Carmacks - Williams Creek, Stu, Carmacks North |
|
Yukon Territory, Canada |
Main commodities:
Cu Au Ag
|
|
 |
|
 |
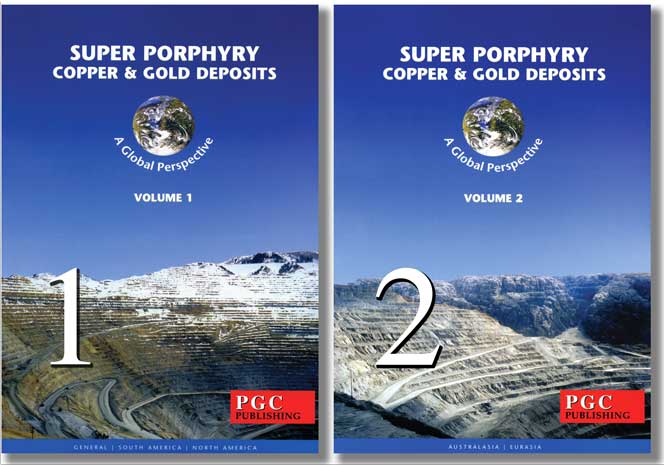 |
Super Porphyry Cu and Au

|
IOCG Deposits - 70 papers
|
All papers now Open Access.
Available as Full Text for direct download or on request. |
|
 |
The Carmacks (previously known as the Williams Creek) copper deposit is located near the 25 km NNW of the town of Carmacks and 195 km NNW of Whitehorse in the Yukon Territory, northwestern Canada (#Location: 62° 20' 24"N, 136° 41' 59"W).
The Stu or Carmacks North Prospect is located 6 km NW of the Carmacks Main Zone, and is described below (#Location: 62° 23' 50"N, 136° 49' 7"W).
Copper occurrences were first reported in the region in 1887 at Hoochekoo Bluff, ~12 km north of the the Carmacks deposit, on the Yukon River. In 1898 the first claims were pegged to cover copper bearing quartz veins at Williams Creek and Merrice Creek Canyons, east of the present Carmacks copper deposit. The discovery of the Casino porphyry copper deposit, 104 km to the NW, in the 1960s precipitated interest in the area and pegging of the Williams Creek claims in 1970. The Dawson Range Joint Venture optioned the property and during a reconnaissance program of mapping and geochemical sampling managed by Archer, Cathro and Associates, located the No. 1 and 2 Zones (as described below). The property was purchased by a consortium that became Western Copper Holdings Ltd. in 1996. A full and positive feasibility study for an open pit mine and heap leach to recover copper metal by solvent extraction/electro-winning was completed in 1994. However, in 1998, after completing some pre-construction preparatory work on site, the project was suspended indefinitely due to low copper prices. In 2004 after prompting from the Yukon Government, Western Copper decided to resurrect the project. Over the ensuing years studies were conducted and changes in the corporate structure and partners in the project changed, including Copper North Mining Corp, until in 2020, Granite Creek Copper Ltd., the owner of the Stu Project, ~7 km to the NW, gained control of the project. The aim is to consolidate further exploration and any potential production in the southern half of the Carmacks-Minto copper belt defined by Carmacks, Stu, Minto and other similar prospects that are distributed over a NW-SE trending, 75 km long interval.
Regional Setting
During the Late Triassic to Jurassic, the Intermontane terranes of Yukon-Tanana, Stikinia, Quesnellia and Cache Creek were accreted to the western margin of North America, resulting in prolific arc magmatism throughout the northern Cordillera (Nelson et al., 2013). This period of magmatism was responsible for the formation of many of the porphyry Cu ± Au, Mo deposits to the south of Yukon, in British Columbia (Logan and Mihalynuk, 2014).
Late Triassic to Early Jurassic magmatism in the Yukon Territory involved the building of a Late Triassic island arc, comprising the Lewes River Group and Stikine Plutonic Suite, that subsequently underwent arc-continent collision with the continent to the east, syncollisional magmatism and exhumation (Nelson et al., 2013). The Lewes River Group volcanic suite and the Minto suite intrusions extends northward into central Yukon where it is terminated, continuing as plutonic equivalents, in the form of the Stikine and Pyroxene Mountain plutonic suites that extend farther northwest into east-central Alaska (Ryan et al., 2013; Dusel-Bacon et al., 2015; Colpron et al., 2016).
