of the Western platform (Loughman and Hallam, 1982) and is covered by the siliciclastic of the Sarayaquillo Formation. Subsequent to this orogenic event, the Colán Formation volcanic island arc in northern Peru and its continuation in Ecuador became a continental magmatic arc, accompanied by major batholithic intrusions in the inner part of the orogen in Ecuador, e.g., the Zamora Batholith (Benavides-Caceres, 1999).
The Late Jurassic transgression, was followed by emergence in the earliest Cretaceous (Berriasian) of almost all of Peru, which produced the Great Pre-Cretaceous unconformity (Benavides-Caceres, 1999).
• Lower to Middle Cretaceous - Following the pre-Cretaceous unconformity, a Lower Cretaceous subduction-related successor volcanic arc along the western margin of the continent, extended beyond the present coastline, and was responsible for the late Berriasian to Valanginian Puente de Piedra volcanic sequence, comprising basalt and basaltic andesite, with anomalous arc and within-plate geochemical characteristics (Atherton et al., 1985).
In the Valanginian, a transgressive event progressed from the western margin of the continent, into the Eastern foreland basin, and produced a major sequence of eastern-derived, platformal siliciclastic sediments of the Chimú Group and related units. These sequences were mainly quartzose sandstone with minor intercalations of plant-bearing carbonaceous shale and coal beds, and interfingered with the Puente de Piedra volcanic sequence to the west. Locally in the north of Peru, these were followed by a thin carbonate unit, the Santa Formation, the top of which is marked by a disconformity, reflecting a minor compressive pulse (Benavides-Caceres, 1999).
During the mid-Lower Cretaceous (Hauterivian), post Chimú Group, a major extensional event across the region led to crustal fracturing and formation of a rift trough, the Western Marginal, or Huarmey-Cañete trough, along the present coastal region, immediately to the west of the Western platform, separating the platform from the NNW trending sialic Paracas arch in the west. This extension corresponded to crustal attenuation, a concurrent mantle surge, high heat flow, and the deposition of a >9000 m thick volcanic pile within the Huarmey-Cañete trough. In the axial sections of the trough, the Aptian to Middle Albian volcanic sequence is predominantly of tholeiitic basalt and basaltic andesite, ranging from lower calc-alkalic arc-type to upper, low-K, MORB-like, tholeiitic compositions (Atherton and Webb, 1989). Volcanism on the eastern margin is high-K, with more silicic volcanic rocks, has more pyroclastic rocks, and includes shallow marine sedimentary intercalations. Deposition marginal to the trough was initially deltaic and shallow marine, grading upward into pelitic, turbiditic and volcaniclastic sequences (Benavides-Caceres, 1999).
The interpreted northern extension of this trough was developed in far northwestern Peru and southern Ecuador, in the following tectonic context. In southern Ecuador, the main NNE structural trend/corridor that separates continental and oceanic terranes in northern-central Ecuador, is disrupted by a set of major splays that swing SW into the Gulf of Guayaquil. These include the Dolores-Guyaquil Megashear, and a little to the south, the major Bulabula fault. The latter appears to define the southern limit of the oceanic Piñón Terrane that occupies the western margin of the continent in Ecuador and Colombia. The main NNE trending regional structural corridor is in part cut to the south of the Gulf of Guayaquil by a WNW-ESE arc-transverse structure, the Piñas-Portovelo fault zone. To the east, however, the main NNE-SSW structural corridor continues south into Peru, swinging to a NNW-SSE trend across the Huancabamba Deflection zone, an east-west continental scale basement feature. This longitudinal structural zone exposes (Proterozoic-)Palaeozoic and Mesozoic metamorphic rocks of the NNE-SSW to north south Olmos arch. The transverse Piñas-Portovelo fault, defines the northern margin of the WNW-ESE trending Amotape range (or Amotape arch). To the west, this latter arch swings to the SW and exposes similar metamorphic rocks to those of the Olmos arch, which include blueschist facies rocks and an associated eclogitic harzburgite complex, indicating the fault represents a suture zone of possible Jurassic to Cretaceous age.
A Cretaceous back-arc basin, the Lancones trough, is developed to the south and SE of the Amotape arch, and west of the Olmos arch in far southern Ecuador and northwestern Peru. This back-arc basin contains the thick Lancones volcanic complex, characterised by an ~10 km thick, mantle derived sequence of basaltic lavas and pyroclastic rocks, including some rhyolites, overlain by a Cretaceous turbiditic succession, mainly shales, greywackes and pyroclastic rocks with conglomerates and sandstone facies of the Upper Middle Albian to Coniacian Copa Sombrero Group (Alamor Group in Ecuador). This trough appears to represent the northern, fault offset continuation of the Western Marginal/Huarmey-Cañete trough (Benavides-Caceres, 1999). It apparently predates accretion of the Piñón terrane to the north, and may also be equivalent to the back-arc basin of the Romeral terrane in Colombia.
Towards the end or the Lower Cretaceous, fractures related to the Western Marginal/Huarmey-Cañete trough, channelled the magmas that produced the Mid Cretaceous (Albian) to Paleocene Coastal Batholith (Benavides-Caceres, 1999).
East of the Huarmey-Cañete trough, the Hauterivian to Middle Albian (mid to late Lower Cretaceous) sedimentary wedge has a conspicuous basal quartzose unit, the Aptian to Lower Albian Goyllarisquisga Formation in the Western platform, and the Cushabatay Formation in the Eastern basin (Benavides-Caceres, 1999).
In northern Peru, the stratigraphy on the western platform has more recently been reorganised (e.g., Navarro et. al., 2010) into the early-Lower to mid-Lower Cretaceous Goyllarisquisga Group that rests on the Late Jurassic to early Cretaceous Chicama Group, and comprises, from the base, the Oyón Formation siliciclastics; Chimú Formation quartzite, with shale interbeds; Santa Formation dolomitic limestone, marly limestones and shale; Carhuaz Formation sandstones; and Farrat Formation medium to coarse grained, crossbedded quartzite and sandstone with intercalated shale and marl.
In northern and central Peru, the Goyllarisquisga Formation/Group was transgressively overlain by a marl and carbonate sequence of the Machay
Group. The Machay Group contains two transgressive sequences separated by a disconformity ascribed to late-middle Albian
uplift and erosion related to the Mochica orogeny (Mégard, 1984). This sequence began with the Albian shallow-water, carbonate rich Inca, Pariahuanca and Chulec Formations, and culminated with the deeper-water, anoxic unit, the Middle Albian Pariatambo Formation. These units represent the lower part of the Machay Group.
The Marañón arch is overlain by the Lower to Middle Albian marly Crisnejas Formation, whilst the same interval is represented by the shaly Esperanza Formation in the Eastern basin (Benavides-Caceres, 1999).
This period of extension was terminated by the two episodes of the (late Middle Albian) compressive Mochica orogeny at 100 and 97 Ma (Myers, 1974; Cobbing et aI., 1981; Megard, 1984) which particular affected the Huarmey-Cañete trough in central and northern Peru and was accompanied by the the emplacement of the early stage tholeiitic gabbros and syn-plutonic dykes of the Coastal batholith. This orogenic event resulted in uplift and erosion and a widespread unconformity, which in the Western platform, marked the top of the Pariatambo Formation and equivalents.
