Tick Hill |
|
Queensland, Qld, Australia |
Main commodities:
Au
|
|
 |
|
 |
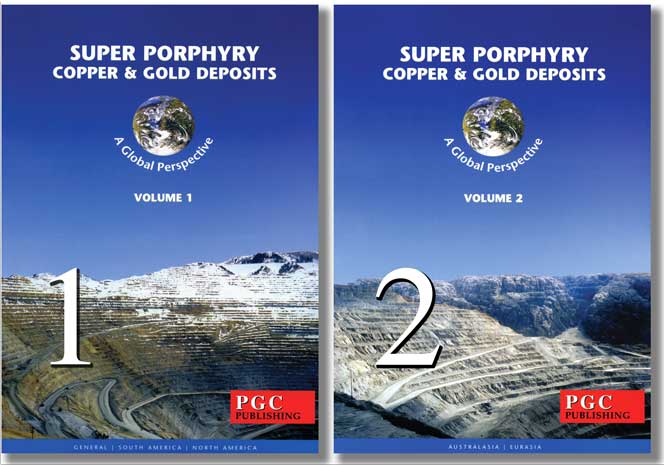 |
Super Porphyry Cu and Au

|
IOCG Deposits - 70 papers
|
All papers now Open Access.
Available as Full Text for direct download or on request. |
|
 |
The Tick Hill gold deposit is situated ~110 km SSE of Mount Isa, ~120 km SSW of Cloncurry and ~30 km SSE of Duchess in northwest Queensland, Australia (#Location: 21° 38' 47"S, 139° 55' 32"E).
The small, but high grade deposit was discovered by Mount Isa Mines Ltd in October 1989 after follow up of a bulk leach extractable gold (BLEG) anomaly from a stream sediment sampling program. Following resource definition, mining commenced in August 1991, with the first gold poured in December 1991. Open pit and
underground mining was undertaken by Carpentaria Gold Pty Ltd and ceased in April 1995.
The deposit produced a total of 15.89 tonnes of gold from 0.706 Mt of ore at an average grade of 22.52 g/t Au. Metallurgical recovery averaged 97% (Le et al., 2021).
The Tick Hill deposit is hosted in high-grade metamorphic rocks and is characterised by the occurrence of free gold with little to no silver and a paucity of sulphides and associated metals. It is located just to the west of the major Pilgrim Fault that defines the eastern margin of the Palaeo- to Mesoproterozoic Mary Kathleen Domain (or Fold Belt), and is near the southern extremity of exposed rocks of that domain, within the Eastern Fold Belt of the Mount Isa Inlier. For an overview of the Eastern Fold Belt see the separate Cloncurry Iron Oxide Copper Gold Province record. To the west, the
metasedimentary rocks of the Mary Kathleen Domain uncomfortably overlie those of the Kalkadoon-Leichhardt Domain, while to the east, they are separated from the rest of the Eastern Fold Belt by the Pilgrim Fault in the south and the Rose Bee Fault in the north (Withnall and Hutton, 2013).
In the core of the Mary Kathleen Domain, gneiss of the 1870 to 1850 Ma Kalkadoon-Leichhardt Complex, the overlying 1780 to 1775 Ma Magna Lynn Metabasalt mafic and felsic volcanics and the 1780 to 1770 Ma Argylla Formation rhyolitic to dacitic tuff, andesite and sedimentary rocks (e.g. Page, 1978; Neumann et al., 2009) are basement to an unconformably overlying shallow water sedimentary sequence (e.g. Withnall and
Hutton, 2013). The latter comprise the ~1755 Ma Ballara Quartzite and extensive developments of the 1750 to 1738 Ma Corella Formation (Blake et al., 1982; Foster and Austin, 2008; Neumann et al., 2009; Withnall and Hutton, 2013; Kositcin et al., 2019). In the central Mary Kathleen Domain, the Corella Formation comprises scapolitic calc-silicate, para-amphibolite, marble, metapelite, quartzite, other metasedimentary units and minor felsic and mafic meta-volcanics (Blake, 1987; Foster and Austin, 2008). Stratigraphic units in the area surrounding Tick Hill have not been dated directly but have been correlated with the Corella Formation (e.g. Wyborn, 1997; Foster and Austin, 2008).