The Late Triassic 217 to 214 Ma Stikine suite occurs as a series of small plutons intruding Upper Triassic arc volcanic rocks of Stikinia and Palaeozoic metasedimentary and meta-igneous rocks of the Yukon-Tanana Terrane in south-central Yukon (Sack et al., 2020).
The Upper Triassic to Lower Jurassic 205 to 194 Ma Minto suite is as a series of large plutons intruding the Lewes River Group and the Yukon-Tanana terrane, interpreted to represent syncollisional magmatism at the onset of arc accretion. The younger 188 to 183 Ma Long Lake and 178 to 168 Ma Bennett- Bryde plutonic suites represent ongoing syncollisional magmatism durin the Lower to Middle Jurassic (Colpron et al., 2015, 2016b; Sack et al., 2020).
The Carmacks deposit lies within the composite 120 x 15 to 25 km Lower Jurassic Granite Mountain Batholith section of a series of Early Jurassic plutons, segmented by Upper Cretaceous and younger volcanic cover. The eastern Granite Mountain batholith belongs to the Minto suite, whilst its western part belongs to the Long Lake suite (Colpron et al., 2016; Sack et al., 2020). The batholith intrudes and obscures the contact between mid-Palaeozoic rocks of the Yukon-Tanana Terrane and Upper Triassic rocks of Stikinia to the west and east respectively. To the east of the batholith, Stikinia arc rocks include volcanic and sedimentary facies and subvolcanic intrusions of the Upper Triassic Povoas Formation, which belongs to the Lewes River Group (Tempelman-Kluit, 1984, 2009; Hart, 1997). In southern Yukon, the Povoas Formation is characterised by variably deformed and subgreenschist to locally amphibolite facies augite porphyritic basalt, volcaniclastic rocks and hornblende gabbro (Hart and Radloff, 1990). This formation is separated from the batholith by the dextral-normal oblique-slip Hoocheekoo fault (Tempelman-Kluit, 1984, 2009). However, sliver-like inliers of similar metamorphosed mafic to intermediate rock are found within the Granite Mountain Batholith hosting Cu-Au-Ag mineralisation at the Carmacks Copper deposit, Minto mine and Stu prospect (Kovacs et al., 2020).
The Late Triassic to Early Jurassic batholiths were emplaced into crust that was being uplifted and exhumed in the Early to Middle Jurassic on the flanks of the subsiding marine Whitehourse Trough (Colpron et al., 2015). Exhumation was near completion in the mid-Cretaceous, when the batholith and enclosed sequences were unconformably overlain by volcanic rocks of the Mount Nansen Group, which are exposed 40 km to the SW of the Carmacks Copper deposit. These were overlain by further volcanic rocks of the Upper Cretaceous Carmacks Group that are preserved as extensive blankets north and south of the Granite Mountain batholith, and as isolated erosional remnants within the batholith (Kovacs et al., 2020).
The Carmacks deposit is near the northwestern limit of Pleistocene glaciation (Duk-Rodkin, 1996), such that glacial erosion was restricted to subalpine areas and bedrock below discontinuous till preserved a deep oxide weathering profile. Palaeoweathering profiles containing copper oxide minerals at the deposit are locally capped by Carmacks Group volcanic rocks, showing that at least part of the oxidation history is Late Cretaceous or older (Kovacs et al., 2020).
Geology
The Carmacks Copper deposit occurs within a series of NNW-trending elongate inliers of amphibolite facies mafic to intermediate meta-igneous rocks and their migmatitic derivatives enclosed within the generally massive granitoids of the Granite Mountain batholith. These mafic rocks include foliated, equigranular amphibolite that is locally texturally transitional with less foliated, hornblende-porphyroblastic amphibolite, whilst rare augite gabbro is also locally mapped. The mafic rocks are interlayered with quartz-plagioclase-biotite schist. There is a texturally transition between the metamorphic and migmatitic rocks, with the latter hosting the bulk of hypogene copper mineralisation. Migmatitic rocks preferentially occur along the eastern flank of the largest, 3 km by 20 to 100 m wide inlier, where they occur along a transitional intrusive contact between metamorphic rocks and the Granite Mountain batholith (Kovaks et al., 2020).