Above this unconformity, a new sedimentary cycle commenced, which essentially followed the same tectonic pattern of extension and subsidence as the preceding cycle, except there was much less subsidence along the Huarmey-Cañete trough, where emplacement of the voluminous Coastal batholith was ongoing, and shallow marine and subaerial volcanic and volcaniclastic deposition took place (Benavides-Caceres, 1999).
On the Western platform, the Arcurquina carbonate bank of southern Peru prograded northward from the Late Albian to the Turonian, to form the upper section of the Machay Group comprising the up to 1500 m thick Jumasha carbonate bank of the Jumasha Formation overlying the Albian Inca, Pariahuanca and Chulec formations. In northern Peru, the shallow-water carbonates of these formations pass northward into a carbonate and marl sequence, represented by the Yumagual, Pulluicana, Quillquinan and Cajamarca formations, more or less following the current continental divide of the Cordillera Occidental. At the same time, the Eastern basin was occupied by the prograde quartzose Agua Caliente Formation and two late cycles of pelitic and quartzitic sedimentary rocks. By the early Campanian, deposition had changed from sediment derived from the east by the proto-Amazon river, to continental red-bed molasse sourced from the rising Andes (Benavides-Caceres, 1999).
In the Upper Cretaceous (Coniacian), a short-lived marine transgression deposited marls and limestones of the Celendín Formation onto the Western platform and drowned the regional Cajamarca-Jumasha-Arcurquina carbonate bank (Benavides-Caceres, 1999). This represents the uppermost unit of the Machay Group.
This period of extension was terminated by the early to middle Campanian Peruvian orogeny, during the mid-Upper Cretaceous.
• Mid-Upper Cretaceous to Paleocene - The Peruvian orogeny from 84 to 79 Ma, marked a major change from intra-oceanic Mariana-type subduction, with its associated extensional regime that had persisted from the Late Triassic, to the Andean-type subduction that prevailed to the present, characterized by recurrent compressive pulses and intense magmatic episodes. A continental magmatic arc, located between the Coastal batholith and the Marañón arch succeeded the Coastal batholith, along the coastal western margin of the Peruvian Andes as a result of subduction by the oceanic Farallon, and from the late Miocene, the Nazca plate. This change was resulted in i). withdrawal of the seas from the Cordilleran region; ii). a series of compressive pulses forming three major discrete fold and thrust belts, the Peruvian, Incaic and Quechua; iii). development of fore-arc, intermontane and extensional foreland basins; iv). crustal shortening, thickening and uplift of the Andean Range; v). development of several significant regional erosion surfaces indicative of periods of relative stability punctuating the compressive pulses.
Tectonism related to the Peruvian orogeny deformed the Western platform and Huarmey-Cañete trough in northern Peru, with NE vergent isoclinal and recumbent folds and thrusts of variable scales, that are inclined to the NE or east, as well as a complex system of flats, ramps and duplexes, and more than 40% shortening, which, in this area, could represent 25 to 30 km (Benavides-Caceres, 1999).
During this period, emplacement of the 1600 km long by ~60 km wide composite Coastal batholith had continued within the Huarmey-Cañete trough in a series of pulses from ~100 Ma, when magmatism switched from the early tholeiitic gabbroid plutonism to the emplacement of the large, calc-alkaline dioritic and tonalitic plutons. This batholith comprises a wide array of granitoid intrusions in the form of plutons, dykes, and sills, channeled through a deep crustal lineament and assembled in a distinctly episodic fashion. Individual pulses continued to after 50 Ma, with lulls from 101 to 95, 90 to 86, 76 to 73 and 57 to 50 Ma. Subsequently, magmatic activity was much more restricted and tended to be limited to a well-marked chain of stocks along the eastern margin of the batholith. This batholith extends from the Peruvian Andes, across the Abancay Deflection into the Central Andes of southern Peru (Benavides-Caceres, 1999).
From the end of the 84 to 79 Ma Peruvian orogenic pulse, until the 59 to 55 Ma Incaic I compressive event, during the latest Cretaceous and Paleocene, the tectonic framework of the Peruvian Andean region, from east to west was (after Benavides-Caceres, 1999):
i). the Eastern foreland basin, which laps onto the Amazonian craton and interfingers with the lapped onto the Solimoes/Amazon basin to the east, and is occupied by a major sequence of dominantly non-marine red beds derived from the rising Andes to the west. From the Middle Cretaceous, the Eastern basin, east of the sub-Andean fault system, evolved into an asymmetric, increasingly subsident, foreland basin largely filled by non-marine, upward-coarsening molasse deposits derived from the exposed Andean mass. A major marine regression commenced in the Campanian and was completed by the Maestrichtian. From the latest Campanian to the Paleocene, foreland subsidence was relatively slow and the basin fill consisted largely of red and brown pelitic marls and clays;
ii). the Marañón arch, which was strongly uplifted, but although emergent, had only low relief;
iii). a belt of narrow intermontane basins and troughs where the non-marine Casapalca Formation red beds and their equivalents were deposited, largely between the gently raised western edge of the continent and its magmatic arc, and the Marañón arc. The thicknesses of these red beds are ~2000 to 4000 m in central Peru, to ~1000 m in northern Peru, and are capped by a major unconformity in both areas. In places, the Casapalca red beds include up to 1200 m of pyroclastic flows, lava and volcanoclastic deposits of the Paleocene Tablachaca Formation (e.g., Rivera et al., 2005);
iv). The Coastal magmatic arc, which in the Peruvian Andes is characterised by the Coastal Batholith, South of the Abancay Deflection, this zone includes the 75 to 59 Ma Toquepala volcanic rocks. These volcanics have not been mapped to the north of the Abancay Deflection, although the presence of the Tablachaca Formation volcanic and volcaniclastic elements within the coeval Casapalca Formation red-beds suggests a volcanic arc may have persisted further to the north along the continental margin;
v). Fore-arc basins, which are preserved as grabens west of the Amotape arch in northwestern Peru, and presumably also west of the largely submerged Paracas arch southward on the continental shelf. These grabens contain a sedimentary sequence which include shallow marine sands, shales and breccias, deposited in subsiding grabens adjacent to actively rising horsts;
vi). the Pacific realm.
In northern Peru, there was little deposition during the period from the late Cretaceous (Campanian) to Paleocene, other than the Casapalca Formation straddling the Cretaceous-Cainozoic boundary.
• Incaic I compressive event between 59 and 55 Ma, which terminated the Cretaceous to Paleocene deposition throughout the Peruvian Andes, and produced a major hiatus and unconformity. It also formed the Incaic Fold and Thrust belt, that extended from the Coastal block, including the rigid Coastal batholith in the west, to the Marañón arch in the east. This represents an eastward migration of deformation from the previous Peruvian Fold and Thrust belt. Erosion related to this orogenic pulse to the north of Cajamarca bevelled rocks from the Proterozoic to the Coastal batholith, and removed as much as 3 to 4 km of roof rocks from the latter, to form the Caldera erosion surface. Between the Abancay deflection and just south of Cajamarca, the Incaic Fold and Thrust belt is divided into two by the longitudinal Incaic megafault, which is marked by the Cordillera Blanca fault system, interpreted to represent a steep, deep-seated reverse fault. To the west of this structure, whilst compression was active, it did not result in significant deformation. In contrast, immediately to the east of the megafault, the rocks were not only strongly folded but also developed a fracture schistosity, with almost vertical fractures that are cut by thrust faulting. These differences are reflected by the nature of the unconformity, which cuts gently folded Albian sedimentary rocks and the Coastal batholith to the west, and strongly folded Jurassic and Cretaceous formations to the east (Benavides-Caceres, 1999).
