Timna Valley |
|
Israel |
Main commodities:
Cu Ag
|
|
 |
|
 |
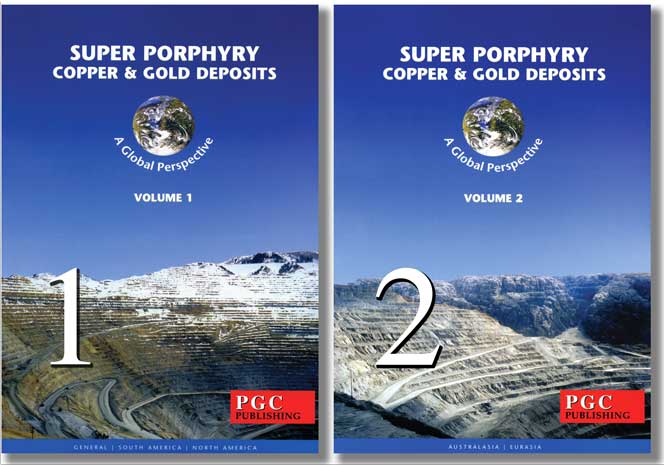 |
Super Porphyry Cu and Au

|
IOCG Deposits - 70 papers
|
All papers now Open Access.
Available as Full Text for direct download or on request. |
|
 |
The historic Timna copper deposit is located within the Negev Desert of southern Israel, ~20 km north of Aquaba. It lies on the western margin of the Wadi Araba which straddles the Jordan (or Dead Sea) Rift Valley and lies along the boundary between the African and Arabian plates. The rift includes the Gulf of Aqaba, the Dead Sea and Sea of Galilee. The principal structure related to that rift valley, and the plate boundary, is the Wadi Araba-Dead Sea Transform Fault, which is interpreted to have displaced Timna by ~100 km of sinistral movement from its assumed original location adjacent to the Feinan deposit, now in Jordan to the NNE. Other related mineralisation, well to the west of the Wadi, include the Bir Nasib District copper and turquoise cluster, 170 km SW to WSW of Timna, on the southwestern margin of the Sinai Peninsula in Egypt. (#Location: Modern Timna Mines - 29° 45' 43"N, 34° 58' 57"E).
The Wadi Araba (or Aravah Valley), is a low-lying, north-south elongated desert, stretching from the head of the Gulf of Aquaba (or Eilat) in the south, to the Dead Sea, ~165 km to the north. It is bordered along its eastern margin by the steep fault escarpments of the Edomite Plateau, while to the west it is flanked by the more gradual slopes of the Negev Highlands. The Timna Valley is ~10 km long and has a horse-shoe shape, flanked by ~300 to 500 m high cliffs of shallowly to flat dipping Cambrian and Cretaceous sedimentary sequences on its outer margin to the north, west and south. To the east, at both ends, it opens into the Wadi Araba. The core of this topographic feature is occupied by the east-west elongate Har Timna, an ~5 x 3 km massif of basement Proterozoic volcano-sedimentary and intrusive rocks. The tallest peak of this massif is the 448 m high Mount Timna, which overlooks the near sea level centre of the Wadi Araba to the east. Copper-rich nodules containing up to 55% Cu, mainly of malachite and chalcocite, have been mined in ancient times, mainly from the Cambrian and Cretaceous sequence in the lower parts of the cliffs bounding the valley, and from the slopes above. It is believed to have been one of the most significant centres of copper mining and metallurgy in antiquity. More than 10 000 ancient mine shafts and diggings have been outlined, distributed throughout the length of the Timna Valley.
Copper has been exploited in the Timna Valley, since at least the 5th millennium BCE, from when the earliest, well-preserved copper smelting furnaces have been dated. Over the succeeding three millennia, copper continued to be produced with steadily improving furnaces and metallurgical processes. Smelter technology advanced from the 'hole in the ground' hearth of the early Chalcolithic Period to the first stone-built bowl furnaces with primitive slag tapping of the Early Bronze Age, to the well ventilated, clay lined, shaft furnaces with efficient slag tapping of the late Bronze Age. By the Chalcolithic Period in the late 4th millennium BCE, it had already been appreciated that the addition of iron oxide as flux to the smelting charge of copper ore and charcoal, greatly improving the smelting process. Whilst some of the earliest evidence of extractive metallurgy are revealed at Timna, it was not until a half-millennium period in the Late Bronze and Iron ages, during the 13th to 9th centuries BCE, that the valley achieved its industrial peak. This increased activity has been initially attributed to Egyptian intervention during the 19th and 20th Dynasties of the New Kingdom, i.e., straddling the reigns of Seti I to Ramesses V, from ~1300 to 1140 BCE. The involvement of Egyptian mining expeditions is documented by numerous hieroglyphic inscriptions inside an Egyptian temple dedicated to the goddess Hathor built in Timna during the reign of Seti I at the end of the 14th century BCE. At the end of the Late Bronze Age, Egypt was weakened by internal struggles, and withdrew from the region. This was coincident with the political collapse of other major influential centres across the Eastern Mediterranean, particularly affecting the copper industry of Cyprus that had been the main source of the metal throughout the region. This Egyptian exodus left the lucrative copper industry in the hands of the local nomadic society that became the Edomite Kingdom. During the ensuing ~300 years, reaching a zenith in the 10th century BCE, intense production took place, whilst the copper mines at Feinan, ~100 km to the north, were also reopened by the Edomites on a large scale. This activity was part of the reorganisation of production in the entire region. The Feinan deposits are also within the Wadi Araba, although there is no archaeological evidence of their having been under direct Egyptian influence (Cavanagh et al., 2022). However, whilst the Egyptians had retreated from Timna by this time, it is suggested that they still disseminating technological knowledge to the Edomites and relied on their mines for the copper they required (e.g., Lehner et al., 2023).
The increased copper production during this period was accompanied by the consolidation of smelting activities, previously dispersed throughout the valley, to two newly walled sites where thousands of tons of slag are preserved, reflecting the scale of activity that took place there. However, to produce this amount of slag, representing hundreds of tons of copper metal, a commensurately greater magnitude of wood would have been required to fuel the furnaces. Studies of the slag indicates the ancient smelters were aware that the best woody fuels available to them in this arid environment were the white broom and acacia trees, which produced dense and high temperature burning wood and tree roots. The likely seasonal arrival of the nomadic mining community and their herds, during this period and the over-exploitation of the slow regenerating fuel and pastural flora appears to have upset the equilibrium of the delicate ecosystem of this extreme environment. This is evidenced by the late and increasing appearance in the slag of other flora, particularly juniper and pistacia trees, that are unsuited to the extreme local conditions, and hence were sourced at greater expense from a longer distance. Production of copper from the Timna Valley came to a sudden halt in the middle of the 9th century BCE. This short duration final stage of activity, when the local flora disappeared from slag, coupled with the total cessation of the lucrative copper industry in the Timna Valley, is interpreted to suggest the diminishing availability of local fuel sources in the southern Wadi Araba was a primary reason for the termination of the industry. Copper mining, which had been operating continuously for about four centuries previously, ceased, and remained closed for the following almost one thousand years (Cavanagh et al., 2022).