Intrusions in the southern Mary Kathleen Domain have been grouped into granites of the ~1740 Ma Burstall-Wonga Suite (e.g. Monument Syenite, Saint Mungo Granite, Birds Well Granite and Tick Hill Granite) and the older Kalkadoon Batholith of the Kalkadoon-Leichhardt Domain, mostly restricted to the region to the west of Tick Hill (e.g. Blake et al., 1982; Wyborn, 1997; Withnall and Hutton, 2013). Four principal deformational events have been recognised, comprising D1 to D4 (e.g. Passchier and Williams,
1989; Holcombe et al., 1991; Oliver, 1995). D1, interpreted to have occurred between 1750 and 1730 Ma, has been interpreted as extensional and has been linked to an early gneissic fabric exposed in high strain zones along the central section of the Mary Kathleen Domain, known as the Wonga-Shinfield Zone (Passchier and Williams, 1989; Holcombe et al., 1991). Although D2, which resulted in upright folding, has been interpreted to represent the main metamorphic stage of the Isan Orogeny between ~1590 and 1550 Ma (e.g. Page, 1983; Passchier and Williams, 1989; Holcombe et al., 1991; Oliver, 1995), Pence et al. (2020) have shown that it took place before 1710 Ma. The D2 tight, north-trending upright folds are characterised by an axial planar cleavage, a penetrative S2 fabric, and steep east-dipping reverse faults parallel to F2 fold axial planes (e.g. Holcombe et al., 1991; MacCready et al., 1998). D3 events corresponded to a transition from ductile to brittle deformation and the formation of a fault network (O'Dea et al., 1997; Betts et al., 2006). A series of NE and NW striking D3 faults offset the Wonga-Shinfield Zone in the southern Mary Kathleen Domain as a result of east-west compression (Forrestal et al., 1998). D3 was coeval with the regional emplacement of the 1530 to 1500 Ma Wimberu/Williams granite plutons (Foster and Austin, 2008) to the east and the related regional albite-hematite-alteration (Wyborn et al., 1988). The subsequent D4 events involved the brittle reactivation of older fault zones at shallow crustal levels, at temperatures <350 to 400°C, with deformation interpreted to lie within the range of 1500 Ma to 1100 Ma (e.g. Oliver, 1995).
The Tick Hill deposit occurs within rocks interpreted as Corella Formation, bounded to the east by the Pilgrim Fault, and to the west by granitic gneiss of the Saint Mungo Granite. These Corella Formation sequences unconformably overlie felsic volcanics assigned to the Argylla Formation (Coughlin, 1993; Wyborn, 1997). To the north, the deposit area is bounded by the NW-trending Plum Mountain Fault and to the south by the NE-trending Mt Bruce Fault (Forrestal et al., 1998). The Corella Formation sequence was intruded by syn-tectonic (i.e. syn D1-2) Tick Hill Granite and Monument Syenite and post-tectonic pegmatite (Wyborn, 1997; Rutherford, 2000). To the east of the Pilgrim Fault and Tick Hill pit, Cambrian rocks are locally preserved as erosion remnants above the Corella Formation.