• Early to Middle Eocene, from 55 to 43 Ma, between the Incaic I and II orogenic pulses, marked the deposition of the first members of the Eocene to Miocene (55 to 15 Ma) Llama-Calipuy Volcanic Sequence, which overlie the tightly folded Incaic I orogen with sharp unconformity. The composite Llama-Calipuy package rocks were predominantly erupted from fissures, and are up to 2000 m thick, composed of subaerial, terrestrial, mafic to felsic tuffs, lavas and agglomerates. They include minor sedimentary rocks that are generally coarse red beds and include scarce fresh-water limestone, as well as a prominent, but irregular, basal conglomerate (Benavides-Caceres, 1999). This volcanic sequence is best developed between the Ecuadorian border and Cajamarca, ~250 km to the SSE, but has also been found forming large areas of the crests and high plains of the Cordillera Occidental, generally east of the Coastal batholith, to as far south as the Chancay River, ~100 km north of Lima (Benavides-Caceres, 1999).
The section of this pile deposited between the Incaic I and II orogenic pulses is represented by the 54.8 to 44.2 Ma Llama Volcanics (Wilson, 1984; Reyes and Caldas, 1987; Noble et al., 1990), largely composed of silicic ash-flow tuffs (Longo et al., 2010). This sequence grades eastward into a coeval, red, coarse and reworked sequence of volcaniclastic material that comprise the Chota Formation, deposited within an intermontaine graben. A number of stocks to the east of the Coastal batholith are coeval with these tuffs, including the 55 to 48.8 Ma Trujillo granitoids (K/Ar; Stewart et al., 1974); 49.7 Ma Cerro Aislado pluton, NNE of Casma (U/Pb; Mukasa, 1986); 48.8±0.5 Ma Pariacoto stock, east from Casma (Rb/Sr; Beckinsale et al., 1985); 55.0 Ma Huaytanl granite (K/Ar; Stewart et al., 1974). In northern Peru, coeval stocks include the 43.6 Ma Picota Diorite (Laughlin et al., 1968), and a pre-mineralisation 46.4 Ma intrusion at Michiquillay (Laughlin et al., 1968).
• Incaic II compressive event, from 43 to 42 Ma, which like the Incaic I deformation, was focused on the same Incaic Fold and Thrust belt between the Coastal block and the Marañón arch. It produced the major Incaic II unconformity, the Laderas erosion surface, which is conspicuous throughout the Peruvian Andes. This event is interpreted to be related to a global period of greater tectonic activity, including a strong increase in convergence rate and change of relative plate motion between the Farallon and South American plates by 42 Ma (Pardo Casas and Molnar, 1987).
The composite Incaic Fold and Thrust belt resulted from major compression and shortening imposed during both the Incaic I and II orogenic pulses, and indicates compression in a SW-NE direction. Between the Abancay deflection in the south, and just south of Cajamarca in the north, the eastern margin of the belt is characterised by a narrow imbricate zone of stacked bedding plane overthrusts over a width of ~5 km, thrust directly against the Marañón arch (Wilson et al., 1967). Individual thrusts, are as much as 25 km in length, and generally controlled by ductile Albian and Upper Cretaceous platform carbonates. To the south of Cerro de Pasco, these thrusts have, in turn, been folded with steep axial planes, flattening fabrics, and the development of schistosity (Benavides-Caceres, 1999).
Immediately to the north of Cajamarca, and the northern end of the Incaic megafault, the fold axes curve sharply to an almost east-west trend, forming the Chimu Andes (Steinmann, 1929; Hollister, 1977) or Cajamarca curvature over a length of ~50 km, before resuming a NNW trend. This structural feature is interpreted to have formed during the Incaic II event (Benavides-Caceres, 1999).
Within this section of the Andes, there are also a series of prominent parallel, NE striking faults and fractures, oriented transverse to the trend of the Cordillera. These structures define the Cutervo-Cajamarca structural zone, and include the following major lineaments the Cajamarca (passing through Cajamarca), Sipan-Hualgayoc (~40 km NW of the Cajamarca lineament), La Zanja-Tantahuatay and Cutervo (south of La Granja, and ~120 km NW of the Cajamarca lineament) and a number of others between Cutervo and the Ecuadorian border.
The Cajamarca lineament closely coincides with the sharp northern margin of a 50 km wide gap in the magmatic arc. North of this gap, the arc expands from its normal width of ~50 to 90 km to a maximum in northern Peru of ~200 km. South of the gap, the arc is 30 to 55 km wide, and more deeply eroded, exposing the underlying Coastal Batholith.
In the northern Peruvian Andes, the Incaic Fold and Thrust belt is characterised by generally upright to east-vergent folds that are as much as 100 km in length and 4 km in width. Their morphology and style is influenced by the geology, such as the ductility contrasts and bedding anisotropy within the Mesozoic formations, between rocks such as the highly competent quartzites of the Chimú Formation, and the ductile carbonates of the Jumasha Formation, which deformed by flexural slip. The overall fold geometry is broadly concentric with Chimú quartzites in the anticlines and Jumasha carbonates in the synclines (Benavides-Caceres, 1999).
Major overthrusts near the marginal eastern imbricate belt, some of which are >100 km in length, are generally related to the Chicama-Oyón anticlinoria, represent considerable tectonic transport, juxtaposing deeper facies from the Western trough against the platform facies of the imbricate belt (Benavides-Caceres, 1999).
The major en echelon structure of the belt, the Cajamarca curvature, and the north-south compression in the Cajamarca area, indicate the Coastal block moved toward the NE, over the eastern block, but with a northward, dextral component, possibly due to oblique subduction.
The Incaic Fold and Thrust belt is also characterised by a system of NE-SW (with lesser east-west and NW-SE) trending transverse faults with significant vertical and lateral displacement that extend beyond the fold and thrust belt (Vidal and Noble, 1994).
• Late Eocene to Earliest Miocene - Following the erosional phase represented by the Incaic II unconformity, a new cycle comprising uplift, extensional tectonics, and magmatic activity, extended from ~42 to 23 Ma as the Lower Calipuy Volcanic Sequence (known as the Tacaza Volcanic Sequence in southern Peru). This sequence is at least 1500 m thick, with a maximum outcrop width of ~150 km in southeastern Peru, narrowing abruptly to ~40 km after crossing the Abancay deflection into the Peruvian Andes. From there, it largely follows the Cordillera Occidental and its eastern slopes, practically to the Ecuadorian border. It is predominantly composed of porphyritic andesite, basalt, dacite, rhyodacite and rhyolite flows, agglomerates, tuffs and welded ignimbrites, intercalated with as much as 50% terrestrial volcaniclastic rocks, red and purple conglomerates and sandstones. Different sections of the sequence are dominated by either lavas, pyroclastic or sedimentary rocks. Locally it includes coarse basal conglomerates that were deposited in fault troughs over an irregular surface. In the south, the Tacaza/Calipuy Volcanic Sequence has been divided into the Late Eocene to Earliest Miocene Lower Calipuy Group and the Early Miocene Upper Calipuy Group that followed the Incaic IV orogenic pulse. However, Benavides-Caceres (1999) notes that in 1999, the two had largely not been differentiated in mapping in central and northern Peru. Hence the extent of each group individually is uncertain.