To the east of the main deposit an earlier phase of the Granite Mountain Batholith is represented by a mainly monzodioritic intrusive which appear to be responsible for the partial melting of the metamorphic rocks and formation of migmatite. A subsequent phase to the west includes K feldspar megacrystic granodiorite, quartz diorite, granite and syenite. The absence of migmatitic rocks along the contact with this second intrusive phase indicates its emplacement did not lead to further migmatisation, suggesting its temperature was insufficient to induce partial melting. The batholith phases are typically undeformed, although alignment of phenocrysts locally defines a weak magmatic foliation. Barren dykes of K feldspar porphyritic granodiorite, quartz monzonite, quartz monzodiorite, granite pegmatite and aplite crosscut the metamorphic host rocks, the mineralisation and other massive intrusive phases and are variably overprinted in the metamorphic rocks by folding and boudinage (Kovaks et al., 2017; 2020). Post-mineralisation granitic pegmatite and aplite dykes are widespread in the area and range from a few centimetres to approximately three metres in thickness (Doerksen et al., 2016).
Inliers of metamorphic rocks within the batholith are composed of a range of meta-igneous rock types. Augite gabbro is massive to locally foliated and is composed of 40 to 45% plagioclase; 30 to 35% clinopyroxene; 20 to 25% hornblende and accessory titanite. It is transitional with hornblende porphyroblastic amphibolite, which comprises 60% plagioclase; 30 to 35% hornblende porphyroblasts that replaced clinopyroxene; 5% biotite and accessory titanite. Melanocratic amphibolites consist of up to 75% hornblende, whilst foliated, equigranular amphibolite typically has 60 to 65% plagioclase feldspar; 25 to 35% hornblende; 10 to 15% biotite; minor quartz (5%) and accessory titanite. Biotite defines a penetrative foliation within these rocks (Kovacs et al., 2020).
The amphibolitic rocks are interlayered with brown-weathering schist that contains 10 to 12% quartz; 70 to 80% plagioclase; 20 to 25% biotite; ~1% titanite and accessory zircon which have locally been recrystallised to a granoblastic texture. These interlayered lithofacies have a gradational contact with texturally and compositionally variable migmatitic rocks, which Kovacs et al. (2020) divide into metatexites and diatexites.
The metatexite is predominantly composed of fine-grained, foliated to granoblastic and stromatically layered quartz-plagioclase-biotite paleosome, and subordinate quartzo-feldspathic leucosome, which occurs as irregular pods and as foliation-parallel layers that locally define mesoscopic folds. It has a bulk mineralogy composed of 50 to 70% plagioclase; 7 to 10% quartz; 7 to 15% biotite and ~2 to 5% K feldspar. Rare medium-grained, euhedral garnet and subhedral orthopyroxene occur locally at the margins of the leucosome (Kovacs et al., 2020).
In contrast, the diatexite is dominated by predominantly massive, compositionally and mineralogically homogenous neosome which contains a large proportion of massive leucosome, with lesser proportions of mm- to metre-scale melanosome interpreted to be schlieren of residual metamorphic protolith. The leucosome is medium grained and composed of 45 to 60% plagioclase; 15 to 25% hornblende; 5 to 10% biotite; 3 to 5% clinopyroxene; 1 to 2% quartz; 1% epidote and accessory titanite and apatite. Plagioclase is typically albitic and forms medium anhedral grains that are typically mantled by intergrowths of quartz, feldspar and biotite that represent late-stage melt. Hornblende and clinopyroxene are medium grained and embayed, indicative of partial consumption by melt-forming reactions (Holyoke and Rushmer, 2002; Sawyer, 2008). Melanosome typically contains 80% hornblende; 7 to 10% biotite; 10% calcite; 2 to 4% K-feldspar; and 1 to 2% quartz. Hornblende has embayed oikocrysts with chadacrysts of biotite and quartz. Fine-grained quartzofeldspathic intergrowths occupy interstitial space between hornblende grains and represent late-stage melt (Kovacs et al., 2020).
NOTE: Much of the mineralogic descriptions above a quoted verbatim from Kovacs et al. (2020) as summarising, rewording or paraphrasing is impractical. As an alternate to this more mineralogic description, Doerksen et al. (2016) describes the hosts as follows.