In the immediate Tick Hill area, D1 produced a prominent mylonitic gneissic fabric in the rocks that host the Tick Hill deposit. This mylonite was formed along a north-trending belt, west of the Pilgrim Fault, characterised by a prominent 60°W (~280°) dip, and a well-developed steeply SW pitching mineral lineation and/or rodding lineation (L1). This fabric is defined by oriented hornblende-plagioclase-clinopyroxene assemblages in metabasalt, and by silimanite-biotite-scapolite in meta-sedimentary rocks. The presence of numerous quartzo-feldspathic horizons and sheared leucogranite layers are interpreted to indicate abundant granite melt pockets, formed either by local migmatisation, granite intrusion or both. Centimetre to metre scale intrafolial folds and sheath folds within the mylonitic fabric are evident, while in places, several overprinting generations of intrafolial folds have been recognised (e.g. Choy, 1994; Oliver, 1998). F1 fold axes associated with intrafolial folds commonly parallel the mineral lineation. The D1 high strain fabric has been interpreted to be linked with the Shinfield-Wonga belt north of Tick Hill, and is considered to be an expression of a ~1740 Ma, mid-crustal extensional shear zone (e.g. Oliver, 1998). D1 mylonites were deformed during D2 to form a series of tight, kilometre scale, asymmetric upright folds. The Tick Hill deposit is located within the eastern limb of one of these structures, the large D2 Tick Hill syncline, which has an axial planar trace paralleling the D1 gneissic fabric (Choy, 1994). To the west of the Tick Hill pit, the D2 folds tighten and intensify, and become transposed into a north-trending high strain zone that parallels the S1 fabric. This has been interpreted to suggest that D1 and D2 events may be expressions of a single, progressive shearing event (Laing, 1993). D2 folds open up towards the Pilgrim Fault to the east. D3 and D4 have been described as NW- and NE-trending fault sets with associated red rock alteration (Tedman-Jones, 2001). The principal D3 structures bound the Tick Hill area to the north and south. Internally, within the deposit, D3 is occurs as an interconnected network of fractures and veins with associated quartz-feldspar alteration and gold mineralisation (Tedman-Jones, 2001). D4 deformation includes a series of NE trending strike-slip faults that offset the D1 Tick Hill gneiss fabric and D2 fold axes (Coughlin, 1993), and overprint the D3 red rock alteration (Tedman-Jones, 2001).
The host rocks to the Tick Hill deposit comprise gneissic and schistose units interpreted to belong to the Corella Formation (e.g. Coughlin, 1993; Choy, 1994; Wyborn, 1997) and comprise from west to east, from hanging wall to footwall as described by Le et al. (2021):
• Hanging wall calc-silicate interlayered with amphibolite gneiss, comprising banded or laminated gneissic calc-silicate and amphibolite, interbedded with lesser biotite schist. It has been subdivided into a series of sub-units:
- Gneissic amphibolite, which has a strong foliation defined by oriented hornblende, which comprises 50 to 80% of the rock, with the remainder comprising plagioclase, scapolite, quartz, magnetite, actinolite, garnet and accessory minerals such as zircon and apatite (enclosed in quartz).
- Gneissic calc-silicate, which constitutes the bulk of the rock in the sequence above the hanging wall quartzite. It is generally composed of feldspar (40 to 60%), scapolite (20 to 35%), quartz (10 to 15%), magnetite (<3 to 4%) with variable hornblende, actinolite and chlorite. Scapolite is very variable from one layer to the next, and where most abundant preserves a spotty texture, with individual grains aligned within the foliation. Hornblende is found as discontinuous patches following the gneissic foliation and locally defines a mineral lineation. Amphibole-rich layers are composed of quartz-feldspar-amphibole ±scapolite, biotite and magnetite, and occur at intervals within the more massive calc-silicate sequence.
- Calc-silicate, which is generally altered and overprinted by sericite and clay after feldspar, and chlorite-hematite-goethite after amphibole-biotite-magnetite. Layers of biotite-schist are locally intercalated, but are too thin and laterally too discontinuous to be mapped as separate units.
- Magnetite-rich calc-silicate, which is found directly above the hanging wall quartzite and locally may be as thick as 30 m. It has a similar composition to the calc-silicate sub-unit, other than the magnetite content, which is much higher. The magnetite concentration increases toward the contact with the overlying quartzite, to form a 4 to 6 m wide contact zone comprising discontinuous, semi-massive to massive granular magnetite lenses. The magnetite-rich calc-silicate is locally intercalated with thin bands of magnetite-bearing amphibolite. The magnetite is aligned parellel to the gneissic fabric, apparently having formed as an early alteration product overprinting and replacing the dominant rock forming minerals, as shown by the presence of abundant inclusions of magnetite in biotite (Le et al., 2021).