In the Cajamarca Mineral Belt, which extends from just to the south of Cajamarca in northern Peru to the Ecuador border, and corresponds to the northern Peruvian volcanic belt, the Lower Calipuy Formation continued as a sequence of mostly andesitic tuffs with massive bands of whitish grey rhyolite, the Porculla Formation, overlain by siliceous ash-flow, crystal and ignimbritic tuffs and dacitic breccia, of the 39 to 35 Ma Huambos Formation (Noble et al., 1990; Longo et al., 2010; Knight Piésold, 2010). Diorite stocks that crop out to the north of Cajamarca, spanning 4 m.y. in the middle Eocene, may be roots of stratovolcanoes that erupted these volcanic rocks.
In addition, during the late Eocene to Oligocene, two parallel belts of extensional fore-arc grabens/basins were formed to the west of the volcanic arc, separated by a narrow horst that broadly corresponds to the Albian Paracas arch (Benavides-Caceres, 1999).
Part of the Oligocene is characterised by volcanic quiescence throughout much of Peru, which may represent the 30 and 27 Ma Incaic III orogenic pulse (see below). Prior to this magmatic lull, a series of intrusive bodies were emplaced along the highest parts of the Cordillera Occidental in central and northern Peru from the middle Eocene to middle Oligocene, between 39 and 29 Ma. Many of these intrusions were subvolcanic plutons and stocks that diapirically pierced and domed the volcanic country rocks and the underlying Mesozoic sedimentary rocks, and are associated with major faults (Benavides-Caceres, 1999).
During the late Eocene to Oligocene, a system of narrow fault controlled, probably transtensional, intermontane basins were developed along the eastern flank of the lower Calipuy volcanic arc, filled by very thick sequences of continental, largely volcaniclastic, red beds, e.g., the Pocobamba Formation, in central Peru, dated at ~39 to 36 Ma (Noble et al., 1979).
Following the inferred Incaic III break, volcanism again flared throughout the central Andes, to form the upper part of the Lower Calipuy Group, which mainly comprises andesitic and dacitic flows, intermediate to acid pyroclastic rocks, rhyolitic tuffs and some sedimentary intercalations in the south (Noble et al., 1990; McKee and Noble 1982).
During the same period, there was further magmatic activity from the late Oligocene to the earliest Miocene. This activity was located in the Cordillera Oriental, to the east of both the main volcanic arc and the belt of intermontane basins, and largely south of the Abancay deflection, but did extend northward into the southern Peruvian Andes. It occurred as a series of small plutons and dykes of peraluminous monzogranites, alkaline intrusions and volcanic rocks with felsic compositions or shoshonitic affinities that were intercalated with Oligocene to lower Miocene basinal sequences (Bonhomme et al., 1985; Laubacher et al., 1988; Cheilletz et al., 1992; Sandeman et al., 1995). These occurrences have been referred to as volcanic rocks of the eastern province (Audebaud and Amosse, 1981), the inner arc domain (Clark et al., 1983a), or the back arc (Francis and Hawkesworth, 1994).
Also during this late Oligocene to the earliest Miocene interval, the string of intermontane basins that had developed between the eastern flank of the magmatic arc and the Marañón arch was reactivated and filled by thick red beds (e.g., the upper Pocobamba Formation). This latter activity was mainly west of the Marañón arch, which remained elevated, and was the source of sediment deposited as molasse in the eastern foreland basin, east of the sub-Andean fault system (Benavides-Caceres, 1999).
Similarly, following a break in sedimentation and an unconformity, late Oligocene to earliest Miocene deposition re-commenced in the fore-arc grabens/basins.
From the Eocene to the middle Oligocene, deposition in the Eastern Basin formed a brackish marine unit of grey shales and carbonates that includes characteristic tuffaceous beds. These were overlain by late Oligocene red and brown pelitic marls and clays, which were interrupted by the compressive Incaic IV event. Subsequently, the basin was markedly subsident and accumulated a great thickness of upward-coarsening coarse Miocene sediments derived from the rising Cordillera (Benavides-Caceres, 1999).
• Incaic III compressive event, from 30 to 27 Ma representing a break in volcanic activity in the magmatic arc, and a local hiatus and unconformity in the fore-arc basins. This interval also corresponded to a major pulse of movement on the Peltetec-Calacalí-Pujilí-Pallatanga fault complex in the northern Andes section of far northwestern Peru.
• Incaic IV compressive event, dated at ~22 Ma, representing a gently folded, mild deformation and erosional unconformity (the Altos de Camilaca erosional surface), separating the lower and upper Calipuy Volcanic sequences and a temporary reduction in volcanic activity (Benavides-Caceres, 1999).
• Early Miocene - Following the Incaic IV orogenic pulse, renewal of activity within the magmatic arc produced the Upper Calipuy and Tacaza Sequence/Groups in northern and southern Peru respectively. These sequences covered all of the Western Cordillera on both sides of the Abancay deflection, and extended into the adjacent Altiplano in the south. The width of the arc was considerably expanded and volcanic detritus extended into the inner fore-arc basins to the west. The main arc was predominantly composed of intermediate, calc-alkaline, extrusive and subvolcanic magmatism and along its western margin included significant developments of silicic ignimbritic facies (Benavides-Caceres, 1999).
Over much of the western flank of the Cordillera Occidental, the upper Calipuy Sequence is represented by a distinctive sequence of westward thinning rhyodacitic ignimbrites that are up to 1000 m thick. In northern Peru, between Cajamarca and the Ecuador border, this sequence is represented in part by the Chala Sequence (Noble et al., 1990), which is, in turn, part of the Upper Calipuy Group. The Chala Sequence commences with conglomerates and ~23.2 Ma volcaniclastic rocks overlying an angular unconformity. These rocks, include up to 415 m of coarsely porphyritic hornblende and hornblende-pyroxene andesites that are divided in two overlapping sequences, the Tual and Chaupiloma lahars, that are separated by a disconformity. Both are dominated by lahar and hyper-concentrated flow deposits. The Tual andesite, which is restricted to the SW and west of the Cajamarca district, contains poorly reworked epiclastic rocks, pumice rich debris-flow and lahar deposits, as well as volcaniclastic sandstones and pebble conglomerates, and has been dated at 19.53±0.13 Ma (Longo et al., 2010). The Chaupiloma lahars, dated at 15.90±0.18 Ma and mainly found in the NE part of the district, are characterised by thick grey lahar deposits of hornblende-pyroxene andesite, interbedded with rare ash-fall tephra and volcaniclastic sedimentary rocks from pebble conglomerates to laminated siltstone. These ages suggest there may have been a significant time gap between the two units (Longo et al., 2010).
In northern Peru, the gap in the arc to the south of the Cajamarca lineament was maintained.
Coeval intrusions are found in both central and northern Peru, including the dioritic to granodioritic Surco pluton in central Peru, dated at 21.0 and 20.7 Ma (U/Pb; Mukasa, 1984). In northern Peru, Early Miocene granitoid stocks are known to the north of Cajamarca, distributed over a ~5 m.y. interval from 20.8 to 15.9 Ma, including stocks at Misacocha (20.8 Ma; Llosa et al, 1999), the dioritic complex at Michiquillay (20.02 to 19.8 Ma; Noble et al, 2004; Davies and Williams, 2005), El Galeno/Amaro (17.3; Thompson, 2002).