The host rocks to the hypogene mineralisation is typically a dark grey to black hornblende-biotite amphibolite with a pervasive foliation. It varies from massive to a facies containing relict hornblende phenocrysts after pyroxene, possibly representing a variation in the interpreted volcanic, protolith. The amphibolite is locally more gneissic reflected in mineralogical and colour segregations, with the amount of mafic minerals varying from ~50 to ~100%. Locally, the penetrative fabric is absent, having apparently been recrystallised to microdiorite by the heat of the adjacent granodiorite intrusion. Sulphide mineralisation within the amphibolite typically follows the foliation, with some discordant sulphide veinlets. Sulphide mineralisation is also hosted by diorite where chalcopyrite and bornite occur interstitially between hornblende crystals as a net-texture.
Structure
The metamorphic rocks preserve a penetrative transposition fabric (S1), defined by the preferred orientation of hornblende and biotite in amphibolite and schist, respectively, or by the leucrocratic and melanocratic layering of migmatitic rocks. S1 pre-dates the emplacement of the Granite Mountain batholith. The felsic plutonic rocks of the Granite Mountain batholith are generally unfoliated, except for a weak tectonic foliation proximal to contacts with metamorphic inliers which implies D2 outlasted emplacement and crystallisation of the batholith. Within the schists, D2 is expressed as upright, NNW trending, tight to isoclinal F2 folds with subhorizontal fold axe. These F2 folds become increasingly disharmonic and rootless in migmatitic rocks. A subvertical, generally NNW aligned crenulation cleavage is locally developed in in F2 fold hinges in schist, whilst a composite S1/S2 fabric is developed along F2 fold limbs (Kovacs et al., 2020).
Deformation increases toward the mineralised zones, suggesting an underlying structure may be a control on mineralisation (Doerksen et al., 2016).
Mineralisation
Mineralisation at the Carmacks Copper Cu-Au-Ag deposit defines an almost 3 km long, NNW trending corridor that includes the Main zone in the north, and the 2000S, 12 and 13 zones progressively to the south. The Main zone comprises zones 1, 4, 7 and 7A, which collectively account for the bulk of the measured and indicated resources. Zones 6 and 8 are 750 m to the SW of the Main Zone (Doerksen et al., 2016).
The character of the deposit varies along strike, resulting to a division into a northern and a southern half. The northern has a more regular thickness, dip angle, length and down dip characteristics, whilst the southern half splays into irregular intercalations in Zones 7 and 7A, and is terminated against sub-parallel faults down dip. Zones 12 and 13 are 1.2 km to the south of Zone 1 and occur over a strike length of 1.2 km and up to 100 m in width. They are hosted by less mafic amphibolite and gneisses than those of Zone 1, and are highly silicified and K feldspar altered (Doerksen et al., 2016).
Deep oxidation of the deposit has produced an oxide cap that can be >200 m thick in Zones 1, 4, 7 and 7A, whilst in Zones 13 and 12 the oxide cap can be as thin as ~40 m. The upper 250 m of the Zone 1 is oxidised. The bulk of the copper found in oxide sections of the No.s 1, 4, 7 and 7A Sub-zones occur as the secondary minerals malachite, cuprite, azurite and tenorite with very minor other secondary copper minerals including covellite, digenite and djurlite. Native copper occurs as dendritic secondary precipitates on fractures, disseminated grains or thin veinlets. Other secondary minerals include limonite, goethite, specular hematite and gypsum. Carbonates occurrence is pervasive, or as irregular patches or microveinlets. It is not common but of the order of 1% of the rock, where present. Within the oxide zone, pyrite is virtually absent and pyrrhotite has been completely leached. Weathering has produced 1 to 3% open space rendering the rock permeable. Secondary Cu and Fe minerals line and fill these cavities, forming both irregular and coliform masses that infill fractures, and rim sulphides. Gold, which is rarely >5 µm in size, occurs as native grains, most commonly in cavities with limonite or in limonite adjacent to sulphides, but also in malachite, plagioclase, chlorite and rarely in quartz grains. Gold grades are higher in the northern half of the deposit, where they average 0.75 g/t compared to 0.27 g/t Au in the southern half, and unlike copper there is no apparent increase in grade with depth. The highest grade gold is not associated with the highest copper values, although gold values in the northern half increase toward the footwall (Doerksen et al., 2016).