The mines at Timna were next exploited, but on a much-reduced scale, by the local Nabataean Kingdom during the 1st century CE, until their conquest by the Romans in 107 CE. Mining continued through the remainder of the second to the fourth centuries CE, apparently by soldiers of the third Roman legion of Cyrenaica, with the copper ore being transported to a large copper furnace at Be'er Orah, south of Timna. Later, following the 7th century CE, Arab conquest of the area, extraction continued under the Umayyad Caliphate, again smelted at Be'er Orah, until the known economically mined copper ore was exhausted.
The modern state of Israel commenced mining copper in the Timna Valley in 1959, but ceased in 1976 due to falling copper prices, before reopening in 1980, but closed permanently in 1985. Mining was undertaken by the state owned Timna Mining Company, from both open pits and underground workings spread over an area of 22 km2. The operation produced ~80% Cu copper cement, with an output of ~5000 tonnes of contained copper in the first year, ramping up to 14 000 tonnes in 1962. This production was based on 'reserves' at Timna in 1959 of ~11 Mt at an unspecified grade (US Bureau of Mines, Mineral Trade Notes Nov 1959). Resources quoted in 2007 (Cox et al. 2007 USGS Open File Report 03-107) were 18.1 Mt @ 1.6% Cu for ~0.29 Mt of contained copper.
In 2005, Arava Mines Ltd, a subsidiary of the Mexican steel company Altos Hornos de Mexico SA, commenced exploration for new resources and began rehabilitating the previous modern workings in preparation for re-opening. This included construction of a new 24 000 tonnes per annum solvent extraction-electrowinning (SX-EW) plant facilitated by a grant from the Israeli Government. However, following the government changing the criteria for the grant, operations were suspended in 2008. No further work has since been entered into.
In 2002, an area of 42 km2 enclosing the main archaeological sites of the Timna Valley was declared as the Timna Park nature reserve, ending all mining activity within the reserve's area.
Geological Setting
The Regional Setting of the Timna Valley deposits is interpreted to be a fault offset continuation of that recorded in the Feinan section of the Wadi Araba of Jordan, ~100 km to the north. However, there are facies variations and differences in the stratigraphy, and the preserved sequences between the major unconformities, as well as a difference in stratigraphic nomenclature used in Jordan and Israel.
The geology of Timna Valley and surrounds can be summarised, as follows, from the basement upwards (after Beyth, Segev and Ginat, 2013):
▸ Neoproterozoic crystalline basement, exposed as the Timna Igneous Complex within the Har Timna Massif, in the core of the horseshoe shaped Timna Valley. Regionally, on the northern tip of the Arabian Nubian Shield, the Neoproterozoic crystalline basement is divided into the older Aqaba Complex, composed of migmatite, paragneiss and schists, mainly after intrusives, and the younger, less metamorphosed sedimentary and volcanic rocks of the Aqaba Complex. The two complexes are separated by a ~605 Ma unconformity as described in the Wadi Araba - Feinan record. The Aqaba Complex is, in turn, overlain by a Lower Palaeozoic succession, across a 530 Ma unconformity.
Within the Har Timna Massif, the crystalline basement is predominantly composed of felsic to mafic high level plutons (Vafnik, 1998) and dykes (Beyth and Peltz, 1992). These include the Timna alkali granite, interpreted to have formed by fractional crystallisation, and an olivine norite, developed as a cumulate in a quasi-layered magmatic cell. Both of these magmatic lithofacies are interpreted to have formed at ~610 Ma , within a 625 Ma calc-alkaline, porphyritic granite (Shpitzer, 1992: Beyth et al., 1994).
The pre-610 Ma monzodiorite, olivine norite and alkali granite intrusives are cut by two distinct phases of rhyolite, andesite and composite dykes. A north-south oriented set predates a 600 Ma quartz monzodiorite, whilst ~593 Ma, ENE trending dyke swarms post-date the latter quartz monzodiorite (Katzir et al., 2007). No igneous rocks have been identified between 590 Ma and 532 Ma. Late doleritic dykes, which are overlain by the Cambrian sandstones, strike at 120° and are dated at 532 Ma (Beyth and Heimann, 1999). This series of dykes is interpreted to reflect a transition from arc-like geochemical signatures (silica oversaturated, low Ti , and strong Nb depletion) of the pre-605 Ma magmatic complex - representing the regional Aqaba Complex, to the Araba Complex, which is overwhelmingly intraplate in character (i.e., marginally saturated in silica, high Ti content and no Nb depletion; Beyth et al., 1994). As such, the latter is most likely only present as intrusives which include some or most of the older north-south dykes, the ~600 Ma quartz monzodiorite, the ENE trending dyke set and the younger dolerites. The Araba Complex volcano-sedimentary sequence therefore appears to have either not been deposited locally, or was eroded, with only the feeder dykes preserved.
▸ Early to Mid Palaeozoic Succession, comprising the:
Cambrian Yam Suf Group, which has a maximum thickness of 220 m in southern Israel, and is composed of three predominantly sandy units:
• Early Cambrian Amudei Shelomo (or Amudei Shlomo) Formation, which is 0 to 90 m thick, is largely composed of fine-grained to grit size, rounded and poorly sorted, brown, red and grey subarkose, intercalated with lenses or beds of quartzitic pebble-conglomerate. The sub-arkose comprises up to 90% quartz grains, up to 15% feldspar, mainly microcline, up to 10% lithic fragments, and heavy minerals. It exhibits large to medium scale trough and planar cross stratification, from 5 to 250 cm thick made up of 0.2 to 10.0 cm beds. The unconformity above the crystalline basement is frequently directly overlain by a basal conglomerate, locally containing angular granitic boulders up to 2 m in diameter. Elsewhere, the basement is overlain by a dark red-brown micaceous silty clay bed a few metres thick, possibly representing a palaeosol. These rocks were deposited in a fluviatile environment, above a widespread, usually peneplained, erosional unconformity, with up to 90 m deep palaeo-channels running east-west and NW-SE. The lower limit is temporally constrained by the underlying 532 Ma dolerite dykes which are cut by the basal unconformity, whilst the upper contact, which is regular, is overlain by the Timna Formation with a biostratigraphic age of Upper Georgian in the late Lower Cambrian (Parnes, 1971; Karcz and Key, 1966).
• Early to Middle Cambrian Timna Formation, which varies between 0 and 44 m in thickness, is exposed in two basins, the northern Timna and southern Elat basins, which are separated by the east-west trending Amram-Neshef ridge (Segev, 1984). Trilobites (Parnes, 1971; Elicki and Geyer, 2013) and brachiopods (Cooper, 1976) indicate the Early- to Middle Cambrian age of the sequence which represents a transgressive-regressive lagoonal cycle. It marks the first Phanerozoic marine transgression above the crystalline basement in the northern Arabian-Nubian Shield, and crops out on both sides of the Dead Sea Rift Valley. In southern Israel it occurs in the vicinity of the Timna Valley, Hat Amram and Elat to the south, persisting into southeastern Sinai in Egypt. To the north and east, on the Jordanian side it is correlated with the Burj Formation from Feinan to the northern Dead Sea (e.g., Segev, Beyth and Bar-Matthews, 1992). It has been sub-divided into the:
- Hakhlil Member, which is essentially composed of silt and sandstone with rhyolitic breccia at the base (Beyth, Segev and Ginat, 2013). In more detail is comprises (after Segev, Beyth and Bar-Matthews, 1992):
i). Basal Conglomerate, that is a 0 to 3.75 m thickness of pink to brown bimodal polymictic conglomerate with up to 20 cm diameter, poorly sorted, angular rhyolitic fragments, and well rounded, well sorted quartzitic pebbles up to 5 cm in diameter;
ii). Laminated Sandstone, which is 1.7 to 5 m thick and composed of well laminated sandstone, which varies from fine grained to grit, and is mainly cemented by carbonate in the western Timna Valley;
iii). Carbonatic Unit, that is 1.2 to 8.5 m thick, with two end member facies; a). fine-grained sandstone to grit, cemented by dolomite and calcite, and b). sandy dolostone. These beds have cross-stratified internal structures, ripple marks, crawl marks, mudcracks, and pseudomorphs after halite crystals. The carbonate layers are interbedded with red, purple and green siltstones and shales, in places containing manganese oxides;
iv). Silty Shaly Unit, composed of 3 to 6 m of varicoloured shales and silts containing thin beds and lenses of limestone and dolostone that forms a 'soft' recessive weathering unit that serves as a good marker indicating the upper Hakhlil Member contact.