East of the main Upper Calipuy/Tacaza magmatic belt, back-arc activity is represented by the alkaline stocks of Oxapampa in central Peru, dated at between 21.0±1.0 Ma (Tambo Maria syenite porphyry stock) and 19.1±1.0 to 17.4±0.6 Ma (whole rock and plagioclase respectively; all K/Ar; Soler, 1991).
• Quechua I Orogenic Pulse, between ~17 and 15 Ma, although the range may vary along the length of the Andes. During the Middle Miocene, the western section of the Peruvian Andes, in particular the area of the Incaic Fold and Thrust belt, was subjected to a major compressive event (Steinmann, 1929; McKee and Noble, 1982; Megard et al., 1985), also referred to as F3 (Sebrier et al., 1988) or D3 (Ellison et al., 1989). It was succeeded by an erosive period resulting in the Puna erosional surface which truncates the crystalline metamorphic rocks of the Marañón arch in the Eastern Cordillera, the Incaic Fold and Thrust belt, and the Calipuy Volcanic Sequence (Benavides-Caceres, 1999).
Deformation was similar to that of the Incaic pulses, with gentle to no deformation to the west of the Incaic megafault (Noble et al., 1979). The event followed the pattern of the Incaic pulses, with very gentle or no deformation west of the Incaic megafault, and more intense activity to the east (Noble et al., 1979). The maximum deformation was along the southwestern margin of the Incaic Fold and Thrust belt, decreasing toward the NE, except in the vicinity of major faults related to the western flank of the Marañón arch, where it became more intense. The Calipuy Sequence volcanic cover was gently deformed, forming large, open, cylindrical folds that progressively decrease in magnitude toward the NE, with subhorizontal fold axes and vertical to subverticaI axial planes. However, the Incaic faults in the underlying pre-Calipuy strata were reactivated, with many being steepened, and many of the folds overprinted, in places coaxially, by an indistinguishable Quechua I deformation (Soulas, 1975). Along the faulted western margin of the Marañón arch, basement slices with an easterly dip, were upthrust. Quechua I deformation occurred in the upper structural levels, without the development of significant schistosity, and in central Peru involved a NE-SW directed shortening direction (Soulas, 1977).
The Quechua I compressive pulse is interpreted to have followed the extinction of the Pacific-Farallon spreading center, at ~18 Ma, and a westward step to a new spreading center, the ancestor of the east Pacific rise (Mammerickx et al., 1980). At the same time, there was an increase in the convergence rate between the Nazca and South America plates (Pilger, 1983).
Except where cut by major rivers, the Puna erosional surface forms the current Andean uplands at elevations of between 3800 and 4500 m which have a smooth relief, with well-graded, gentle rolling hills and wide open shallow valleys. Above this surface are the large post-Quechua I stratovolcanoes
as well as other major topographic features, such as the Cordillera Blanca. The eastern sections of the Puna erosional surface in the Cordillera Oriental have been subjected to uplift, deep dissection and erosion prior to 10 Ma, and are obscured by intense vegetation (Petersen et al., 1977; Noble et al., 1984, 1990; Noble and McKee, 1999). Along the western flank of the Andes, this surface is warped, forming an inclined plane that descends from ~4400 m to almost sea level, with an average slope of 10% over an interval of ~60 km (Dollfuss, 1965, 1973). However, it has become apparent that the current geomorphic Puna erosional surface represents the convergence and superposition of several pre- and post-Quechua I erosion surfaces, although the dominant component is the Quechua I unconformity (Dalmayrac et al., 1980).
• Middle to Early-Late Miocene, from ~16 to 8 Ma. Formation of the Puna erosional surface was followed by a period of strong normal faulting and intense uplift of up to 1500 m, and erosion of as much as 3 to 4 km in the Cordillera Oriental, decreasing westward towards the coast. The intense fracturing concurrent with uplift led to the reactivation of the intermontane graben/troughs and the formation of new ones, most of which are located along the eastern flank of the volcanic arc, close to the boundary between the Incaic Fold and Thrust belt and the Marañón arch. Some of these grabens were formed well within the arc itself also. Another set of these intermontane basins developed in the arc gap to the south of the NE-SW Cajamarca lineament, filled with ~250 m of late Miocene lacustrine shale, mudstone, sandstone and diatomite and ~400 m alluvial coarse sandstone, fine conglomerate, and reddish shale grading into clast-supported boulder conglomerates.
The uplift and faulting described above was accompanied by intense magmatic activity along the arc, resulting in deposition of the Sillapaca Volcanic Sequence in south and central Peru and equivalents in the north, as well the emplacement of a series of intrusives, including the composite Cordillera Blanca batholith. In the southern part of the Cordillera Oriental, back-arc volcanism was also developed (Benavides-Caceres, 1999).
The 16 to 8 Ma Sillapaca Volcanic Sequence in southern and central Peru is composed of two basic facies, one dominantly volcanic, known as the Sillapaca Group, and the other one dominantly volcano-sedimentary, known as the Maure Group. The Sillapaca Group comprises a thick and widespread pile of flat-lying, or gently warped, volcanic rocks, dominantly lavas, but also pyroclastic flows of mainly dacitic to trachyandesitic composition and of calc-alkaline affinities. They form the cover to the Cordillera Occidental and adjacent plateau, and include both deeply eroded volcanic centres, recent basalt domes. As with the Calipuy Sequence, the western facies of the Sillapaca Volcanic Sequence is represented by silicic ash-flow tuffs intercalated with alluvial gravels (Benavides-Caceres, 1999). The Maure Group is deposited within the intermontane basins and comprises up to 1500 m of lacustrine sediments, siltstones and micritic limestones, intercalated with lavas, ignimbrites, reworked tuffs and soft, porous lapilli, crystal and lithic tuffs.
The main outcrop belt of this volcanic arc is ~100 km wide, and extends along the high crest or plateaus of the Cordillera Occidental (Benavides-Caceres, 1999).
The magmatic arc that produced the Sillapaca Volcanic Sequence also includes a series of intrusive bodies, spread from the middle part of the Pacific slope of the Cordillera Occidental, through the high plateaus and into the Cordillera Oriental. Whilst generally of small size, a prominent exception is the Cordillera Blanca batholith in central to northern Peru. The smaller intrusions include stocks of 13 to 14 Ma tonalites, 10.5±0.3 Ma granodiorite, 13.5 and 12.7 Ma granites, 10 and 7.8 Ma porphyry complexes, 15.0 Ma monzonites, 15.2 and 13.1 Ma rhyodacite at Cerro de Pasco; 8.3 to 8.2 Ma Morococha quartz monzonite porphyry; 9.8 Ma Antamina porphyry; 15.3 to 14.6 Ma Magistral diorite to quartz monzonite; 14.1 Ma diorite and the 9 to 6.8 Ma quartz, feldspar and granodiorite porphyries at Toromocho. The Cordillera Blanca batholith is ~200 x 10 to 20 km in dimensions, with apophyses giving a width of 30 to 35 km. It plunges to both the north and south, as expressed by a string of individual stocks of similar lithology and age. It was emplaced along the Cordillera Blanca fault zone, part of the Incaic megafault system, which marks the western boundary of the more heavily deformed section of the Incaic Fold and Thrust belt. It is is essentially composed of a leucogranodiorite with subsidiary tonalite and granodiorite and monzogranite, surrounded by a spectacular metamorphic aureole. Atherton and Petford (1996) described it as a high sodium leucogranodiorite, with minor diorite and tonalite, and to have calc-alkaline composition with >70% Si02 and a trondhjeimitic character. Petford (1996) proposed that it was produced by partial melting of new mafic mantle material emplaced at the base of the thickening keel of the Cordillera. The batholith rocks have well-defined planar foliation that is particularly intense close to the western fault zone. K/Ar age determinations for the Cordillera Blanca batholith (Landis and Rye, 1974; Stewart et al., 1974; Cobbing et al., 1981; Petford and Atherton, 1992) fall into two broad groups: an older 12 to 9 Ma tonalite-granodiorite, and a younger 6.3 to 2.7 Ma leucogranite forming the bulk of the batholith. The 12 to 9 Ma group is envisaged as emplaced at depths of about 5 km below the Quechua I surface, accompanying intense sinistral transtensional faulting. The 8 to 7 Ma magmatic gap is attributed to the Quechua II orogenic pulse, followed by a return to an extensional regime from 7 to 5 Ma, terminated by the weak compressive 5 to 4 Ma Quechua III deformation, and the resumption of extension from 4 Ma to the present (Benavides-Caceres, 1999).
