Igarape Cinzento, Alvo GT-46 |
|
Para, Brazil |
Main commodities:
Cu
|
|
 |
|
 |
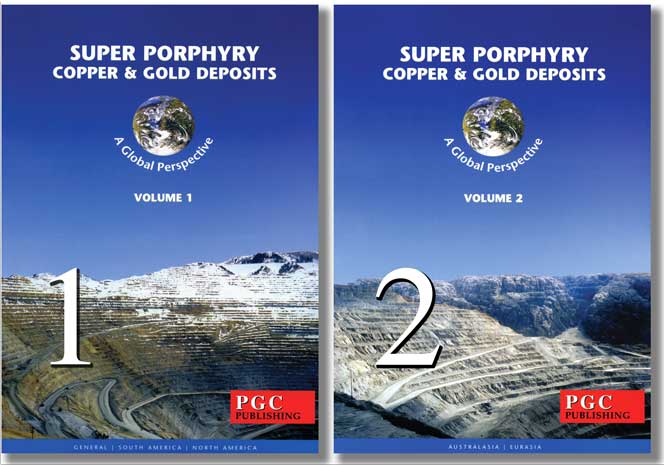 |
Super Porphyry Cu and Au

|
IOCG Deposits - 70 papers
|
All papers now Open Access.
Available as Full Text for direct download or on request. |
|
 |
The Igarapé Cinzento (Alvo GT-46) IOCG deposit is located in the northwestern part of the Cinzento shear zone in Carajás IOCG Province, Para State, Brazil, ~45 km WNW of Salobo and 120 km WNW of Parauapebas.
Regional Setting
See the Carajas IOCG Province record.
Geology
The Igarapé Cinzento/Alvo GT-46 deposit lies along the subvertical limb of a fold deforming a metavolcanosedimentary sequence of the Grão Pará Group, a member of the Itacaiúnas Supergroup. In the deposit area, the host sequence comprises amphibolites alternating with biotite- and actinolite-biotite schists, gradually transitioning into almandine-biotite schists and biotite-almandine schists with interlayered metamorphosed banded iron formations (BIFs). The sequence is cut by foliated tonalite to granodiorite, pegmatite, granites and dolerite dykes (Toledo et al., 2019).
The main lithostratigraphic units are as follows (as described by Toledo et al., 2019):
Amphibolite - is a fine- to medium-grained, dark green, granoblastic to locally blastoporphyritic rock with generally evenly spaced foliation planes. It comprises approximately 40% hornblende, 25% plagioclase, 15% quartz and 10% diopside with 5% magnetite, 3% biotite, 1% ilmenite and 1% titanite. This metamorphic assemblage generally has a polygonal granoblastic texture, with minerals aligned along the dominant foliation planes. Foliation is accentuated by >1 to <10 mm leucocratic bands containing 250 to 500 µm plagioclase, hornblende and minor quartz crystals, alternating with melanocratic layers composed of ~250 µm to 2 mm hornblende crystals. Blastoporphyritic textures are locally developed containing mantled porphyroclasts of 5 to 10 mm plagioclase.
Biotite- and actinolite-biotite schists are fine- to medium-grained, granolepidoblastic to mylonitic rocks that are dark grey, composed of 40 to 50% biotite, 30% plagioclase, 20% quartz and where present, up to 10% actinolite. Intensely deformed domains contain as much as 80 to 90% comminuted quartz-plagioclase-biotite grains that are 50 to 100 µm in size, with 10 to 20% ~400 µm plagioclase porphyroclasts. Foliation is conspicuous, varying from discrete to continuous and anastomosing mineral banding marked by the preferred orientation of recrystallised quartz and plagioclase crystals and mafic minerals. Sigmoidal biotite and actinolite foliation delineates mylonitic S planes, deflected by C surfaces, to form S-C shear fabrics.