• Hanging wall quartzite, which has been intensely deformed and in the immediate hanging wall to mineralisation it has been overprinted by quartz-feldspar alteration. It is grey-white in colour and dominated by quartz, although there is a variation in the composition of secondary minerals that occur as inclusions in quartz. It is locally enriched in biotite, or hornblende-chlorite-epidote or feldspar, with inclusion phases aligned parallel to the gneissic layering and partly overgrown by quartz. It is magnetite-bearing along the contact with the overlying calc-silicate unit, although the magnetite content decreases away from the contact, to form a weakly magnetic central interval of glassy quartzite that is discontinuous along strike. The magnetite-rich zone within the quartzite locally occurs directly above the mineralised zone, especially in amphibolite-rich areas. Silicified biotite schist intercalations are locally common. The unit has an annealed granoblastic texture, with the dominant foliation only weakly preserved. It can also be intensely brecciated and pervasively altered producing a spotty texture.
• Biotite schist, which occurs between the hanging wall and footwall quartzite units and comprises three sub-units:
- Quartz-feldspar-biotite-amphibole schist or meta-psammite, that occurs along the base of the hanging wall quartzite, separating the quartzite from the ore zone. The transition from quartzite to biotite schist is gradational, with its exact position masked by silicification. The least altered sections comprise a fine-grained, light-tan, massive to weakly foliated arkose siltstone or arenite, with a composition that varies laterally, including quartz (35 to 60%, including secondary quartz due to silicification), feldspar (15 to 20%), biotite (5 to 20%), amphibole (10 to 20%) and magnetite ±ilmenite (2 to 3%). Oriented biotite, amphibole and quartz produce a strong schistose fabric.
- Biotite-schist with local sillimanite or meta-pelite, which commonly occurs below the quartz-feldspar-biotite-amphibole schist and extends into the footwall of the ore zone. Biotite-rich varieties follow the layering, whilst sillimanite is locally abundant, especially in footwall sections of the ore zone, although much of the sillimanite has been replaced by muscovite. Within the ore zone, the biotite schist has been intensely silicified and altered to chlorite-hematite, with the pre-existing mylonitic texture strongly recrystallised into an interlocking texture of granoblastic quartz grains. This sub-unit is generally composed of fine- to coarse-grained biotite and/or biotite-amphibole (30 to 80%), with quartz (<30%) and feldspar (K feldspar and plagioclase, <50%) that was partially altered to sericite and clay minerals.
- Quartz-feldspar mylonite, that is the principle host to gold mineralisation, and based on its composition, is probably of intrusive origin, representing intrusive granite sheets or granitic migmatite (Le et al., 2021). It occurs as layers within biotite-schist and comprises equal amounts of quartz and feldspar (albite and oligoclase) that occur as discontinuous ribbon grains interlaminated with thin quartz bands. The individual quartz and feldspar lamellae are generally <3 mm in thickness, and they are mostly thinner, although in places quartz-rich or feldspar-rich bands (or veins) can be up to 10 cm thick. The feldspar is predominantly albite with minor K feldspar and Na-rich oligoclase. The quartz-feldspar mylonite has a characteristic sugary, granoblastic texture, reflecting post-deformational annealing. Recrystallized fine-grained quartz-feldspar mylonite is locally is truncated, or overprinted, by laminated <2 mm partings of grey quartz, which is parallel, or at low angles, to the original mylonitic foliation (Le et al., 2021).
- Minor intercalations of amphibolite gneiss and quartzite.
• Footwall quartzite, which has a thickness that is variable along strike and with depth, pinching out towards the southern end of the pit. It comprises thickly bedded (>30 cm) grey-white translucent orthoquartzite with traces of biotite, magnetite and heavy minerals including zircon and apatite. It has a granoblastic texture with a grain size that is commonly 150 to 350 µm, and has been strongly deformed. However, it lacks the spotty texture resulting from abundant inclusions of feldspar, magnetite, hornblende or biotite, and alteration, which characterises the hanging wall quartzite.
The origin of the Hanging wall and footwall quartzite ridges is not certain, often referred to as 'metasomatic alteration zones', rather than sedimentary units, based on their cross-cutting, discontinuous nature (e.g. Laing, 1993; Coughlin, 1993; Oliver, 1998).