The lower boundary of the Hakhlil Member locally oversteps the underlying Cambrian sequence to directly onlap the crystalline basement across an angular unconformity, particularly in the Har Timna Massif and western Timna Valley. However, where in contact, the boundary with the underlying Amudei Shelomo
Formation is conformable. The upper boundary with the Sasgon Member is also conformable.
- Sasgon Member, which constitutes the upper Timna Formation, is composed of three lithofacies. The lower two, the dolomitic and the sandy facies are laterally equivalent, whilst the third, the shaly lithofacies, overlies the other two. These facies are as follows (after Segev, Beyth and Bar-Matthews, 1992):
i). Dolomitic Lithofacies (previously Nimra Member), which, within the Timna Valley, has a maximum thickness of 28 m and comprises well-bedded sandy dolostone and dolomitic sandstone. Sedimentary textures include laminar stromatolites, cross-stratification, ripple marks and common fossil remnants, all indicating a shallow water to marine regime (Segev,1996; Segev and Sass, 1989).
ii). Sandy Lithofacies (previously the Zebra or Nehushtan Member), which are largely manganese-rich, and may be up to 21 m in thickness, but is generally 5 to 7 m. It is made up of fine-grained to gritty sub-arkose, cemented by manganese oxides, clay minerals and often also by authigenic fluorapatite. This lithofacies includes steeply inclined cross-stratification, deformed and contorted laminations, collapse structures, poorly sorted breccias and intra-formational faults (Segev, 1986; Segev and Sass. 1989). The sandstones carry an average of 2.83% Mn, occurring as manganese oxides, mainly hollandite, cryptomelane, pyrolusite and coronadite (Bar-Matthews, 1986; 1987).
iii). Shaly Lithofacies (previously the Mikhrot Member), which has a uniform thickness of 1.5 to 2 m, overlies both the dolomitic and the sandy lithofacies. It is composed of usually light green, red or brown clay, siltstone and fine-grained subarkose, which in places are black due to manganese mineralisation. The shales are contorted, and are often brecciated at the contact with the overlying Shehoret Formation. Locally, cryptocrystalline fluorapatite/micritic phosphorite occurs as small lenses that are tens of cm in length and up to 15 cm thick within one or two horizons in the shaly lithofacies, commonly containing 400 to 750 ppm U.
At a number of locations within the eastern Timna Valley, the Sasgon Member is a sandstone and grit bed containing a few well-rounded quartzitic pebbles. The maximum thickness of this coarse facies is 7.5 m, although it rapidly wedges out to the west.
Segev, Beyth and Bar-Matthews (1992), suggest that, based on analysis of the facies distribution, sedimentology, petrochemical and geochemical data, the lateral transitions between the dolomitic and the sandy lithofacies was not depositional, but rather that the sandy lithofacies is the in situ insoluble residue following dissolution of the carbonate/dolomite of the sandy dolostone of the Dolomitic Lithofacies by acidic groundwater/diagenetic fluids. This dissolution is interpreted to have also resulted in deflation and thinning of the Sasgon Member sequence in the transition between the dolomitic to sandy lithofacies.
The Amudei Shelomo and Timna formations both onlap the palaeo-relief of Ihe Neoproterozoic crystalline basement as exposed in the Har Timna Massif. As a consequence, the Timna Formation decreases in thickness from 45 m in the western Timna Valley to zero, to the north and east, and to the south at Har Amram, which is ~7 km south of the Timna Valley. The upper contact between the Timna and Shehoret formations is conformable (e.g., Weissbrod,1969; Segev, Beyth and Bar-Matthews, 1992).
• Shehoret Formation, which varies from 35 m thick in the southern part of the Timna Valley, to 25 m in the north. The formation is mainly composed of Middle Cambrian silt, sandstone and sub-arkose. It forms steep, reddish cliffs above the brown to black, soft, massive, Timna Formation, and has been divided into three members:
- A lower Multicoloured Member at the base of the formation, which is restricted to the Timna Valley. It is composed of alternating beds of yellowish, fine to coarse-grained grit and pebble subarkose, with interbeds of reddish brown silt and clay. In locations with high basement relief, as in the northern Timna Valley and Har Amram to the south, the Multicolored Member oversteps the preceding stratigraphy and rests directly on crystalline basment.
- The White Member, which does not outcrop in the Timna Valley, is exposed to the south of Har Amram, and is almost entirely composed of fine-grained arkose. Unlike the Multicoloured and overlying Variegated members, which were deposited in a fluviatile environment, this facies was laid down in a marine littoral regime (Weissbrod, 1981).
- The Variegated Member contains cross-bedded sandstones and horizontally layered silty clay beds.
Where both units are present, the contact between the Shehoret and Sasgon formations is conformable, but is typified by contorted shales and brecciated sandstones implying post depositional displacement.
• Netafim Formation, which, although found regionally, is absent from the Timna Valley. It conformably overlies the Shehoret Formation and is composed of a Late Cambrian fine-grained quartz arenite with alternating layers of siltstone and claystone sequence, which has a maximum thickness of 22 m.
In the Timna Valley, the upper contact between the Shehoret Formation and the Amir Formation, at the base of the immediately overlying Cretaceous Kurnub Group, is an erosional unconformity, which is estimated to have truncated ~2500 m of Ordovician to Lower Cretaceous sedimentary rocks (Weissbrod, 1981; Weissbrod and Gvirtzman. 1988) representing a stratigraphic gap of ~400 million years. This missing succession is described in the Wadi Araba - Feinan record where it is present.
▸ Cretaceous Succession, comprising the:
• Early Cretaceous Kurnub Group, which is ~200 m thick and was deposited above the regional erosive unconformity spanning a number of erosive events evident elsewhere in the stratigraphic record over the period from the Late Cambrian to Early Cretaceous. It is predominantly composed of quartz arenites, subdivided as follows:
- Amir Formation, up to 37 m of white friable, very fine-grained, subrounded to subangular, moderately well sorted, laminated, cross-bedded sandstone, with rare, randomly distributed coarse grains and granules of quartz. The sandstone commonly has a kaolinitic matrix and is cemented by amorphous silica. Recumbent cross-bedding and ripple marks are evident. Thin layers of variegated micaceous siltstone are found, mainly in the middle part of the unit. The contact with the underlying Cambrian rocks, despite the long hiatus it represents, is a paraconformity and often difficult to pinpoint, whilst the contact with the overlying Avrona Formation is unconformable. In the Timna Valley, it forms white to yellowish cliffs with a dark brown patina, overlying the reddish cliffs of the Shehoret Formation (e.g., Shpitzer, 1992: Beyth et al., 1994).