Biotite, which is subhedral, ranges from 500 µm to 2 mm in size, and in intensely deformed zones,has undulose extinction, folding, kinking and sigmoidal shapes. Anhedral plagioclase crystals are tapered with bent twinning planes, and occur as recrystallised <50 µm grains around cores of porphyroclasts, forming both core and mantle structures. Quartz has interlobate grain boundaries and a bimodal grain size distribution. Recrystallised equigranular, <100 µm quartz occur between ~500 µm porphyroclasts with undulose extinction. Quartz ribbons are observed in mylonitic zones, alternating with biotite layers and recrystallised plagioclase. Acicular to elongate prismatic actinolite occurs as crystal aggregates.
Almandine-biotite schist to biotite-almandine schist, which are dark green, fine- to coarse-grained, lepidoblastic and intensely foliated, porphyroblastic rocks composed of 10 to 30% almandine porphyroblasts set within a matrix of 20 to 40% biotite, 30% quartz, 10% plagioclase and rare amphibole (actinolite). Almandine poikiloblasts are fractured and with inclusions of very small quartz, ~3% tourmaline, 2% apatite and albite crystals. The principal foliations form an anastomosing, spaced pattern of schistosity. Earlier foliation planes are preserved as trails of inclusion within poikiloblastic almandine. The youngest foliation is marked by the orientation of combined recrystallised quartz and plagioclase within the axial planes of folded biotite crystals, and varies from zonal to continuous to anastomosing.
Almandine is commonly rotated and locally folded. Biotite surrounds almandine, commonly with pressure shadows. Biotite may be deformed with kink bands. Quartz has loboid boundaries with deformation lamellae and a bimodal grain size distribution, comprising approximately uniform-sized, up to 0.5 mm recrystallised grains separating up to 3 mm porphyroclasts with undulose extinction. Like quartz, plagioclase also has a bimodal size distribution, with large crystals which have tapering twinning planes and undulose extinction. Actinolite occurs as subhedral prismatic grains.
Metamorphosed banded iron formation (BIF) occurs as coarse-grained, grey, granoblastic rock with 1 to <20 mm laminae/bands. It is composed of 45% quartz, 40% magnetite, ~10% grunerite and 5% almandine. The principal foliation is defined by alternating bands of quartz and magnetite. Recrystallised quartz and magnetite and stretched magnetite delineate an anastomosing later, parallel foliation. Quartz grains with undulose extinction have irregular to loboid grain boundaries, and incipient deformation lamellae. Small to >1 mm magnetite crystals are subhedral to anhedral with a prismatic or granular habits. Grunerite occurs as acicular to prismatic aggregates, whilst almandine locally surrounds magnetite crystals.
Intrusive rocks - The hydrothermally altered host sequence is cut by tonalite/granodiorite, pegmatite, granite and dolerite dykes. The contact zone between pegmatites and the host sequence is characterised by almandine, quartz and biotite crystals that are up to 5 cm across and by late pervasive chlorite alteration of the wall rocks. Pegmatite and granite cut the foliated tonalite/granodiorite. Dolerite dykes are only locally observed crosscutting the biotite schists.
The principal intrusive rocks are as follows (as described by Toledo et al., 2019):
Foliated granodiorite-tonalite - a light grey or brownish pink, medium- to fine-grained rock, composed of 40 to 50% plagioclase, 30% quartz, 5 to 20% K feldspar (orthoclase and microcline) and 5 to 15% biotite, with up to 3% secondary chlorite and as much as 2% epidote. Feldspar and quartz crystals have interlobate boundaries, with incipient to well-defined grain orientations, and have a bimodal grain size distribution. Quartz crystals have undulose extinction with subgrain development, whilst plagioclase crystals have tapering twin planes. Subhedral biotite, along with oriented quartz and feldspar crystals, define the foliation planes. Secondary chlorite partially to totally replaces biotite and also occurs associated with epidote, filling late veins and veinlets.