• Footwall calc-silicate interlayered with amphibolite gneiss, similar in appearance to the various calc-silicate and amphibolite lithologies described above. In the immediate footwall to the footwall quartzite unit, calc-silicate lithologies dominate, whilst distal to the quartzite it is transitional into amphibolite.
• Late pegmatite veins that are late-tectonic in character, intruded into the gneiss units as dykes, whilst the gneissic layering is locally overprinted by pegmatite-like metasomatic alteration zones, which developed as rims along late fractures. The veins and dykes are somewhat deformed and locally boudinaged, but have no penetrative, mylonitic deformation fabrics and cut across the pervasive gneissic layering that characterises the host sequence. The late pegmatite veins are generally <0.6 m thick dyke-like bodies that preserve a fine- to medium-grained texture of intergrown plagioclase and quartz near the margins of the dykes with a core dominated by plagioclase (85 to 95%) with variable quartz (<10%), K feldspar (<5%) and biotite (0 to 10%). Pegmatite-like veins occur in the footwall of the ore body, where they are associated with metasomatized zones with a granular texture and diffuse boundaries in which feldspar (30 to 40%), quartz (20 to 30%) and minor biotite (<5%), overprint and replace the underlying metamorphic fabrics. Thesc pegmatite intrusions were strongly affected by later hydrothermal alteration, in which feldspar was replaced by an albite-sericite assemblage.
Mineralisation
The orebody, as defined by the 1 g/t Au envelope, is carrot-shaped in section, tapering downward over a vertical depth of 240 m (MIM, 1993, 2000; Tedman-Jones, 2001). It is more tabular in plan with a strike length of ~150 m, dipping at ~55°W, parallel to S1, with a linear high-grade 16.5 g/t Au core that plunges at 55° and azimuth of 285°. The strike extent of the ore zone decreases with increasing depth and on the two lowest mine levels (130 mRL and 117 mRL), the ore trend switches abruptly from NNE to east where it has been described as narrow chlorite-hematite-carbonate breccia veins that are up to several metres in thickness (Tedman-Jones, 2001; Le et al., 2021).
At surface, the >0.1 ppm Tick Hill geochemical gold anomaly was ~160 x 30 m, which coincided with a pre-mining, NNE-trending, 210 x 50 m magnetic low (Nano et al., 2000). The gold anomaly overlaps a strongly D3 fractured and hematite-altered zone in the immediate hanging wall of the D1 foliation truncation plane that was reactivated as a D3 shear zone. The plunge of the high-grade core of the ore zone coincides with the intersection lineation between the NNE- and east-trending D3 faults, and the intersection lineation between the D3 faults and the S1 foliation planes. The plunge of the high-grade core is also oriented close to the measured L1 mineral lineation described above. The low grade 0.1 g/t Au envelope coincides with areas where east-trending D3 fractures and breccia zones are most prominent, and extends outside the pit along some of the more prominent east-trending D3 fracture zones (Le et al., 2021).
As detailed above, gold mineralisation is largely restricted to the strongly altered quartz-feldspar mylonite sub-unit and adjacent biotite schist, calc-silicate and hanging wall quartzite. The mineralisation boundaries to are sharp, with grades dropping abruptly away from the D1-2 silificied and D3 altered fracture zones. Gold can commonly be seen as an alignment of coarse grains that parallel the dominant mylonitic S1 fabric. These gold grains are also commonly found associated with thin laminar D3 quartz veins, which either occur along the S1 mylonitic foliation or along steep east-trending fractures. The is also a spatial relationship between gold mineralisation and D3 alteration haloes near coarse-grained post-tectonic quartz-feldspar pegmatite dykes, with fine gold grains typically occurring near patches of chlorite, albite and hematite. The east-trending quartz-feldspar pegmatitic veins are locally associated with breccia zones, which are most prominent at depth in the immediate footwall of high-grade ore zones. At shallower levels where breccia is less common, high grade mineralisation is concentrated in areas of intense laminar D3 veining (Le et al., 2021).