In northern Peru, to the north of the Cajamarca Lineament in the Cajamarca-Yanacocha area, the ~15 Ma hiatus at the top of the Upper Calipuy Group, is followed by younger andesitic and dacitic lavas, domes, ignimbrites, and minor subvolcanic intrusions, dykes, and breccias of the 14.5 to 8.4 Ma Yanacocha Volcanic Complex (Longo et al., 2010). This complex has been subdivided (after Teal and Benavides, 2010) into the: Lower Andesite Sequence, mainly lavas which are 20 to 320 m thick and equivalent to the 14.5 to 13.3 Ma Atazaico Andesite (Llongo et al., 2010)> These are followed, after a volcanic gap, by the Yanacocha Pyroclastic Sequence, including the explosive Cori Coshpa trachyandesitic ignimbrite and overlying Maqui Maqui dacitic ignimbrite, that together constitute the 12.6 to 12.4 Ma, 50 to 225 m thick Colorado Pyroclastic Sequence. These are overlain by the Upper Andesite Sequence, which comprises intercalated andesitic to dacitic to rhyolitic flows and ignimbrites dominated by multiple flow dome complexes. It commences with the 45 to 310 m of pyroxene-hornblende andesitic to dacitic lavas (of the regional effusive, 12.1 to 11.6 Ma Azufre Andesite) and the explosive, 40 to 350 m of ignimbrites (the 11.5 to 11.2 Ma, San Jose Ignimbrite). These more substantial units are overlain by a condensed sequence of 10.78±0.05 Ma flow foliated dacitic domes and associated silicified laminated rocks (Corimayo dacitic domes), the Cerro Yanacocha dacite porphyry intrusion, and the post-mineral Yanacocha Lake rhyolitic dyke and coeval welded rhyolitic, 8.43±0.04 Ma Negritos rhyolitic ignimbrite. Intrusions within this area comprise multiple pulses of late-stage dykes, plugs and stocks have been intruded into the volcanic pile, ranging in age from 14 to 8.4 Ma (Turner, 1997; Longo, 2005).
No volcanism is evident in Northern Peru, to the NNW of Cajamarca since ~8.4 Ma at the onset of the Quechua II Orogenic Pulse (Longo et al., 2010).
New fore-arc basins, including the Sechura basin in northern Peru, were formed along the western flank of the volcanic arc, containing up to 250 m of argillaceous and calcareous quartz sandstones, with bentonitic shale interbeds, and up to 1000 m of diatomaceous and bentonitic shales, with minor tuffaceous sandstone, siltstone, diatomites and bentonites (Benavides-Caceres, 1999).
During this period, the back-arc magmatic belt, established in the Cordillera Oriental of southern Peru during the Oligocene, resumed activity in association with the intermontane basins, marked by the presence of peraluminous volcanic and intrusive rocks (Clark et al., 1983, 1990; Laubacher et al., 1988), with two main pulses, from 17 to 15 and 10 to 7 Ma respectively.
Along the eastern flank of the Cordillera Oriental, subsidence in the Eastern Foreland Basin underwent a marked acceleration. During this period, the deltaic Lower Ipururo Formation of reddish argillites, cross-stratified sandstones, micro-conglomerates and medium to coarse sandstones were deposited over the Incaic III unconformity within this basin, and is capped by another hiatus separating it from the grey to black marls and limestones and fine-grained hummocky cross-stratified calcarenites of the thin Middle Ipururo Formation (Benavides-Caceres, 1999).
• Quechua II Orogenic Pulse, between ~8 and 7 Ma, reflected by a significant unconformity that separates the Sillapaca Volcanic Sequence
and older rocks in the south from the overlying Barroso Volcanic Sequence (8 Ma to present). Deformation, which had commenced between 10 and 8 Ma, was most intense in the sub-Andean belt, forming the Sub-Andean Fold and Thrust belt between the Marañón arch and the Brazilian shield, but predominantly on the western margin of the Eastern Foreland Basin. It largely composed of east-vergent, asymmetric folds and thrust faults, with considerable variations in width and structural style in different segments. To the east it passes into a undeformed, west-dipping monocline of the foreland area, where eastward-thinning sedimentary units lie over the Brazilian shield. During this period, the Marañón arch was compressed and uplifted between inward-dipping reverse faults along both of its margins (Benavides-Caceres, 1999).
• Latest Miocene to Pleistocene - The Quechua II orogenic pulse was followed by renewed extension and uplift, accompanied by volcanic activity, interrupted by the Quechua III and IV compressive pulses at 5 to 4 and 2.0 to 1.6 Ma respectively. The bulk of the uplift was prior to 6 Ma, with only ~ 500 to 600 m subsequently, accompanied by deep incision to form the present cordilleran topography.
Post-Quechua II volcanic activity is represented by the Barroso Volcanic Sequence, accompanied by the continuing development of the intermontane graben basins between the eastern flank of the arc and the Marañón arch, and of the fore-arc basins located in the submerged area between the western flank of the arc and the trench. Following the Quechua III event, the intermontane basins were once again reactivated, and filled with lacustrine sediments and epiclastic rocks, whilst the fore-arc basins similarly recommenced subsidence and deposition of up to 2300 m of deltaic siliciclastic rocks.
The Barroso Volcanic Sequence has an unconformable to transitional contact with the underlying Sillapaca Volcanic Sequence, and in the type area of the Barroso Group comprises ~1500 m of greyish andesitic and trachytic tuffs and lavas, as well as post-glacial ash-flow units and lahars. They represent a calc-alkaline suite (Jakes and White 1972) without any tholeiitic component (Lefevre, 1973; Klincketal., 1986; Ellison et al., 1989), in which the intermediate lavas, andesites, and dacites are the most abundant lithologies. They are largely composed of andesitic lavas and pyroclastic deposits arranged in simple or composite stratovolcanoes, where they have high initial dips. The sequence is split into a Lower 7 to 5 Ma and Upper 4 Ma to present, Barroso Volcanic Sequence by the intervening Quechua III compressive pulse.