Pegmatite is light pink and composed of a coarse grained assemblage that includes ~30% albite, 30% quartz, 25% microcline and 7% biotite, with ~2% muscovite, 1% epidote, <1% tourmaline, minor molybdenite and <<1% chalcopyrite, and ~5% secondary chlorite. Large feldspar and quartz crystals have poorly defined margins and show incipient development of smaller subgrains. The pegmatites are emplaced along foliation planes and lithological contacts in the enclosing rocks, forming both discordant and concordant bodies. Microcline psuedomorphs perthitic textures with exsolved Na-rich lamellae. Secondary chlorite replaces biotite on its outer margins and along cleavages. Pegmatites are also altered to a Ca-rich assemblage of carbonate, epidote and sericite that spreads through plagioclase crystals or along their rims. Potassic metasomatism is locally observed as <100 µm veinlets of K feldspar.
Granite is isotropic, light pink, and composed of 30% microcline, 25% quartz, 20% plagioclase, 10% chlorite, 10% biotite and 5% epidote, with pre- served phaneritic 'non-equigranular' texture. Microcline has igneous exsolution textures, with perthitic albite lamellae. Quartz has incipient subgrain development, with polygonal contacts between crystals. Plagioclase is partially to totally altered to carbonate, epidote and sericite, whilst potassic metasomatism is reflected by the presence of <50 µm orthoclase veinlets that cut microcline crystals.
Dolerite is dark green, fine- to medium-grained and occurs as a 40 m wide, undeformed dyke with subophitic textures. It is composed of ~30% primary plagioclase, 5% augite, 5% magnetite and 1% ilmenite, with ~35% secondary amphibole (hastingsite), 15% scapolite and 9% epidote. Augite occurs as rare preserved cores that have been intensely altered and replaced by hastingsite, but is also replaced by epidote and scapolite. Plagioclase is found as elongated tabular crystals partially included within hastingsite/augite crystals, preserving the subophitic igneous texture.
Hydrothermal Alteration and Copper Mineralisation
The hydrothermal alteration at the GT-46 deposit is characterised by a number of different styles of mineral associations, and their pattern of distribution.
The earliest alteration comprises a regional sodic-calcic assemblage, followed by a potassic suite, iron metasomatism and then disseminated mineralisation (mineralisation I). The zones of disseminated hydrothermal alteration and ore minerals define ductile foliations that follow early foliation planes, occurring adjacent to metamorphic minerals (e.g., biotite, hornblende, plagioclase, almandine) or partially replacing them along crystal contacts, or cleavage planes. These initial mineralised zones are cut by veins and breccias of mineralization II, which are composed of massive magnetite and chalcopyrite. Late alteration zones, characterised by pervasive chloritisation, are spatially associated with granitoids, which intersect all hydrothermally altered and mineralised zones (Toledo et al., 2019). The main alteration assemblages are (from Toledo et al., 2019).
Sodic-calcic alteration is reflected by the occurrence of hastingsite and albite. Hastingsite occurs through selective replacement of metamorphic hornblende, actinolite, grunerite and biotite along crystal rims in all host rocks. Hydrothermal albite has discontinuous irregular and undeformed twin planes, and overgrows metamorphic plagioclase or alters it along crystal margins. Albite is developed interstitially to the host rock crystals in amphibolites and almandine-biotite schists (Toledo et al., 2019).
Potassic alteration is defined by anhedral hydrothermal biotite (biotite II) which replaces metamorphic amphibole along crystal margins and cleavage planes in all host rocks, forming overgrowth halos in metamorphic biotite within biotite schist. It also infills almandine fractures and plagioclase cleavages within almandine-biotite schist and is found along magnetite boundaries and fractures in BIFs. Biotite II also replaces hydrothermal hastingsite and is precipitated along albite grain margins or forms thin (up to 20 µm) veinlets (Toledo et al., 2019).
Hydrothermal Magnetite (magnetite II) crystals increasingly replace and pseudomorph almandine, to form in iron-enriched zones within almandine-biotite schist. Massive magnetite II also occurs as inclusions in chalcopyrite and as overgrowths along the margins of metamorphic magnetite I.
Epidote, allanite and clinozoisite are frequently associated with biotite II and albite, and occur as euhedral to subhedral zoned crystals intergrown with sulphides and magnetite II. Together with anhedral scapolite, which may be partially included in chalcopyrite within the BIFs, these minerals represent the gangue assemblage within the mineralisation I ore zones (Toledo et al., 2019).