Gold is almost exclusively present as disseminated grains of free gold that may be up to 0.5 mm in size with Au:Ag ratios of >100. The quartz-feldspar mylonite is locally extremely gold-rich (e.g. up to 3209 ppm @ 0.5 m in one drill hole). Gold grains in such intervals locally display a characteristic granoblastic texture, and commonly exhibit a bimodal grain size distribution where irregularly shaped larger grains are surrounded by a halo of finer grains (e.g. Choy, 1994). Some of the finer grains contain minor Ag, generally <5.3 wt.%) and <2 wt.% Cu. Coarse-grained gold is frequently enclosed within recrystallised quartz, with the quartz occurring as a metamorphic 'moat' surrounding the gold. Gold is also found along the boundaries between quartz and feldspar grains. The calc-silicate units host gold that is locally enclosed within granoblastic scapolite, hornblende and pyroxene grains that are aligned in the peak-metamorphic S1 fabric. Fine-grained gold is found either as haloes near larger gold grains, or as trails associated with strongly silicified zones and alteration minerals that included bismuth selenides and sulphides. The sulfide content in the mineralised quartz-feldspar mylonite is generally less than in the other units, with the abundance of sulphides increasing towards the footwall of the deposit (Le et al., 2021).
Only minor enrichment in other metals and sulphides is found within the high-grade core of the deposit. Available assay data for Cu, Co, Ni, Pb, Zn, As, Fe, Mn (MIM, 2000) indicate that, with few exceptions, on a metre-scale, there is no correlation between gold and any of the other metals. On the deposit scale, there is a general enrichment in Cu (>100 ppm), Co (>40 ppm), S, As and Bi suggested in the immediate footwall of the ore zone (Nano, 1999; Tedman-Jones, 2001), with minor Co enrichment of >40 ppm partially overlapping the 1 g/t Au envelope. Cu values may be as high as several thousand ppm in the footwall to the ore zone and can be linked to chalcopyrite-pyrite and minor bornite-pyrrhotite mineralisation, which is possibly also responsible for an induced polarization anomaly that was observed over the Tick Hill ore body (Nano, 1999). However, Cu values are rarely elevated in the ore zone itself (Le et al., 2021).
The Tick Hill deposit is associated with two periods of alteration:
• An early high temperature event during D1 that involved emplacement of gold incorporated in hornblende and diopside grains aligned in the S1 fabric, although evidence for this early event is only partly preserved, due of the intensity of the later stages of hydrothermal overprints during D3. This D1 alteration is characterised by intense silicification and associated magnetite alteration that is most evident within the hanging wall quartzite unit.
Within the ore zone, silicification of this phase was intense and involved the growth of quartz at the expense of other silicates, which now occur as inclusion trails in quartz. Early magnetite was largely destroyed during the lower temperature D3 hydrothermal overprint during which magnetite was replaced by hematite. Gold grades have a negative correlation with magnetic susceptibility, which on a larger scale is concistent with an aeromagnetic low that coincides with the ore zone and the D3 alteration halo.
• A second event at lower temperatures during D3, which resulted in the development of a low- to moderate-temperature alteration zone that is ~100 m in diameter, overprinting the earlier high temperature alteration assemblages as well as the D1 peak metamorphic mineral assemblages and associated structures. This alteration best developed within the ore zone and its hanging wall rocks, above the S1-3 foliation truncation plane, whilst the footwall rocks to that surface are significantly less altered (Geological Survey of Queensland, 2015). This lower temperature alteration assemblage varies with rock type and exhibits a zonal pattern, with the most intense alteration occurring along D3 fracture zones and associated breccia. From the proximal mineralisation outward, the alteration zonation includes: i). intense to ii). strong silicification, with an associated growth of abite, hematite, chlorite, epidote, sericite, actinolite/hornblende, oligoclase, surrounded by iii). a shell characterized by chlorite and epidote, and iv). an extensive zone with clay alteration. Boundaries between these zones are gradational with localised overlaps in alteration assemblages, whilst late clay alteration occurs throughout (e.g. Geological Survey of Queensland, 2015). Within the high-grade ore zone, intense silicification altered the quartz-feldspar mylonite and biotite-rich schist, with pre- or early-D3 pervasive silicification causing quartz growth across the mylonitic fabric. Later silicification is linked to the emplacement of thin quartz veins (sub-)parallel to the S1 foliation, and the growth of quartz in alteration haloes near quartz-feldspar veins and pegmatite dykes. Carbonate alteration is generally found as a late-D3 and D4 fracture fill. Calcite locally occurs as secondary growth along sheeted quartz veins and east-trending fractures in the mineralised zone, although it is not pervasive. However, in the lower keel of the deposit, gold was mined from east-trending breccia veins that were reported to contain chlorite-hematite-carbonate in the matrix (Tedman-Jones, 2001). This implies carbonate growth may have been more abundant at depth and occurred in associated with gold.