- Avrona Formation, ~60 m of mainly grey, white and yellowish, medium to fine-grained, thickly bedded and commonly cross-bedded, sub-rounded to rounded quartzose sandstones with abundant thin layers of grit and quartzite pebbles. The sandstone is poorly cemented by calcite. It forms rounded cliffs, often with arches. The unconformable contact with the Amir Formation is irregular and manifested as a lithological change, often overlain by a basal conglomerate. The upper contact with the Samar Formation is mostly unconformable, although locally, white sandstones of the Avrona Formation grade into the variegated siltstones and sandstones of the Samar Formation.
- Samar Formation, which ranges from ~80 to as much as 150 m in thickness, comprises violet, brown and whitish fine-grained sandstone, alternating with variegated lateritic siltstone layers that are mainly found in the lower part of the unit (Weissbrod, 1992). The unit is interpretd to be of fluvial origin, and forms a soft geomorphological unit below the cliffs of the more resistant Judea Group. The upper boundary with the marine carbonates of the Judea Group is interpreted to be mid to late Albian to lower Cenomanian in the late Lower to early Upper Cretaceous, and is most likely unconformable, but may represent a facies change.
- Barak Formation, previously known as the 'Crazy Wall', which has been mapped in a few locations at the northern edge of the Timna Valley, mainly within the Avrana Formation, but also transgressing downward to the Timna Formation. It appears to comprises phreato-volcanic rocks associated with ~107 ±2 to 99 ±2 Ma, Early Cretaceous, basaltic explosive pipes, likely associated with an east-west trending, ~10 x 0.5 km, shallow gabbroic intrusion known in the area. The volcanoclastic nature of the sedimentary rocks, in conjunction with the geometry of the depression it fills, is interpreted to suggest a fossil explosive pipe with possible linear offshoots, refilled with its own ejecta which had undergone limited transport and weathering. Mafic magmatism of this age is widespread in Israel. The following vertical succession has been mapped by Weissbrod et al. (1989), as reported by Segev, Beyth and Bar-Matthews (1992):
i). an up to 13 m thick, reddish, coarse, polymict breccia composed of highly angular local sandstone, granite and dolostone, together with fragmented basalts. Clasts of up to 1.5 m across are predominantly of sandstone, with finer fractions dominated by dolostone and weathered basalt;
ii). an up to 20 m thickness of a green to black, finer breccia, with up to 10 cm wide clasts dominated by weathered alkaline basalt, although the original texture and mineralogy have been obliterated by alteration;
iii). up to 20 m of greenish, grading upwards into yellow, non-stratified, poorly sorted arenite with granule- to gravel-sized clasts of weathered basalt that diminish in abundance upward.
iv). fine-grained sedimentary rocks, including dolostone and chert up to 2 m thick, overlain by palaeosol.
During Late Barremian to Albian times, the southern margins of the Tethys Ocean progressively transgressed southward over the Arabian-Nubian continent in pulses, evidenced by shallow marine sedimentary facies interspersed with terrestrial clastic rocks. Since the Early Cenomanian in the early Upper Cretaceous, the sea onlapped to cover the Timna-Elat region and gradually progressed southwards into Sinai.
• Upper Cretaceous Judea Group, of latest Albian to Coniacian age, is a thick platform carbonatic sequence, composed of four marine formations, as follows, from the base:
- Hazera Formation, which is 120 m thick, and is further subdivided into the: i). lower Hevyon Member, which forms 30 m thick light brown dolostone cliffs, overlying an up to 10 m thick yellow glauconitic-dolomitic-sandstone bed at the base, which, in turn, unconformably overlies the Samar Formation. The overlying, 30 m thick, ii). En Yorqe'am Member, is composed of green marl and clayey limestone rich in bivalves. The iii). Zafit Member grey limestone with chert nodules containing Nerinea gastropods forms a 30 m high cliff. The upper iv). Yotvata Member comprises white to grey, well-bedded limestone in its lower sections, paper limestone at the middle and white limestone containing the ammonite Neolobites Vibrayeanus indicative of the Late Cenomanian. Exposure of this member in the Timna Valley is limited to a small down-faulted block in the Nahal Timna graben. A thin red-brown limonitic-dolostone bed at the top of the member marks the top of the Hazera Formation. During the Late Cenomanian-Early Turonian, deposition in the area took in shallow NE-SW elongated basins (Freund, 1962).
- Ora Formation, which is ~104 m thick, deposited in the largest basin in the Timna-Elat area, located between the coast to the south and the elevated carbonate platform belt in the north. The basin shallowed to a lagoon, variously containing brackish-water with vegetation, or hypersaline conditions depositing dolomite and gypsum. The formation is divided into: i). a lower member, composed of green to grey shales with gypsum veins and thin beds of yellow nodular limestone with Early Turonian ammonites; ii). a middle member, known as the fossiliferous dolostone of the 'Vroman Bank' in the Makhtesh Ramon area; and iii). an upper member, made up of red and green beds of shale, a 5 m thick massive gypsum unit, and thin beds of limestone and dolostone.
- Gerofit Formation, which is ~130 m thick and composed of grey, finely crystallised limestone, precipitated in a shallow marine environment. It commences with limestone, intercalated with green clay, which has been structurally disrupted along the rift margin. This facies is overlain by a middle suite of white chalky limestone, which is, in turn, succeeded by well-bedded, cliff forming, limestone and dolostone with thin beds of chert and chert nodules, and common crustaceaen burrows. This formation floors the plains to the north and west of the Timna Cliffs.
- Zihor Formation (or Eteq Formation south of the Timna Valley ), which is of Late Turonian-Coniacian age and composed of limestone, marl and dolostone rich in fossils such as stromatoporoids and oyster beds.
▸ Cenozoic Succession, comprising the:
• The Young Clastic or Dead Sea Group, deposit in association with rifting related to the Dead Sea Transform. South of the Timna Valley, the Miocene (Garfunkel et al., 1974) or Eocene (Avni et al., 2012) Raham Conglomerate is exposed, comprising a poorly sorted, coarse conglomerate with rounded-angular boulders mainly of the Eocene, Avdat Group carbonate rocks (which is not locally exposed), but lacking magmatic and metamorphic rocks. Three Pliestocene to Holocene fluvial terraces are evident within the Timna Valley (Ginat et al., 2009): i). the high Old River Terraces 30 to 60 m above the present channels; ii). the Middle River Terraces, 10 to 30 m above the current channels; and iii). the younger Lower River Terraces, which are 5 to 10 m above the present channel. Dating of these sediments ranged from >231 000 to 14 000 years ago. In the southern Wadi Araba, to the east of the Timna Valley margin, these young clastics within the Dead Sea Rift are >1000 m thick (Segev, Beyth and Bar-Matthews, 1992).