Disseminated mineralisation I is preferentially developed in spatial association with Fe metasomatised zones. Ore minerals comprise 35 to 40% chalcopyrite with 30 to 35% bornite, associated with 25 to 35% magnetite II, minor molybdenite and pyrite, and accessory cassiterite, sphalerite, pentlandite, tellurides (Bi, Ag, Ni, Pb) and late chalcocite. Chalcopyrite, bornite, and magnetite II are the dominant minerals of the ore assemblage, occurring as disseminated prismatic/granular crystals or massive aggregates that define a late foliation, filling intermineral spaces or mineral cleavages and fractures (e.g., biotite and almandine). Molybdenite, pyrite and accessory ore minerals may occur as inclusions in chalcopyrite or as small crystals between the major ore minerals. Chalcocite replaces bornite along the rims of the latter or as thin veinlets. Native gold is found as rare inclusions in tellurides (Toledo et al., 2019).
Mineralisation II differs form mineralisation I, crosscutting the earlier hydrothermally altered rocks and ore zones, and host-rock structures. It occurs as breccia and fracture fillings and as veins/veinlets. It is less extensive in the deposit than mineralisation I, and is characterised by Mg- and Ca-rich minerals (e.g., chlorite and calcite), and by magnetite III, chalcopyrite II, quartz II and subordinate allanite II and albite II. Magnetite III and chalcopyrite II crystals, commonly with associated allanite II, have a medium grain size and are subhedral. Chlorite, calcite, and albite II form massive aggregates (Toledo et al., 2019).
Ore breccias are characteristic of mineralisation II, composed of magnetite, quartz and chlorite fragments set in a matrix of variable amounts of chalcopyrite, magnetite and chlorite ±calcite (Toledo et al., 2019).
Zoned veins or veinlets are composed of a chalcopyrite-magnetite core, with chlorite and/or calcite rims. In some magnetite-calcite contacts, acicular grains of both minerals are present. Thin, <10 µm inclusions of the carbonate-fluoride mineral bastnä̈site [(Ce, La)CO3F] are associated with allanite grains accompanied by considerably contents of rare earth elements such as Ce, La, Nd, and Y, which are only identified in SEM analysis (Toledo et al., 2019).
Late alteration is defined by up to 10 cm wide pervasive chloritisation halos and large biotite, quartz and almandine crystals that are spatially associated with pegmatites and quartz albite veins related to granitoids. The pegmatites, veins and these pervasive halos crosscut all host rocks, with pervasive replacement obliterating the primary textures and previous alteration assemblages. Up to 5 cm wide quartz III, almandine II, and biotite II crystals are associated with <1 mm massive chlorite II crystals that occur in a random pattern. Subordinated chalcopyrite III and molybdenite II are generally evident. Euhedral to subhedral almandine is commonly partially to totally replaced by chlorite, forming chlorite pseudomorphs after garnet. This stage includes Mineralisation III (Toledo et al., 2019).
Geochronology and chemistry
The evolution of the Igarapé Cinzento deposit and its host succession includes the following events (Toledo et al., 2019):
• Deposition of the host amphibolite in the Grão Pará Group and Itacaiúnas Supergroup at 2774 ±19 Ma (25 grains LA-ICP-MS U-Pb zircon). This age is interpreted to be the age of igneous protolith crystallization and, therefore, the formation of the volcanosedimentary sequence;
• Amphibolite facies tectonometamorphic event (Dn), between 2.77 and 2.72 Ga;
• Development of the Cinzento shear zone (Dn+1) at 2.72 Ga, with coeval circulation of hydrothermal fluids related to the development of alteration zones and mineralization I (Re-Os ICP-MS from mineralisation I molybdenite);
• Superimposed hydrothermal events at ~2.6 (U-Pb monazite and Re-Os molybdenite) related to mineralisation II, and ~2.5 Ga (Re-Os molybdenite) from a late stage mineralisation III spatially associated with granitoid intrusions;
• Regional granitoid emplacement at ~2.5 Ga (U-Pb zircon), based on the similar age of the foliated granodiorite and undeformed granitic rocks and pegmatites.