Secondary copper mineralisation does not appear to be restricted to particular rock types, a result of the remobilisation of copper during supergene processes. In the northern half of Zone 1, oxide copper mineralisation forms high and low grade intervals that are reasonably consistent both along strike and down dip and cross lithologic boundaries, although higher grades tend to form closer to the footwall section, with lower grades in the hanging wall half. However, oxide copper grades do increase with depth in both the footwall and hanging wall within this zone as a whole (Doerksen et al., 2016).
In contrast to the oxide ores, hypogene copper mineralisation is apparently primarily associated with amphibolite gneiss units and early-formed diorites (Doerksen et al., 2016), mainly hosted by the feldspar-amphibole schist, quartz-plagioclase-biotite schist, and migmatitic rocks (Kovaks et al., 2017). Primary mineralisation, below the zone of oxidation comprises mainly chalcopyrite, bornite, molybdenite, magnetite, pyrite and pyrrhotite (Doerksen et al., 2016). Chalcopyrite and bornite are the main sulphide minerals, whereas pyrite is notably low. Locally, molybdenite is present in high concentrations in migmatite, but is sparse at the deposit scale. Magnetite is common in the western part of the deposit but minor elsewhere (Kovaks et al., 2017). Primary copper mineralisation appears to be zoned from bornite in the north to chalcopyrite, and finally to minor pyrite-pyrrhotite in the south. Narrow veinlets of anhydride were found in the deepest drill hole. Other primary minerals include magnetite, gold, molybdenite, native bismuth, bismuthinite, arsenopyrite, pyrite, pyrrhotite and carbonate. Of these molybdenite, visible gold, native bismuth, bismuthinite and arsenopyrite only occur rarely. These hypogene sulphides and magnetite occur as foliation parallel stringers, narrow massive sulphide bands, net textured accumulations, bands of heavy disseminations or less frequently disseminations within the host rocks (Doerksen et al., 2016). Chalcopyrite-dominant stringers and blebs, intergrown with subordinate bornite and trace pyrite, parallel S1 foliation in amphibolite and quartz-plagioclase-biotite schist (Kovaks et al., 2020). Where hosted in schistose rocks, copper mineralisation occurs as chalcopyrite dominant stringers that parallel the dominant S1 foliation and generally occupies 1 to 2% of the rock volume. Chalcopyrite occurs as elongate, foliation-parallel blebs of up to 1 mm with much less abundant bornite. In contrast, mineralisation hosted by migmatitic rocks, particularly diatexite migmatite occurs as net-textured intergrowths of bornite and chalcopyrite that occupy the interstices of generally fresh, magmatic silicate grains. In this net-textured mineralisation, especially in the melanosome, copper sulphides are up to 20% of the rock by volume and include bornite and lesser chalcopyrite comprising 15 and 4 to 5% respectively, with the two sulphides occurring together as aggregates of up to 2 to 4 mm across that are clearly interstitial with respect to silicate grains. A few grains of bornite contain abundant exsolution blebs of chalcopyrite. Chalcopyrite generally forms anhedral patches that are 1 to 1.8 mm across, intimately intergrown with bornite. Both chalcopyrite and bornite are commonly replaced by digenite along 50 mm wide fractures and along grain margins. Hematite forms irregular, narrow (10 mm), patches along fractures and the margins of bornite-chalcopyrite-digenite. Chalcocite forms fine-grained, prismatic needles that occur at bornite-chalcopyrite grain boundaries (Kovaks et al., 2017). Disseminated chalcopyrite and pyrite are only a minor component of the hypogene mineralisation, occurring in more massive rocks without an obvious S1 foliation, although it also occurs in the leucosome of metatexite (Kovaks et al., 2020).
Kovaks et al., 2017 conclude that, as the mineralisation at Carmacks is hosted by metamorphic rocks and is S1-parallel, the copper mineralisation within the schists (Cu1) was deposited in the protolith prior to D1 deformation. However, net-textured mineralisation (Cu2) is restricted to migmatitic host rocks, which requires crystallisation of igneous silicates from a silicate melt, followed by precipitation of bornite and chalcopyrite from an immiscible sulphide liquid. They interpret the previously mineralised (Cu1) and deformed (D1) rocks to have been heated to partial melting conditions in the thermal aureole of the earlier eastern phase of the Granite Mountain Batholith, in which copper and trace metals entered a mobile sulphide liquid phase that was re-precipitated as sulphides on cooling. The net-textured Cu2 sulphides mantle unaltered plagioclase and hornblende in migmatitic rocks. If this mineralisation were introduced via hydrothermal fluids, the plagioclase and mafic mineral phases would be expected to have been altered and Fe-bearing silicates replaced by sulphide minerals. The ore mineral distribution broadly reflects a western chalcopyrite zone, related to the metamorphic host rocks, and an eastern bornite-chalcopyrite-digenite zone that correlates with migmatitic host rocks.