Throughout the late Miocene and Pliocene, particularly since the Quechua III compressive pulse, volcanic activity receded southward, reaching its present position after the Quechua IV event. The gradual north to south quenching of the volcanic activity between 3 and 14°S, corresponding to the interval between the Huancabamba and Abancay deflections respectively, reflects the progressive flattening of the subducted slab over this interval as thicker oceanic crust between the Carnegie and Nazca oceanic ridges enters the subduction zone. To the north and south of these deflections, current chains of stratovolcanic cones are related to Pleistocene and Holocene continental arc volcanism that forms a broad zone centred on the Cordillera Occidental.
The late Miocene and Pliocene volcanism was accompanied by the greatly intensified subsidence of the eastern foreland basin where the Upper Ipururo Formation was deposited unconformably above the Middle Ipururo Formation during the late Miocene and Pliocene. This unit comprises a fluvial sequence of cross-bedded conglomerates of well-rounded pebbles, intercalated with siltstones and argillites and cross-bedded sandstones. With the Lower and Middle members, this formation reaches a maximum of 5700 m in thickness (Hermoza et al., 2005). When this unit had been deposited, the Eastern foreland basin had reached its maximum subsidence, accommodating >10 km of Tertiary and Quaternary sedimentary rocks. This represents a combined down-throw immediately to the east of the exposed Proterozoic to Palaeozoic basement in the Marañón arch of the order of 12 km in northern Peru, and ~4 km in the south of the country, with a thinner succession between the two extremes. During the Pliocene, there are a number of small, isolated volcanic plugs or chimneys of 5.4 to 4.4 Ma (Stewart, 1971) peralkaline volcanic rocks, described as undersaturated alkalic rocks, comprising feldspathoid phonolite and alkali trachyte (James, 1978), intruded into the Sub-Andean Fold and Thrust belt (Benavides-Caceres, 1999).
• Quechua III Orogenic Pulse, between ~5 and 4 Ma, was a gentle compressive event which is reflected by locally recognisable unconformities (Benavides-Caceres, 1999).
• Quechua IV Orogenic Pulse, between ~2 and 1.6 Ma, close to the boundary between the Pliocene and the Pleistocene, when in general, the entire Andean Orogen was under compression (Mercier et al., 1992). It is marked by local unconformities between overlying fluviolacustrine terraces and fans as well as by glacial deposits and underlying volcanosedimentary sequences (Benavides-Caceres, 1999).
• Middle Pleistocene to Present - Following the Quechua IV compressive pulse, the geodynamic pattern changed to a regime characterised by
north-south extension along the Cordillera, particularly in its higher sections, and compressional conditions, both along the Sub-Andean Fold and Thrust belt and the western marginal zone between the Nazca and South American plates. This pattern has been interpreted as a balance between boundary forces related to plate convergence and gravitational forces related to isostatically elevated masses (Sebrier et al., 1985, 1988; Mercier et al., 1992; Assumpçao, 1992).
Distribution of Mineralisation
Porphyry style mineralisation in the Peruvian Andes, between the Huancabamba and Abancay 'deflections', is mainly distributed along the eastern margin of the Cordillera Occidental. Associated porphyry stocks intrude either Tertiary volcanic and/or Cretaceous sedimentary rocks, frequently carbonates, either within the eastern edge of the volcanic arc, or up to 50 km to the east. The greatest density of deposits is in the Cajamarca Mineral Belt between the Ecuadorian border and the NE-SW Cajamarca Lineament that defines SE margin of the tertiary arc, prior to a 50 km gap in the arc. Within the same mineral belt, the greatest density is within the southeastern 100 km of this part of the arc.
• Cajamarca Mineral Belt, which occupies the northern Peruvian volcanic belt, includes the giant Yanacocha complex of deposits, an extensive mineral system that spans the range of ore styles form the deeper Kupfertal porphyry Cu-Mo mineralisation, passing up into associated high sulphidation lithocap Cu-Au and epithermal Au ores of the main Yanacocha deposits. The upper 50 to 300 m of the system has been strongly oxidised. Sections have been scoured and physically transported by Pleistocene glaciers, and partially reworked, to form the La Quinua deposits. The Yanacocha group of deposits were formed between 13.5 and 8.2 Ma, and define an ~18 x 6 km corridor aligned along the 50 to 60° trending Chicama-Yanacocha trans-orogen structure, immediately to the north of, and parallel to, the Cajamarca lineament which marks the start of the volcanic gap in the Tertiary arc.
To the east of Yanacocha, on the eastern margin of the preserved arc, there are a group of Miocene porphyry deposits, emplaced between 20 and 10 Ma, distributed over a 50 x 10 km, NW-SE elongated zone that includes:
Michiquillay (20.0 to 19.8 Ma),
El Galeno (17.5 to 16.5 Ma),
Minas Conga (Chailhuagón, Perol and Amaro; 15.8 to 15.6 Ma),
Cerro Corona (14.3 to 10.5 Ma), and the Tantahuatay (13.3 to 8.3 Ma) high sulphidation Au-Ag deposit, The smaller La Zanja (15.6 to 11.9 Ma) high sulphidation gold deposit (24 Mt @ 0.8 g/t Au; Cardozo and Bustamante, 2013) is 30 km to the SW of Tantahuatay, and NW of the centre of Yanacocha. A further 60 km NW along the same trend is the
La Granja (14 to 10 Ma) deposit, followed some 35 km further NW by Cañariaco (Cañariaco Norte, Cañariaco Sur and Quebrada Verde; 17.9 to 15.8 Ma) and
Rio Blanco (16 Ma), still further to the NW close to the Ecuadorian border. Across the border ~100 km to the NNW in southern Ecuador there is another cluster of Porphyry Cu deposits of the same age (see the North Andes - Panama Cu-Au Province in Ecuador record). The porphyry deposits of the Cajamarca Mineral Belt incorporates two geochemically distinct groups along this trend namely: i). porphyry Cu-Mo±Au deposits which include La Granja, Michiquillay, El Galeno, Cañariaco and Rio Blanco; and ii). porphyry Cu-Au deposits which include Cerro Corona, Minas Conga and La Carpa.
• Central Peru Mineral Belt, which extends from the northern tip of the Tertiary volcanic arc where it resumes after the 50 km break south Cajamarca, and extends south to the Abancay 'deflection'. This belt, which is ~120 km wide and 700 km long, contains a series of clusters of porphyry Cu±Au±Mo±Zn, polymetallic porphyry/skarn, epithermal Au±Ag, and Cordilleran epithermal polymetallic deposits. Almost all are directly related to Miocene magmatism and represent different erosional levels and temporal phases of large intrusive-related hydrothermal regimes.
The northern-most cluster includes the Quiruvilca (middle Miocene) Cordilleran epithermal polymetallic Pb-Cu-Zn-Ag deposit within the Tertiary volcanic arc. Approximately 12 km ENE of Quiruvilca, the Lagunas Norte (17.0±0.22 Ma) high-sulphidation epithermal Au deposit is hosted by an inlier of Upper Jurassic to Lower Cretaceous basement within the Tertiary volcanic arc. Next, some 10 km further to the ENE is the La Arena (~25 to 24 Ma) high-sulphidation epithermal Au telescoped onto a porphyry Cu-Au deposit on the northeastern margin of the preserved volcanic arc, the oldest of the porphyry systems in the Peruvian Andes. Approximately 30 km to the NNW, some 15 to 25 km to the NE of the the Tertiary volcanic arc, the Shahuindo (~16 Ma) intermediate sulphidation Au deposit overprints early high sulphidation mineralisation, associated with Miocene igneous intrusion within Mesozoic basement. The Magistral (15.3 to 14.6 Ma) porphyry-skarn Cu-Mo deposit is located ~50 km SE of La Arena, associated with Miocene intrusion into carbonate rich Cretaceous basement, ~30 km east of the preserved exposures of the main volcanic arc.