Structure
Three main structural trends are evident in the region around Timna, namely:
i) Neoproterozoic, folding resulting from the collision of East and West Gondwana between 650 and 600 Ma (e.g., Johnson et al., 2011, and references therein), succeeded from ~600 to 590 Ma by the formation of the up to 200 km wide zone of NW-SE sinistral strike-slip faulting known as the Najd Fault System (see the "Regional Setting" section of the Wadi Araba - Feinan record for more detail.
ii) an east-west transverse fault system crossing the southern Negev and central Sinai from the Gulf of Suez to the Wadi Araba. The southernmost and most prominent of these structures, the Themed (or Tamad) Fault separates the southern Har Amram and the northern Timna Valley blocks a few km south of the Timna Valley southern arm. The Har Amram Block is characterised by intense north-south faulting, whereas the Timna Valley Block is more 'tabular' or intact. The Themed Fault is interpreted to represent a dextral strike-slip structure with an estimated lateral displacement of ~2.5 km (Bartov, 1974 and Garfunkel, 1970). Closer to the Cenozoic Dead Sea Transform and Wadi Araba/Dead Sea Rift, its strike rotates from east west to ENE-WSW. This change in strike is taken to suggest the Themed Fault, which is believed to be a Proterozoic structure, was reactivated during the Miocene by northward movement of the southern block during activation of the Dead Sea Transform. This east-west array parallels the shoreline during deposition of the Lower Palaeozoic sequence.
iii) Dead Sea Transform Fault and related younger structures, which trend NNE-SSW to north-south, and are Cenozoic in age, post-dating both previous trends. The Dead Sea Transform and Dead Sea Rift are related to the break-up of the Arabian-Nubian Shield and separate the Arabia and Africa Plates. This break-up was accompanied by widespread, predominantly basaltic, volcanism that began in the Oligocene, mainly from 30 to 20 Ma, continuing into the Miocene (Meneisy and Keuzer, 1974; Coleman et al., 1977 and Seinitz et al., 1978). Major 'rift only' faulting began after 20 Ma, in the Miocene. Garfunkel (1981) suggested sinistral displacement of ~60 km took place during an initial Miocene phase, followed by another ~40 km during a Plio-Pleistocene event. This was accompanied by a 1 to 2 km uplift of the African Plate along the Wadi Araba, increasing to 3 to 5 km closer to the Red Sea, and even more in the Dead Sea. The greater part of the Dead Sea Transform is marked by a conspicuous 10 to 20 km wide depression to it's east, generally delimited by normal faults, and largely filled with Cenozoic sediments. Movement on this structure continues to the present (Segev, Beyth and Bar-Matthews, 1992).
As detailed above, the Timna Valley has a semi-circular horseshoe shape, cored by a basement high of Proterozoic rocks of the Timna Igneous Complex in the Har Timna, and was truncated to the east by the Dead Sea Transform Fault. The unconformably overlying Lower Palaeozoic sedimentary sequence dips at 5 to 10° radially outward and progressively transgress onto basement up sequence. Whilst Benter and Vroman (1955) described the Timna Valley as a domal structure, Mart and Freund (1977) argued the absence of a radial fault set negated such an interpretation. Segev (1986) suggested instead that the uplifting of the Har Timna Block was the result of reverse faulting normal to the main compressional stress, the direction of which was generally north-south, presumably related to the Najd Fault System, possibly reactivated during the Cenozoic. Within the Har Timna Block, both the north-south to NW-SE Najd and ENE Themed fault systems control the Araba Complex dyke emplacement within the Neoproterozoic Aqaba Magmatic Complex. Uplift and peneplanation occurred during the Lower Cambrian (Garfunkel, 1980), prior to deposition of the Yam Suf Group. However, deposition of the latter group has been interpreted to have taken place in a number of grabens and fault separated tectonic block, consistent with the uplift of the Har Timna Block being the result of a complex of reverse faults. Subsequent uplift during the Palaeozoic and Mesozoic is reflected by the unconformities at the top of the Shehoret Formation and the base of the Avrona Member in the Timna Valley (Weissbrod, 1981) and probably during the Lower Cretaceous to Cenozoic (Beyth and Segev, 1983; Weissbrod et al., 1989).
Mineralisation
Copper mineralisation within the Timna Valley has been found to varying degrees within Neoproterozoic igneous rocks, at various levels through the Cambrian sedimentary sequence, and within Lower Cretaceous sandstones. However, ancient copper production was mainly from the top of the Amir
and base of the Avrona formations in the northwestern Timna Valley, both of which are of Cretaceous age, whilst modern mining activity has concentrated on Cambrian host rocks south of Mount Timna.
Other mineral occurrences are found along the western margins of the Dead Sea Transform, including gold (Bogoch et al., 1993) and manganese, barium and lead mineralisation in southeastern Sinai (Ayalon et al., 1985).
Mineralisation within the Timna Valley and surrounds is distributed as follows:
• Neoproterozoic Crystalline Basement - in which hypogene oxide and supergene sulphide copper mineralisation is common, although none has been found to be economic in either ancient or in modern times (Burgath et al., 1984). Chrysocolla and chalcocite occur in igneous rocks of the basement crystalline complex, but generally very close the contact with the overlying permeable Palaeozoic sedimentary rocks. Zlatkine and Wurzburger (1957) suggested this mineralisation likely originated from downward percolation of copper-rich solutions from the overlying sedimentary rocks which are also mineralised. Wurzburger (1967, 1970) reported hypogene sulphides and supergene oxides within mafic rocks, whilst porphyritic calc-alkaline granite contains chalcopyrite, digenite, chalcocite and covellite, whereas the alkali rhyolitic dykes only contain chaIcocite and covellite. The sulphides have been oxidised to green minerals, mainly paratacamite [Cu2(OH)3Cl2], malachite [Cu2CO3(OH)2] and chrysocolla [CuSi03•2(H20)]. Pyrite, largely oxidised to hematite, occurs in alkaline rhyolitic dykes. However, Beyth, Segev and Ginat (2013) regard these sulphides as the result of a hydrothermal copper and manganese enrichment event that occurred in the final magmatic stage of the Timna Igneous Complex at ~600 Ma. The olivine gabbro of the complex contains pyrrhotite-pentlandite, chalcopyrite and two forms of magnetite. This Ni-Fe-Cu sulphide assemblage is considered to be the result of an early magmatic sulphide segregation and relatively late sulphide crystallisation (Segev, Beyth and Bar-Matthews, 1992).
• Early to Middle Cambrian Timna Formation of the Yam Suf Group, distributed as follows within the principal lithofacies of the Sasgon Member which is the main host to copper mineralisation within this sequence:
- Dolomitic Lithofacies, which is predominantly a sandy dolostone. These dolostones are mainly composed of massive, coarse-grained dolomite, although the lower section is a laminated dolostone. Whilst all of the dolostones deposited within the Timna Basin carry anomalous copper, averaging 600 ppm Cu, a few stromatolitic dolomitic units, mainly in the lower, more laminated parts of the Sasgon Member, host economic stratabound copper mineralisation. These cupriferous dolostones are evident in most of the dolomitic lithofacies outcrops throughout the Timna Valley (Segev, 1980), occurring as 13 to 100 cm thick beds carrying between 0.22% and 1.77%, averaging 0.68% Cu. Significant copper mineralisation within the dolostone rarely occurs as sulphides, and is dominantly hydrated copper chlorides and carbonates (Segev, 1986; Shlomovitch, 1995), predominantly paratacamite [Cu2(OH)3Cl2] (Segev, Beyth and Bar-Matthews, 1992). However, within the massive dolostone, sulphide mineralisation is also present, occurring as small, 0.1 to a few millimetres diameter, metallic to green-coloured, rounded spherules to rod/lens like concretions up to 20 x 5 mm. These spherules and concretions have a copper sulphide core, surrounded by a green envelope of secondary copper minerals which has a sharp contact with the host dolostone. In places, the metallic sulphides have irregular shapes, and are surrounded by a green envelope from which small veins protrude into the host dolostone (Segev, Beyth and Bar-Matthews, 1992).
