Karlawinda - Bibra, Francopan |
|
Western Australia, WA, Australia |
Main commodities:
Au
|
|
 |
|
 |
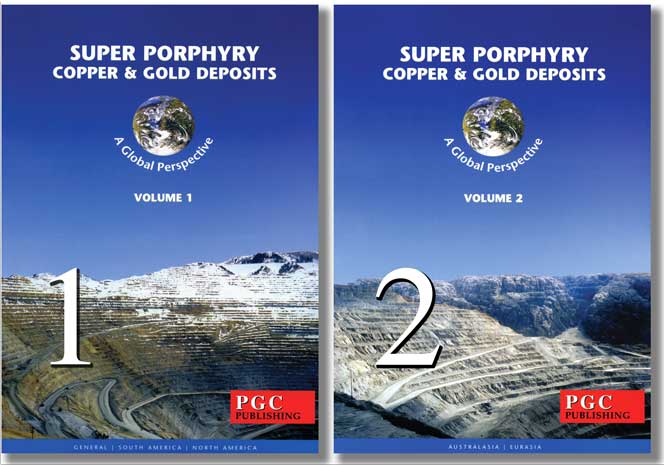 |
Super Porphyry Cu and Au

|
IOCG Deposits - 70 papers
|
All papers now Open Access.
Available as Full Text for direct download or on request. |
|
 |
The Karlawinda gold project, which principally comprises the Bibra and Francopan deposits, is located in the Sylvania Inlier of the Pilbara Craton in Western Australia, 58 km SE of the town of Newman (#Location - Bibra: 23° 45' 40"S, 120° 6' 38"E).
Gold mineralisation was originally discovered by WMC Resources Ltd at the Francopan Prospect in 2005. The project was subsequently acquired by Independence Group (IGO) in 2008 who discovered the significant Bibra Gold Deposit in 2009. Bibra is largely exposed on the southern margin of the Archaean Sylvania Inlier, but plunges to the SE below Palaeoproterozoic Bangemall Basin cover. The Francopan deposit, located 5 km to the ESE, is predominantly covered by the south dipping Palaoeproterozoic sedimentary sequence, which comprises shales and carbonates and intrusive gabbro. In 2016 Capricorn Metals Ltd purchased the Karlawinda Gold project from IGO, and conducted additional drilling to expand the resource. A feasibility study was completed in 2017 and construction commenced on an open pit mine at Bibra in 2019, with first production in mid 2021. The Francopan deposit contains higher grade zones and may potentially be exploited by underground mining (Capricorn Metals).
Regional Setting
The Pilbara Craton is an Archaean granite-greenstone terrane in Western Australia that rests on an unexposed 3.80 to 3.55 Ga basement in the east. It was mainly formed from ~3.53 Ga from two main main elements, the East Pilbara Terrane and West Pilbara Superterrane. The former is the ancient nucleus of the craton, and was overlain by a volcanic plateau between 3.53 and 3.22 Ga, the result of multiple mantle plume events. Hickman (2012) suggest that between 3.22 and 3.16 Ga, rifting of the East Pilbara Terrane split off two terranes, the Karratha and Kurrana to the west and east respectively, separated from the main core by intervening basins floored by oceanic crust. These two NE-SW trending basins, the Mosquito Creek and Mallina basins were filled with immature sediments. The ~3.07 to 2.83 Ga Prinsep Orogeny ensued, initially involving a further series of mainly extensional rift basins and granitic supersuites of the 3.05 to 2.93 Ga de Grey Superbasin, which unconformably overlies the five distinct terranes that make up the Pilbara Craton. This was followed by craton-wide compressional deformation between 2.95 and 2.93 Ga, culminating in the final accretion of the various terranes at 2.91 Ga. Post-tectonic granitic intrusions between 2.89 and 2.83 Ga completed the final cratonisation of the Pilbara Craton (Van Kranendonk et al., 2007; Hickman, 2012). The southern half of the Craton is unconformably overlain by the Late Archaean to Palaeoproterozoic 2.78 to 2.43 Ga Fortescue, Hamersley and Turee Creek Groups in their respective basins, which include rift volcanics, banded iron formations and metasedimentary rocks that cover ~70% of the craton (Cawood and Tyler, 2004). These basins represent a rift to passive margin regime.