Geochronologic constraints indicate the Cu1 hypogene mineralisation occurred between ~217 and 213 Ma, prior to metamorphism in the latest Triassic at ~206 Ma and batholith emplacement in the Lower Jurassic at ~200 to 194 Ma. Deformation, metamorphism and tectonic burial of the deposits occurred during imbrication of the Intermontane terranes in the latest Triassic and earliest Jurassic from ~205 to 195 Ma which overlapped batholithic intrusion. This intrusion and deep burial resulted in anatectic melting of the large, mineralised xenoliths at midcrustal depths at ~197 to 196 Ma. Sulphide and silicate melting took place at ~600 to 750°C (Kovaks et al., 2020).
Disseminated Cu1 chalcopyrite and pyrrhotite in amphibolite within the deposit are characterised by a narrow S isotope ranges of -0.60 to +0.65‰ (Pearson, 1977; Tafti, 2005), which is interpreted to be consistent with a magmatic source for the mineralising fluids (Wilson et al., 2007). The proto deposit has been assumed to have been porphyry-style (Kovaks et al., 2020), albeit hosted by a mafic sequence.
Reserves and Resources
Published Mineral Resources in January 2016 were (Copper North Mining Corp, NI 43-101 Tech Rept.):
Oxide + Transitional Mineralisation
Measured + Indicated Mineral Reources - 15.690 Mt @ 0.94% Cu Total, 0.74% Cu Soluble, 0.20% Cu sulphide, 0.38 g/t Au, 3.97 g/t Ag.
Inferred Mineral Reources - 0.913 Mt @ 0.45% Cu Total, 0.30% Cu Soluble, 0.15% Cu sulphide, 0.12 g/t Au, 1.90 g/t Ag;
Sulphide Mineralisation
Measured + Indicated Mineral Reources - 8.068 Mt @ 0.68% Cu Total, 0.05% Cu Soluble, 0.65% Cu sulphide, 0.18 g/t Au, 2.33 g/t Ag;
Inferred Mineral Reources - 8.407 Mt @ 0.63% Cu Total, 0.03% Cu Soluble, 0.61% Cu sulphide, 0.15 g/t Au, 1.99 g/t Ag;
Published Ore Reserves in October 2012 were (Copper North Mining Corp, NI 43-101 Tech Rept.):
Proved + Probable Ore Reserves - 11.551 Mt @ 0.977% Cu Total, 0.805% Cu Soluble, 0.172% Cu sulphide, 0.435 g/t Au, 4.34 g/t Ag.
In addition to the references listed below, this record has in part been drawn from: Doerksen, G., McLeod, K., Arseneau, G., Dreisinger, D., Anstey, D., Esford, F. and Hester, M.G., 2016 - Preliminary economic assessment technical report on the Carmacks Project, Yukon, Canada; an NI 43-101 Technical report prepared for Copper North Mining Corp., 227p.
Stu or Carmacks North
Like Carmacks, the Stu prospect is also hosted within the Granite Mountain Batholith. Within the deposit area, two main phases have been recognised, namely: i). weakly foliated to unfoliated, fine to medium-grained, grey-white to grey, equigranular porphyritic granodiorite with 5 to 15% K feldspar phenocrysts; this phase underlies most of the prospect area and surrounds; and ii). an unfoliated equigranular quartz monzonite found to the SW. The geology and geophysical grain generally trend NW, parallel to the boundary between the two intrusive phases which occurs in the transition between a relative magnetic low, to the SW, and a high to the NE (Sack et al., 2016).