Within the lower laminated dolomite, mineralisation occurs as thin, undulating laminae, generally <1, but locally up to a few mm thick, mainly composed of paratacamite, alternating with clay and mica-rich laminae. These laminae are cut by thin veins, similar in composition and appearance to the laminae, which commonly crosscut several of those laminae (Segev, Beyth and Bar-Matthews, 1992).
The copper sulphides within the dolomitic lithofacies are predominantly djurleite [Cu1.93S] and covellite [CuS], although solid-solution phases are also common, mainly chalcocite [Cu2S), digenite [Cu1.8S], and anilite [Cu1.75S]. Whilst djurleite dominates, covellite needles are in sharp contact with the djurleite towards the outer sections of the spherules and along cleavage surfaces, taken to indicate a solid-solution transition from djurleite to covellite (e.g., Frueh, 1954, Djurle, 1958, Morimoto et al., 1969, and Morimoto and Koto, 1970). The sulphides all carry ~0.13 to ~2.65 wt.% Ag, which increases with the decline in Cu/S ratio, interpreted to suggest Ag replaces Cu in the sulphide lattice, where it occurs as acentite (Ag2S). Acentite usually accompanies covellite suggesting they are genetically related. Smooth and well preserved voids are evident in the copper sulphide surfaces occasionally filled with gypsum, quartz, K feldspar, calcite, and native copper, suggesting they represent trapped inclusions of the original sediment (Shlomovitch, et al., 1999). The formation of this covellite, digenite and anilite series sulphide assemblage is regarded as typical of a low-temperature regime between 25 and 75°C (e.g., Morimoto and Koto, 1970). The assemblage is unusual, in that there is an absence iron bearing sulphides, particularly pyrite and chalcopyrite. This is taken to indicate deposition of sulphides within an Fe poor regime. Bar-Matthews and Matthers (1990) have shown that the upper temperature range of sulphide deposition at ~50°C could have overlapped temperatures prevailing during the dolomitisation of the host carbonate sequence. The prevalence of the covellite-digenite-anilite sulphide assemblage, all low temperature minerals, is also consistent with their contemporaneously formation.
The dolostones are also enriched in manganese, with 1.7 to 2.8% Mn occurring within the dolomite lattice (Bar-Matthews, 1986, 1987; Segev, 1986, 1992).
The 'green envelopes' encapsulating almost all of the sulphide concretions/spherules described above are composed of copper hydroxides, usually malachite [Cu2(OH)2CO3] and paratacamite, which commonly completely replace the sulphide minerals. However, whilst malachite replaces the sulphides and mimics their shape, paratacamite can form veins that emanate from the rim of the spherules into the surrounding host dolostone, and form horizontal laminae along layers rich in micas and clays. Secondary malachite occurs within the laminae of paratacamite, where it appears to predate the latter. Where quartz and feldspars are enclosed within the sulphide spherules, veins of chrysocolla [Cu2-xSiO5(OH)3•yH2O] are developed cutting the spherules. Chrysocolla development within the sulphides is rare, and restricted to the presence of silicate minerals. Sporadic, micron-sized crystals of Zn-rich mottramite [Pb(Cu, Zn)(VO4)(OH)] are evident within the green envelopes.
Layers of clay are found within the laminated dolostone, including both detrital, mainly kaolinite, and authigenic, mainly illite. Concentrations of between 500 to 4000 ppm Cu occurs within these clays, but is restricted to the authigenic illitic species, where infrared analyses indicate it occupies octahedral sites within the illite crystal lattice.
Shlomovitch et al. (1999) discuss the transition from reducing to oxidising conditions required for the replacement of the sulphide spherules by malachite which occurred in the presence of carbonate ion in solution. Phase diagrams show that this process requires an increase in Eh. In contrast, the subsequent replacement by paratacamite requires the presence of chloride ion, but also the lowering of the pH. This, they suggest, means the replacement of sulphide minerals first occurred during changes from reducing to oxidising in the presence of carbonate ion species in solution. Subsequently, a reduction in the pH is inferred by the presence of chloride solutions, resulting in the formation of paratacamite. Whilst the oxidation of sulphides to malachite was restricted to the sulphide spherules, the paratacamite laminae and veins were developed within the doloston country rock, well beyond the spherules, especially along layers rich in mica and clays. The conversion of 'detrital/weathering clays' to form secondary authigenic clays within the dolostone was followed by adsorption of copper into the authigenic illite lattice.
- Sandy Lithofacies, which is interpreted to represent the insoluble residue following the dissolution and removal of carbonate from the laterally equivalent sandy dolostone that is the 'dolomitic lithofacies'. This unit is the main host to copper mineralisation within the Sasgon Member, with mineable grades averaging 1.1 to 1.5% Cu over thicknesses of 4 to 8 m (Barsky, 1978). Mineralisation is distributed in fractures, veinlets, nodules, joints, along bedding planes and as disseminations throughout the sandy and shaly host-rocks (Bartura and Wurzburger, 1974). The bulk of the copper minerals in the sandy and shaly lithofacies are chrysocolla [CuSi03•2(H20)] and plancheite [Cu8(Si4O11)2(OH)4•H2O] with lesser malachite, pseudomalachite and bisbeeite (Shlomovitch et al., 1999; McLoad, 1959; Milton, 1966; Bartura and Gross, 1966; Wurzburger, 1967, 1970). Sulphides are absent from the sandy and shaly lithofacies.
Both chrysocolla and plancheite are members of a larger family of minerals with variable composition and colour. The usually light blue chrysocolla group minerals are poorly structured and contain 2 to 8 molecules of water (Hindman, 1985). In contrast, the darker turquoise to deep blue-purple colours that characterise the plancheite group correspond to a progressive shift to a relatively more highly structured mineral species, with lesser water molecules and an increase in crystallinity, but very similar Cu:Si ratio. The transition from light to dark colours directly reflects this change in character. Darker blue chrysocolla mainly occurs as cement to the sandstones, whilst light blue chrysocolla is found along fracture planes. Deep blue-turquoise coloured plancheite is found as ellipsoids that range from a few millimetres to a few centimetres in diameter, within developments of chrysocolla. Smaller veins of this plancheite emanate from the ellipsoids cutting across the sandy lithofacies into the dolomitic lithofacies. Petrography shows these copper silicate minerals replace clays, quartz and feldspars, resulting in the surface of the remaining silicate minerals, onto which the chrysocolla and plancheite are deposited, being visibly etched. A secondary generation of chrysocolla with the lightest colour, and the highest number of water molecules, occurs along fracture planes. Other secondary minerals found within the sandy lithofacies include pseudomalachite [Cu5(OH)4PO4] and dioptase [CuSiO3•H2O] together with authigenic fluorapatite [Ca5(PO4)3F] (Bartura and Gross, 1966).