The east-west elongated, 145 x 25 to 45 km, 5600 km2 Sylvania Inlier is exposed below the southeastern margin of the Hamersley Basin, whilst 1.40 to 1.07 Ga Collier Group deltaic to deep-marine facies sedimentary rocks of the Bangemall Basin onlap its southern and eastern margins. To the west it is overlain by Palaeoproterozoic conglomerate with interbedded fine sandstone and mudstone of the Bresnahan Basin that is younger than the Hamersley Group, but older than the Bangemall Basin sequence. The Sylvania Inlier is the southernmost granite-greenstone block of the Pilbara Craton, and the only such terrane to be exposed south of the Hamersley Basin. However, a comparison of the rare earth element and isotope systematics of granitoids in the Sylvania Inlier and those over the bulk of the northern Pilbara, north of the Hamersley Basin, found no similarities (Tyler et al., 1992). This may be explained by the inferred correlation of the Sylvania Inlier with the Kurrana Terrane that was rifted from the eastern margin of the East Pilbara Terrane and is only exposed as a very limited inlier in the eastern extremity of the northern Pilbara.
The Sylvania Inlier is interpreted to be an autochthonous to parautochthonous block occupying an anticlinal fold core in the west, and a stack of parautochthonous to allochthonous thrust sheets in the east. This geometry is thought to have formed in response to the Palaeoproterozoic collision between the Yilgarn and Pilbara cratons. It lies within the northeastern Capricorn Orogen, a 1000 km long by 500 km wide, ESE trending region of variably deformed meta-igneous, metasedimentary and low grade sedimentary rocks that separates the two cratons. The Capricorn Orogen began to form at ~2.21 Ga with the progressive amalgamation of the two cratons and the exotic Glenburgh Terrane of the Gascoyne Province in the west, to form the West Australian Craton which was completed by 1.95 Ga. This was followed by subsequent intra-cratonic reworking which persisted until 1.03 Ga, punctuated by a series of major tectono-thermal events affecting the cratons and the pre-collisional basins on their margins.
Around two thirds of the Sylvania Inlier is composed of ~2793 Ma granites, whilst the remainder comprises 3500 to 3450 Ma greenstone successions, and dykes of ultramafic rocks, gabbro, anorthosite and ~3450 Ma monzonite (Tyler 1990, 1991; Hollingsworth et al., 2001). Variably foliated granite to granodiorite intrusives are found in the eastern two thirds, as are most of the greenstone succession, whilst foliated porphyritic granite and foliated, gneissic or migmatitic biotite monzogranite, granodiorite and tonalite compositions, as well as lesser greenstones, outcrop in the western third (Bagas et al., 2008). The greenstone successions are only a minor component, which, with mafic intrusions, younger granites and ultramafic rocks, including dunite and serpentinite, are discontinuously developed within the dominant granite background (Bagas et al., 2008). All primary fabrics within these rocks have been obliterated (Thorne and Trendall, 2001). The folded >25 km long Jimblebar greenstone belt in the northeastern part of the inlier is the largest and most coherent greenstone belt and is cut by weakly deformed equigranular granites, which are in turn cut by younger mafic dykes (S. Johnson, quoted by Ferguson 2013). The Karlawinda greenstone belt is on the south-central margin of the inlier. The greenstone belts generally comprise ultramafic to intermediate volcanic rocks, conglomerate, pelitic schist, quartzo-feldspathic schist, quartzite, chert and banded iron formation (Hollingsworth et al., 2001; Bagas et al., 2008). The granites contain 3481, 3023, 2912 and 2832 Ma xenocrystic zircons, whilst quartzite from the greenstone succession has detrital zircon ages of ~3665, 3612, 3600 and 3560 Ma (SHRIMP U–Pb Hollingsworth et al., 2001). One of the dolerite dykes cutting the greenstones has been dated at 2747 Ma (Wingate, 1999).