Within the porphyritic granodiorite there are a series of strongly foliated bodies that vary from unfoliated granodiorite to biotite schist and biotite orthogneiss, with the latter being more common than schist. The foliation within these bodies strikes NW and dips at ~75°NE, although the bodies themselves dip more shallowly at between 40 and 70°NE. The contacts between the granodiorite, biotite schist and biotite orthogneiss is generally transitional over several cm, whilst locally, K feldspar phenocrysts bearing unfoliated granodiorite impinge upon foliated rocks in a fashion suggesting that the contact is primarily intrusive. This, in conjunction with the overall rock distribution of the rocks suggest the unfoliated granodiorite engulfed earlier foliated varieties. Other less extensive intrusive lithologies locally recognised include aplite, pegmatite, microdiorite and andesite dykes. Aplite and pegmatite dykes that are generally <1 m thick occur as NW striking, steeply dipping swarms, found proximal to contacts between foliated and unfoliated varieties of granodiorite, suggesting these contacts are zones of weakness. These dykes are mineralogically and compositionally similar to the quartz monzonitic intrusive phase. Crosscutting relationships suggest the intrusive succession was granodioritic orthogneiss → unfoliated granodiorite → quartz monzonite → aplite/pegmatite → microdiorite → andesite dykes. Locally outcrops of the Upper Cretaceous Carmacks Group volcanics overlie and related mafic intrusions intrude the other rock types (Sack et al., 2016).
Cu, Au ± Ag mineralisation is mostly associated with the biotite schist and orthogneiss of these strongly foliated bodies, though locally supergene copper minerals are found in adjacent unfoliated granodiorite. Mineralisation appears to primarily occur within multiple discrete bodies of foliated granodioritic rocks that have been intruded and isolated as rafts/xenoliths within the unfoliated granodiorite and quartz monzonite. Mineralisation occurs as both hypogene sulphide minerals, chalcopyrite and bornite, and in the oxide zone as supergene assemblage that is dominantly malachite, with lesser tenorite, chalcocite, azurite, chrysocolla and possible brochantite. The supergene minerals display textures indicative of transport and open space fill. Where hypogene copper sulphides occur within the foliated granodiorite and gneiss they replace mafic minerals. The highest gold and silver values are associated with bornite-rich sections. Magnetite is locally abundant in both mineralised and barren rock.
Grades at Stu average 0.2 to 0.6% Cu (Sack et al., 2020).
In addition to the references listed below, this record has in part been drawn from: James, D. and Davidson, G.S., 2018 - Technical Report on the Stu Copper Property Near Carmacks, Yukon, CANADA; an NI 43-101 Technical report prepared for Granite Creek Copper Ltd., 114p.
Return to Top
The most recent source geological information used to prepare this decription was dated: 2020.
This description is a summary from published sources, the chief of which are listed below. © Copyright Porter GeoConsultancy Pty Ltd. Unauthorised copying, reproduction, storage or dissemination prohibited.
|
|
|
Kovacs, N., Allan, M.M., Crowley, J.L., Colpron, M., Hart, C.J.R., Zagorevski, A. and Creaser, R.A., 2020 - Carmacks Copper Cu-Au-Ag Deposit: Mineralization and Postore Migmatization of a Stikine Arc Porphyry Copper System in Yukon, Canada: in Econ. Geol. v.115, pp. 1413-1442.
|
Kovacs, N., Allan, M.M., Zagorevski, A., Milton, J.E. and Hart, C.J.R., 2017 - New geological insights into the Carmacks Copper Cu-Au-Ag deposit, central Yukon: in MacFarlane, K.E. and Weston, L.H. (Eds.), 2017 Yukon Exploration and Geology 2016, Proceedings, Yukon MINFILE 115I 008, pp. 117-140.
|
Sack, P.J., Casselman, S., James, D. and Harris, B., 2016 - Copper-gold ± silver mineralization at the Stu occurrence, central Yukon: in MacFarlane, K.E. and Nordling, M.G. (Eds.), 2016 Yukon Exploration and Geology, Yukon Geological Survey, Yukon MINFILE 115I 011, pp. 207-222.
|
Porter GeoConsultancy Pty Ltd (PorterGeo) provides access to this database at no charge. It is largely based on scientific papers and reports in the public domain, and was current when the sources consulted were published. While PorterGeo endeavour to ensure the information was accurate at the time of compilation and subsequent updating, PorterGeo, its employees and servants: i). do not warrant, or make any representation regarding the use, or results of the use of the information contained herein as to its correctness, accuracy, currency, or otherwise; and ii). expressly disclaim all liability or responsibility to any person using the information or conclusions contained herein.
|
Top | Search Again | PGC Home | Terms & Conditions
|
|