Paratacamite and malachite, which are common in the dolomitic lithofacies, are relatively rare in the sandy and silty lithofacies. These observations are interpreted to reflect the following three stage paragenesis: i). amorphous chrysocolla replaced silicates, principally clays, feldspars and quartz; ii). a transition from an amorphous to a better crystallised, less hydrous phase, specifically the formation of plancheite concretions and veins from the primary chrysocolla; iii). formation of secondary chrysocolla along fracture planes.
These 'oxide' assemblage has been encountered throughout the mineralised interval to as deep as 900 m below surface. This 'oxide' assemblage is interpreted to be the result of oxidation and hydration reactions during and after dolomitisation, whilst the sulphides within the dolomitic lithofacies is taken to precede dolomitisation.
Manganese and phosphate are concentrated in the same unit, mainly occurring as cement to the clastic host, which is a laminated manganese-rich sandstone. Locally, these elements are also present as nodules of manganese or lenses of phosphate. The unit averages 2.83% Mn, although some laminated manganese-rich sandstones contain up to 11% Mn, whilst individual nodules may contain as much as 49% Mn. The average phosphate content of the same rocks ranges from 0 to 5% P2O5, whilst some nodules carry as much as 30% P2O5. This mineralisation is cross-cut by vein-filling fractures usually composed of hollandite and coronadite, which may extend down into the Hakhlil Member, and in some cases even persisting even lower into the Amudei Shelomo Formation. Locally, massive, discordant manganese mineralisation that is horizontally and vertically banded, and concretionary, is associated with iron oxides (Segev, 1986) and is found in kaolinite-rich sandstones of the sandy lithofacies close to a major fault. The kaolinite is interpreted to be the product of intensive kaolinitisation of illites and K feldspars (Segev, Beyth and Bar-Matthews, 1992).
At a number of sites sampled, uranium enrichments of up to 4000 ppm U3O8 were found within some of the manganese assemblages and/or the phosphorites (Segev, Beyth and Bar-Matthews, 1992).
- Shaly Lithofacies, which overlies both the dolomitic and sandy lithofacies, also contains widespread copper mineralisation, similar to that described for the sandy lithofacies. In addition, a range of manganese nodules are locally evident, mainly around Hac Mikhrot on the northern limb of the Timna Valley. These range in size and shape from 1 to ~30 cm diameter spherical, to up to 50 x 15 cm and lenticular. Three of these nodules have been dated, returning ages in the Lower Cretaceous, coeval with local basaltic plugs (Segev et aI., 1992, and have been overprinted by Neogene hydrothermal processes (Segev, Beyth and Bar-Matthews,1992). While there is no direct support for the manganese nodules forming by diagenetic processess during the Lower Palaeozoic, the same authors posit it is still possible that some manganese nodules did form contemporaneously with the Lower Cambrian carbonate hosts and served as an easily available source for manganese when reactive solutions affected the Timna Formation. This would require resetting of Lower Palaeozoic dates during the Cretaceous. Locally, cryptocrystalline fluorapatite is found as small stratabound lenses up to tens of centimetres in length, and as much as 15 cm thick, concentrated in one or two horizons. These lenses commonly carry 400 to 750 ppm U (Segev and Beyth, 1983) - see the Negev Desert Uranium and Phosphate Mineralisation record.
• Early to Middle Cambrian Shehoret Formation of the Yam Suf Group, which overlies the Timna Group, and has been sub-divided as follows:
- Lower Member, which in the Timna Valley includes copper rich horizons, referred to as 'Copper B'. This member is regarded as the equivalent of the Variegated Sandstone Unit in Jordan, which marks the return to terrigenous sedimentation above the marine lagoonal dolostone-limestone-shale package of the Burj Formation (see the Deposit Geology and Copper Mineralisation section of the Wadi Araba Copper District - Feinan record.
In Israel, mineralisation within this unit comprises copper species impregnating brecciated fluviatile sandstones and forming lenses up to tens of centimetres thick with the same mineralogical composition as described above for the 'sandy lithofacies' of the Sasgon Member, i.e., predominantly chrysocolla and plancheite, lacking sulphides. These minerals form an intergranular cement within the host sandstone, filling fractures to form fissure-filling veinlets and occasionally, as small patches/aggregates (Segev, Beyth and Bar-Matthews, 1992).
- Middle or White Member, which is not found in the Timna Valley or to it's north, but is exposed south of Har Amram, a few km south of the Timna Valley southern arm. This member, which comprises fine grained white sandstones, is interpreted to have been deposited in a marine littoral regime. Two 2 to 10 cm thick stratabound copper-rich horizons 4 m apart are made up of lenses that are each several metres long are found in the middle section of this member. These developments include small, 1 to 10 mm spherical nodules, often forming aggregates within the central part of the mineralised horizons. Only a few of these nodules contained primary copper sulphides. In most, cuprite and malachite are the dominant minerals. The most extensive mineralised area within this unit is in Wadi Abu Khusheiba in the eastern Araba Valley (described in the Wadi Araba Copper District record.). Within this unit copper is found as up to 20 cm diameter copper nodules composed of primary chalcocite, bornite and chalcopyrite with secondary cuprite and malachite. Malachite is found as a thin coating on pebbles in the basal conglomerates of this unit, or in the groundmass between these pebbles (Basta and Sunna, 1972). Andesitic and rhyolitic dykes and volcanic breccia occur in close proximity to the chalcopyrite, chalcocite and covellite mineralisation (Burgath et al., 1984). The main differences between the White Member mineralisation and that of the Lower Cambrian stratabound accumulations hosted by the Timna Formation is the dominance of carbonate minerals in the latter. Also at that time the Lower Cambrian sequence was covered by the lower member of the Shehoret Formation which separated the two (Segev, Beyth and Bar-Matthews, 1992).
• Lower Cretaceous Kurnub Group, which hosted the bulk of the copper ores mined in antiquity. Mineralisation of this group is predominantly hosted at the base of the Avrona Formation over a thickness of up to 15 m, and to a lesser extent at the top of the immediately underlying Amir Formation. It is widespread in the western Timna Valley and to a lesser extent on the northern and southern arms of the valley. Similar mineralisation is exposed 4 to 5 km to the south, near Amudei Amram, and to the east of Nahal Roded.
Copper grades within the Avrona Formation decrease upwards, and is preferentially deposited at the top of palaeochannels filled by cross-bedded sandstones (Keidar, 1984). The same author recognised three modes of occurrence of copper mineralisation:
i). replacement of plant debris;
ii). green concretions and nodules that are generally elliptical to spherical with mean diameters of ~3 cm. Both the concretions and nodules have cores of chalcocite and covellite, surrounded by two rings of detrital quartz that are cemented by malachite, lesser atacamite, and iron oxides. The concretions have a well-crystallised core nucleated on plant material which is replaced by copper minerals, with the encapsulating quartz grains increasing outward from the centre. The nodules do not contain these rings of cemented quartz grains. The copper content in the concretions varies from a few to ~44% Cu, with up to 5000 ppm Zn, up to 4700 ppm Pb and up to 26O ppm Ag. Iron oxides, mainly hematite, is closely associated with the copper sulphides, although no clear paragenetic relationship has been elucidated.
iii). impregnation and cementation of sandstones, predominantly comprising malachite, which replaces the original kaolinitic cement, and atacamite.