The D3 alteration and mineralisation is interpreted to have involved a strongly oxidised, acidic, saline, hydrous fluid. (Le et al., 2021) suggest there is also a possible genetic relationship between syn-D3 pegmatite and late-stage alteration fluids. They also suggest that in terms of alteration assemblages and structural setting the Tick Hill deposit shares many similarities with the ~1850 Ma, Au-Cu-Bi mineralisation in the Tennant Creek area. They also suggest that in terms of alteration characteristics, the Tick Hill deposit shares similarities with IOCG deposits of the Mount Isa Inlier, Eastern Fold Belt. Tick Hill lies within the same widespread albite-hematite-scapolite epidote alteration domain as the IOCG deposits of the province. This alteration is interpreted to be linked to the remobilisation of evaporite deposits during various deformational and intrusive events (e.g. Oliver et al., 1994; Oliver, 1995; De Jong and Williams, 1995; Withnall and Hutton, 2013) and is accompanied by widespread brecciation, iron oxide alteration and the syn- to post-tectonic emplacement of both felsic and mafic intrusions (e.g. Oliver, 1995; Oliver et al., 2008). However, they conclude, its high-grade gold-only nature, lack of Cu, and the presence of Bi-selenides are at variance with most IOCG systems and make it a unique deposit in the Mt Isa Inlier. However, it association with the same regional mineralising system that produced the IOCG deposits of the province cannot be discounted.
The information in this summary is drawn from, and closely follows Le et al. (2021) as cited below. See that reference for more detail.
The most recent source geological information used to prepare this decription was dated: 2021.
This description is a summary from published sources, the chief of which are listed below. © Copyright Porter GeoConsultancy Pty Ltd. Unauthorised copying, reproduction, storage or dissemination prohibited.
Tick Hill
|
We are endeavouring to ascertain why those pages that display Google Maps cannot now do so correctly, and will remedy as soon as practical.
|
|
Le, T.X., Dirks, P.G.H.M., Sanislav, I.V., Huizenga, J.M., Cocker, H.A. and Manestar, G.N., 2021 - Geological setting and mineralization characteristics of the Tick Hill Gold Deposit, Mount Isa Inlier, Queensland, Australia: in Ore Geology Reviews v.137, 25p. doi.org/10.1016/j.oregeorev.2021.104288.
|
Le, T.X., Dirks, P.H.G.M., Sanislav, I.V., Huizenga, J.M., Cocker, H.A. and Manestar, G.N., 2021 - Geochronological constraints on the geological history and gold mineralization in the Tick Hill region, Mt Isa Inlier: in Precambrian Research v.366, 27p. doi.org/10.1016/j.precamres.2021.106422.
|
Porter GeoConsultancy Pty Ltd (PorterGeo) provides access to this database at no charge. It is largely based on scientific papers and reports in the public domain, and was current when the sources consulted were published. While PorterGeo endeavour to ensure the information was accurate at the time of compilation and subsequent updating, PorterGeo, its employees and servants: i). do not warrant, or make any representation regarding the use, or results of the use of the information contained herein as to its correctness, accuracy, currency, or otherwise; and ii). expressly disclaim all liability or responsibility to any person using the information or conclusions contained herein.
|
Top | Search Again | PGC Home | Terms & Conditions
|
|