The Igarapé Cinzento (Alvo GT-46) deposit resulted from overprinting hydrothermal processes during at least two distinct mineralisation
events. Alteration styles reflect differences in the mineralising regime, which progressed from early ductile deformation to later by brittle fracturing.
Early hydrothermal alteration, characterized by sodic-calcic and then potassic assemblages as well as iron metasomatism with associated disseminated mineralisation I, was controlled by ductile structures depositing chalcopyrite-bornite-magnetite, and 2718 ± 56 Ma, Re-Os molybdenite).
Veins and breccias containing chalcopyrite-magnetite(-chlorite-calcite-quartz) were emplaced during an overprinting episode of mineralization II at ~2.6 Ga.
Late alteration at ~2.5 Ga represents fluid remobilisation with chloritisation and crystal growth spatially related to pegmatite intrusions. Re-Os molybdenite ages of 2503 ±51 and 2449 ±44 Ma provide evidence of younger episodes of hydrothermal fluid circulation within the deposit.
Fluid inclusion data indicates early alteration zones were related to aqueous-saturated, highly saline (32 to 50 wt.% NaClequiv., total homogenisation temperature [Tht] = 203 to 450°C) and carbonic fluids (CO2 melting temperature [TmCO2] = -56.3 to
-54.5°C) for early alteration zones and saline (2.1 to 27.5 wt % NaClequiv., Tht = 153.3 to 215.5°C) solutions for late alteration zones.
These data are inconclusive as regards the source of mineralising fluids involved in the evolution of the deposit which may involve: i). hypersaline fluids derived from hydrothermal-magmatic brines or evaporative brines/evaporite-derived fluids, ii). saline solutions generated by progressive dilution of primary hypersaline fluids with shallower, colder and less saline fluids (e.g., meteoric, seawater), and iii). carbonic components sourced from magmatic fluids, devolatilization of carbonate units and, partially, metamorphic fluids.
Sulphur is interpreted to have been leached from country rocks in the earlier hydrothermal stages (mineralization I, δ34S = 0.01 to 1.99‰), and to have come from mixed granitic and external oxidised sources for later
overprinting events (mineralisation II, δ34S = 9.75 to 11.25‰). These data are interpreted to provide evidence for the recurrence of hydrothermal activity, indicating the existence of different potential sources of metals and sulphur during the evolution of the deposit.
Reserves and Resources
No resource estimates have been located for the Igarapé Cinzento deposit/prospect. Mineralisation appears to occur as a number of steeply dipping bodies with widths of a few tens of metres and vertical extent of a few hundred metres.
The most recent source geological information used to prepare this decription was dated: 2019.
This description is a summary from published sources, the chief of which are listed below. © Copyright Porter GeoConsultancy Pty Ltd. Unauthorised copying, reproduction, storage or dissemination prohibited.
|
|
Toledo, P., Moreto, C.P.N., Xavier, R.P., Gao, J., Matos, J.H. and Melo, G.H.C., 2019 - Multistage Evolution of the Neoarchean (ca. 2.7 Ga) Igarape Cinzento (GT-46) Iron Oxide Copper-Gold Deposit, Cinzento Shear Zone, Carajas Province, Brazil: in Econ. Geol. v.114, pp. 1-34.
|
Porter GeoConsultancy Pty Ltd (PorterGeo) provides access to this database at no charge. It is largely based on scientific papers and reports in the public domain, and was current when the sources consulted were published. While PorterGeo endeavour to ensure the information was accurate at the time of compilation and subsequent updating, PorterGeo, its employees and servants: i). do not warrant, or make any representation regarding the use, or results of the use of the information contained herein as to its correctness, accuracy, currency, or otherwise; and ii). expressly disclaim all liability or responsibility to any person using the information or conclusions contained herein.
|
Top | Search Again | PGC Home | Terms & Conditions
|
|