Geology and Mineralisation
The main deposit at Karlawinda is Bibra, which is hosted within an upper amphibolite facies-metamorphosed quartz-rich turbidite sequence of alternating Archaean amphibolites and garnet schists. The majority of the mineralisation is hosted within silicified and magnetite altered, mylonitised sandstone. The following main rock types have been identified (after Clow, 2010; Ferguson, 2013):
• Metasedimentary rocks after sandstone, siltstone and mudstone protliths, now predominantly metamorphic psammites, psammopelites and schists, differentiated by grain-size and dominant peak-metamorphic mineralogy. Porphyroblastic garnets are common in all three facies, but is less common where mineralised. Wackes are hornblende-dominated with subordinate quartz, feldspar, biotite and garnet. Siltstones and mudstones protoliths are represented in approximately equal proportions in the Bibra host package and are more abundant than both psammites and amphibolites. Wackes are much less common but are frequently associated with mineralisation. Petrographic studies (England, 2010) indicate peak metamorphic conditions reached lower amphibolite facies and that the protoliths were relatively rich in magnetite, ilmenite and apatite, with prominent, enigmatic monazite. The same study also revealed that textures of the light-coloured rocks are consistent with a plagioclase-rich arenite somewhat enriched in heavy minerals, but could also mimic a highly deformed, strongly recrystallised igneous rock. Pyrrhotite, magnetite and ilmenite in light-coloured samples is primary with retrograde pyrite, however, some coarse pyrite may have been present during peak metamorphism;
• Amphibolites, which alternate with the metasediments, are almost entirely composed of hornblende with very minor quartz and plagioclase. These are interpreted to represent metamorphosed dolerites. Individual units, and horizons within units, may be more quartz- and plagioclase-rich and also contain minor biotite and porphyroblastic garnets. The grain size varies from 1 to 4 mm, with the alignment of elongate hornblende crystals defining the foliation. Sharp contacts between metasediments and amphibolites have been interpreted to suggest the latter are metamorphosed igneous sills intruded into a semi-consolidated sedimentary pile, although foliation development may mask inhomogeneity or peperites related to magma injection into wet-sediments (Skilling et al., 2002).;
• Quartz-feldspar mylonite, which forms a high strain zone towards the centre of the metasedimentary units, subparallel to the east-west margin of the Sylvania Inlier. The majority of the mineralisation is hosted within silicified and magnetite altered sandstone that has been mylonitised to varying degrees;
• Granite, which dominates to the north of the deposit.
Where mineralised, the sandstones/schists are usually quartz-feldspar dominant, due to more abundant quartz, feldspar and hornblende-rich bands. They usually only contain minor or no biotite, chlorite or sericite, although some samples do have significant biotite-hornblende components (i.e. >10% each). They are fine- to medium-grained, with only minor foliation, which is distinguished by the alignment of tabular quartz-feldspar-hornblende-titanite and fine-grained, minor to continuous lepidoblastic biotite cleavage where biotite is present. Mineralised schists have large grain-size variations over short distances with a quartz -feldspar -biotite -carbonate -ilmenite -pyrrhotite -titanite ±hornblende peak metamorphic assemblage. Where hornblende is present, a weak gneissic foliation of quartz- to hornblende-rich bands occurs. Ilmenite, magnetite, hematite and titanite occur throughout the mineralised schist units, and there is an association between opaque minerals, carbonate and plagioclase.
Pyrite, pyrrhotite, chalcopyrite and magnetite are present in mineralised units. These include several generations of pyrite, including recrystallised diagenetic pyrite and at least two generations of hydrothermal pyrite. These sulphides occur as disseminations and in fractures and veins/veinlets. Pyrite is also found as post-foliation fracture fill and is mantled by chalcopyrite, and cored by pyrrhotite. Pyrrhotite is also commonly encased in quartz and feldspar and shielded from post-peak metamorphic retrogression. Pyrite and pyrrhotite have been deformed and dissembled during deformation, and appear to have flowed in a ductile manner in some fractures. Foliated veinlets include barren, subidioblastic quartz, carbonate, carbonate-opaque and fine 10 to 80µm quartz-carbonate-epidote-pyrite. Veinlets/fractures crosscutting foliation include carbonate and limonite-epidote-clay, with the latter being associated with sericite-clay alteration selvages increasing in intensity towards fractures (Ferguson 2013). Petrographic studies (England, 2010) of samples from Bibra suggest mineralisation was pre-peak metamorphism, while studies by Clow (2010) concluded that gold was introduced pre- or syn-peak metamorphism, and hosted within silicate minerals defining mineral lineations (Ferguson, 2013). Capricorn Metals (2020) report that geological logging suggests biotite, carbonate and magnetite alteration forms a halo surrounding the intense silica, pyrite and gold mineralisation. This assemblage may be the metamorphosed product of a lower grade primary suite of alteration minerals.
Primary gold mineralisation is associated with sulphides and deformed quartz-plagioclase veins that predominantly occur within poorly sorted sandstone units. It is largely present as inclusions on sulphide grain boundaries and within silicate minerals, as well as in solid solution in low-As to arsenian pyrite. However, the small size of gold inclusions, <40 µm, but mostly <10 µm, and their large influence on total gold content, when combined with gold grain size distribution, suggests the bulk of the gold at Bibra occurs as inclusions with only a minor contribution from gold remaining as solid solution in pyrite. The wall-rocks have been silicificied in mineralised horizons, as well as the addition of plagioclase, calcite, ankerite, pyrite, arsenopyrite, petzite (Ag3AuTe2) and other telluride minerals, electrum and native gold (Ferguson, 2013). Silicates and sulphides encapsulate ~75% of the gold-containing grains observed by Ferguson (2013), which include native gold (<20% Ag), electrum, solid solution gold in arsenian pyrite, microscopic inclusions in sulphide minerals, and gold-telluride minerals such as petzite (Ferguson, 2013).