The next significant cluster of deposits is ~200 km SSE of Quiruvilca, and includes the Pierina (~14.9 Ma) high sulphidation epithermal Au deposit, ~10 km to the north of Huaraz, deposited within Miocene volcanic rocks of the main Tertiary volcanic arc. A group of smaller mines and the Soledad prospect are located in a similar setting, 20 km south of Huaraz. The Hilarion Cordilleran epithermal polymetallic deposit, 55 km SSE of Huaraz, contains 52 Mt @ 4.53% Zn, 0.70% Pb, just to the east of the Tertiary volcanic arc. The other main deposit in this 'cluster' is the Antamina (~9.8 Ma) porphyry/skarn Cu-Mo-Zn deposit, located ~30 km to the east of the main Tertiary magmatic arc and 50 km east of Huaraz.
Most of the previous deposits are located on the eastern slopes of the Cordillera Occidental. However, the next cluster is located ~175 km SE of Huaraz, on the western slopes of the Cordillera Oriental, further from the current Peru Trench, and is almost exclusively composed of Cordilleran epithermal polymetallic Cu-Zn-Pb-Ag deposits, with some associated high sulphidation epithermal gold mineralisation. The key deposits include Cerro de Pasco (15.4 to 15.1 Ma), the Colquijirca District (10.8 to 10.6 Ma polymetallic ores overprinting ~11.9 to 11.1 Ma high-sulphidation Au-Ag mineralisation) group of deposits, 8 km south of Cerro de Pasco, the Huaron (Miocene?) deposit, 37 km SSW of Cerro de Pasco, and the Atacocha and El Porvenir (mid-Miocene?) deposits, 10 km north of Cerro de Pasco.
Another significant cluster is located 100 km south of Cerro de Pasco, and 265 km SSE of Huaraz, on the eastern slopes of the Cordillera Occidental, in the Morococha district (~7 Ma Toromocho porphyry Cu-Mo and marginally younger surrounding Morococha Cordilleran epithermal polymetallic vein and manto replacement Cu-Zn-Pb-Ag deposits) on the eastern margin of the Tertiary volcanic arc. The Casapalca and Yauliyacu polymetallic Ag-Pb-Zn-Cu vein cluster, which has produced >6100 t of Ag over the last 100 years, is located ~12 km WSW of Morococha.
The polymetallic Yauricocha Cu-Au-Ag-Zn-Pb deposit is located ~165 km to the SSE of Morococha, close to Abancay Deflection. It dominantly comprises high-temperature, pipe-like, limestone replacement sulphide bodies containing massive pyrite with enargite, bornite, chalcopyrite, galena and sphalerite, located in >10 separate bodies over an interval of ~9 km along the contact with a granodiorite mass. The near-vertical intrusions of Miocene granodiorite are fringed by an aureole of calc-silicate alteration within the intruded Middle Cretaceous Jumasha Formation limestone and Celendín Formation chert, marl and lutite. NI 43-101 compliant mineral resources in 2015 (SRK, 2015) were measured + indicated resources - 7.871 Mt @ 61 g/t Ag, 0.67 g/t Au, 1.12% Cu, 0.94% Pb, 2.70% Zn, plus inferred resources of 3.745 Mt @ 49 g/t Ag, 0.53 g/t Au, 1.33% Cu, 0.58% Pb, 1.86% Zn.
• Other Cu and Base Metal Deposits. To the south, a string of IOCG occurrences and deposits extends across the Abancay/Pisco Deflection from the Central Andes, ~200 km south of Lima, and includes deposits such as Raul-Condestable and Eliana.
Also to the SW, the Cerro Lindo volcanic-hosted massive sulphide Zn-Pb-Cu-Ag deposit is hosted by Cretaceous volcano-sedimentary rocks of the Western Marginal (or Huarmey-Cañete) trough, whilst in the inferred northern extension of the same trough, the Lancones basin in far NW Peru, the Tambo Grande volcanic-hosted massive sulphide Zn-Pb-Cu-Ag deposit (Inferred resource - 42.3 Mt @ 1.47% Zn, 0.36% Pb, 2.04% Cu, 37.7 g/t Ag; Northern Miner, May, 1999) is hosted by Cretaceous volcanic rocks of the Lancones volcanic complex.
In addition, there are a number of carbonate hosted ("Mississippi Valley Type") deposits known on the western margin of the Eastern Foreland basin in the central and northern section of the Peruvian Andes. These include San Vincente, ~120 km SE of Cerro de Pasco, and Shalipayco (total resource - 28 Mt @ 5.06% Zn, 0.48% Pb, 35.9 g/t Ag; Milpo, 2014) in the Junin district, 35 km SE of Cerro de Pasco, and Bongará (total resource - 13.6 Mt @ 10.48% Zn, 1.24% Pb, 12.6 g/t Ag; Milpo, 2014), 200 km NNE of Cajamarca. All are hosted by carbonate units of the Late Triassic to early Jurassic Pucara basin.
The most recent source geological information used to prepare this decription was dated: 2010.
This description is a summary from published sources, the chief of which are listed below. © Copyright Porter GeoConsultancy Pty Ltd. Unauthorised copying, reproduction, storage or dissemination prohibited.
|
|
|
Benavides-Caceres, V., 1999 - Orogenic Evolution of the Peruvian Andes: The Andean Cycle: in Society of Economic Geologists, Denver, Special Publication No. 7 pp. 61-107.
|
Chew, D.M., Cardona, A. and Miskovic, A., 2010 - Tectonic evolution of western Amazonia from the assembly of Rodinia to its break-up: in International Geology Review v.53, pp. 1280-1296.
|
Chew, D.M., Kosler, J., Whitehouse, M.J., Gutjahr, M., Spikings, R.A. and Miskovic, A., 2007 - U-Pb geochronologic evidence for the evolution of the Gondwanan margin of the north-central Andes: in GSA Bulletin v.119, pp. 697–711.
|
Ramos, V.A., 2008 - The Basement of the Central Andes: The Arequipa and Related Terranes: in Annual Review of Earth and Planetary Sciences v.36, pp. 289-324
|
Rosas, S., Fontbote, L. and Tankard, A., 2007 - Tectonic evolution and paleogeography of the Mesozoic Pucara Basin, central Peru: in J. of South American Earth Sciences v.24, pp. 1-24.
|
Porter GeoConsultancy Pty Ltd (PorterGeo) provides access to this database at no charge. It is largely based on scientific papers and reports in the public domain, and was current when the sources consulted were published. While PorterGeo endeavour to ensure the information was accurate at the time of compilation and subsequent updating, PorterGeo, its employees and servants: i). do not warrant, or make any representation regarding the use, or results of the use of the information contained herein as to its correctness, accuracy, currency, or otherwise; and ii). expressly disclaim all liability or responsibility to any person using the information or conclusions contained herein.
|
Top | Search Again | PGC Home | Terms & Conditions
|
|