The Bibra deposit has a strike and down dip length length each of >1.8 km and in 2021 was open open along strike and down dip. Gold is present as both laterite and oxide to primary mineralisation.
The laterite mineralisation occupies a surface area of ~1.25 x 1.15 km and occurs just below the surface as a pisolitic, lateritic, duricrust composed of maghemite, goethite and hematite.
This is underlain by oxide gold mineralisation, which is usually ~10 metres below surface, and is hosted in kaolin and smectite rich clays and persists to a depth of ~60 m.
The oxide to primary gold mineralisation has been developed in at least two parallel, NE-SW striking, 40 m thick sandstone units that form lodes dipping shallowly, at ~22°WNW. These two main primary lodes are known as the Main Footwall and Main Hanging wall. As such, the gold mineralisation is stratabound with lineations controlling higher-grade shoots. The lodes typically contain grades of ~0.9 g/t Au over their complete width, although high-grade shoots have developed parallel to the metamorphic fabric plunging to the WNW with a rod-like geometry. Down-plunge, the grades and thicknesses remain consistent whilst along strike they are more variable. The primary mineralisation in fresh rock is marked by 3 to 10% sulphides, subhedral magnetite grains, quartz veins/veinlets and fine-grained gold.
External to the main mineralisation, other more discrete lodes occur, including Port Rush, Easky, Finns and Easky East in the hanging wall to the main mineralisation, whilst to the south of the main Bibra pit area, mineralisation continues into the Southern Corridor and Tramore lodes (Capricorn Metals Limited website viewed June 2021).
Resources and Reserves
Open pit Mineral Resources as at April, 2020 (Capricorn Metals web page viewed June, 2020) were at a 0.3 g/t Au cut-off:
Bibra - Indicated + Inferred Mineral Resource - 62.3 Mt @ 0.8 g/t Au for 50.3 t of contained gold;
Southern Corridor - Indicated + Inferred Mineral Resource - 21.3 Mt @ 0.7 g/t Au for 14.8 t of contained gold;
Easky - Indicated + Inferred Mineral Resource - 3.1 Mt @ 0.5 g/t Au for 1.6 t of contained gold;
TOTAL - Indicated + Inferred Mineral Resource - 86.7 Mt @ 0.8 g/t Au for 66.7 t of contained gold;
Open pit Ore Reserves as at April, 2020 (Capricorn Metals web page viewed June, 2020) were at a 0.3 g/t Au cut-off:
Bibra - Proved + Probable Ore Reserve - 43.5 Mt @ 0.9 g/t Au for 37.3 t of contained gold.
The most recent source geological information used to prepare this decription was dated: 2020.
This description is a summary from published sources, the chief of which are listed below. © Copyright Porter GeoConsultancy Pty Ltd. Unauthorised copying, reproduction, storage or dissemination prohibited.
Karlawinda - Bibra
|
|
Bagas, L., Bierlein, F.P., Bodorkos, S. and Nelson, D.R., 2008 - Tectonic setting, evolution and orogenic gold potential of the late Mesoarchaean Mosquito Creek Basin, North Pilbara Craton, Western Australia: in Precambrian Research v.160, pp. 227-244.
|
Ferguson, M.R.M., 2013 - Mineral Paragenesis and Geometallurgy of the Karlawinda Deposit, Western Australia: in University of Tasmania, School of Earth Sciences, CODES Centre of Excellence in Ore Deposits, BSc Hons Thesis, 312p.
|
Porter GeoConsultancy Pty Ltd (PorterGeo) provides access to this database at no charge. It is largely based on scientific papers and reports in the public domain, and was current when the sources consulted were published. While PorterGeo endeavour to ensure the information was accurate at the time of compilation and subsequent updating, PorterGeo, its employees and servants: i). do not warrant, or make any representation regarding the use, or results of the use of the information contained herein as to its correctness, accuracy, currency, or otherwise; and ii). expressly disclaim all liability or responsibility to any person using the information or conclusions contained herein.
|
Top | Search Again | PGC Home | Terms & Conditions
|
|