The extensive development of copper accumulations close to the contact between the Arnir and Avrona formations suggests a stratigraphic and/or a lithologic control. However, copper mineralisation is also often emplaced in small fractures and faults, implied to indicate a structural control. North of Nahal Mangan, which is on the north eastern tip of the Timna Valley, where it is cut by the Dead Sea Transform Fault and flows into the Wadi Araba, mineralisation was emplaced along faults cutting both the Cretaceous Amir and Cambrian Shehoret formation. Numerous fossilised trees replaced by hematite and goethite are found at the base of the Avrona Formation in the same part of the section enriched in copper (Ayaion et al> 1985). Mineralisation in this part of the sequence is characterised by high Fe, associated with high concentrations of Pb, Cu and Zn with lesser Mo, Ni, Co and U. Some of the Pb within the ferruginous trees occurs as wulfenite [PbMoO4].
This record has largely been descriptive. However, Beyth, Segev and Ginat (2013) interpret the following periods/stages of copper deposition in the district, after Beyth (1987); Segev and Sass (1989); Shlomovitch et al. 1999; Segev and Beyth (2011):
i). Hydrothermal copper and manganese were emplaced by the final magmatic stage of the Timna Igneous Complex at ~600 Ma. Copper occurs as the sulphide minerals chalcopyrite, digenite, chalcocite and covelite, which have subsequently been parly oxidised to paratacamite, malachite and chrysocolla (Wurzburger, 1967).
ii). Diagenetic deposition of copper and manganese in Cambrian lagoonal carbonates of the Sasgon Member at ~520 Ma, mainly as djurleite and covellite with minor chalcocite, digenite and anilite containing silver as a significant minor element (Shlomovitch et al., 1999). This may have resulted from leaching of the mineralised basement Timna Igneous Complex. Diagenetic manganese nodules composed of pyrolusite and hollandite and uranium–enriched, stratabound manganese were also deposited in the same carbonates (Bar-Matthews, 1987).
iii). A Lower Cretaceous phreatovolcanic event related to the Barak Formation mafic intrusions circulated thermal brines which dissolved Cu and Mn enriched dolostone from the Sasgon Member, leaving coronadite nodules after the oxidation of manganese (Bar-Matthews, 1987). Copper was transported up-section through faults and highly permeable country rocks to the Cretaceous upper Amir and lower Avrona formations and precipitated as sulphides which were subsequently partially oxidised to malchite and atacamite at ~100 Ma (Keidar, 1984).
iv). Thermal brines circulating through the Dead Sea Transform related faults and fractures led to the dissolution (karstification) and enriched of the dolomitic lithofacies of the Sasgon Member through volume reduction (Weber et al., 2009). As a result, after this dissolution of the dolostone lithofacies, the insoluble residue, the sandy lithofacies, was enriched in copper and phosphate-uranium mineralisation from ~14 Ma to the present. The current Timna Valley ground water comprises thermal (T = ~30°C) Ca-chloritic brines with extreme high Ca/Mg ratios, low 87Sr/86Sr isotopic ratios and enrichment in copper, iron and manganese (Beyth et al., 1983). These brines were most probably involved in the epigenetic alteration of the Timna Igneous Complex (Marco et al., 1993 and Beyth et al., 1997) and had been involved in the karstification and mineral enrichment of the overlying sequence. The main late copper mineralization is concentrated within the sandy and shaly lithofacies as epigenetic secondary minerals in the paragenetic order: chrysocolla → plancheite → pseudomalachite → dioptase (Shlomovitch et al., 1999).
Whilst this paragenesis is largely consistent with the observations recorded, it is unusual for a sediment hosted copper deposit. An alternative interpretation might involve an initial stage involving deposition of sulphides from an oxidised solution which permeated the thick, widespread oxidised aquifer represented by the sandstones of the Palaeozoic and structurally connected overlying Cretaceous sequences. The sedimentary architecture of this part of the Arabian-Nubian shield would have allowed the migration of such fluids in much the same way as it favoured distribution of hydrocarbons a little to the east. As in most sediment hosted deposits and belts worldwide, when circulating copper bearing oxidised fluids encounter a reductant, sulphides are precipitated. Reductants include sour (i.e., H2S bearing) gaseous or liquid hydrocarbons; sulphate rich evaporites; and carbonaceous matter such as black/bituminous pyritic sediments containing buried vegetation in various stages of decomposition. Remnant hydrocarbons are not represented locally but may have once done so. Pre-dolomitisation sulphate rich evaporites are hinted at within the lagoonal facies of the Cambrian Sasgon Member dolostones, by remnant pockets of gypsum encapsulated within the now almost entirely dolomitised facies. This dolostone is the only significant host to copper sulphides in the Cambrian sequence. The Cretaceous Arnir and Avrona formations also contain sulphides in a section characterised by vegetation layers, containing fossil tree beds, although subsequent widespread oxidation has totally removed any carbonaceous matter.
Hosts to sediment hosted copper mineralisation in the district span a wide time range, which might be interpreted to reflect multiple pulses of mineralisation, or more likely a single widespread mineralising event during the Cretaceous, accompanying the extensional, mantle sourced magmatism of the period, as is also common in sediment hosted copper districts worldwide. Such fluids may have been complemented by dissolution of copper from the Timna Igneous Complex basement.
However, this sulphide mineralisation has been swamped and all but obliterated by a late extensive pulse of oxidised fluid which progressively replaced sulphides (crystals, aggregates and nodules) with copper carbonates in the carbonatic beds and with copper silicates in the silicate rich units. This same event appears to have also dissolved primary copper mineralisation and redistributed it in aquifers as predominantly copper silicates with a mineralogy very similar to that seen in secondary exotic copper deposits such as Exotica, Mina Sur in Chile and the supergene enriched sediment hosted mineralisation at Maroochydore copper deposit in Western Australia. This mineralisation is characterised by deposition in the upper section of less permeable 'floors' below thick oxidised arenites.
For more detail and discussion see the Evolution and Discussion section at the end of the Wadi Araba Copper District record.
Production and Resources
Based on the tonnage of slag remaining in the Timna Valley, it appears that whilst it was a significant producer by the standards of antiquity, the area most likely only yielded several hundreds to more than a thousand tonnes of copper to the 7th century CE.
Mining between 1959 and 1976, rejuvenated on a smaller scale between 1980 and 1985, produced between 5000 and 14 000 tonnes of contained copper per annum from an original reserve of ~11 Mt at an unspecified grade (US Bureau of Mines, Mineral Trade Notes Nov 1959).
Remaining resources quoted in 2007 (Cox et al. 2007 USGS Open File Report 03-107) were 18.1 Mt @ 1.6% Cu for ~0.29 Mt of contained copper.
See the history section at the beginning of the record for background.
The most recent source geological information used to prepare this decription was dated: 2013.
This description is a summary from published sources, the chief of which are listed below. © Copyright Porter GeoConsultancy Pty Ltd. Unauthorised copying, reproduction, storage or dissemination prohibited.
Timna Mines (modern)
|
|
|
Beyth, M., Segev, A. and Ginat, H., 2013 - Stratigraphy and Structure of the Timna Valley and Adjacent Ancient Mining Areas: in Ministry of Energy and Water Resources, Geological Survey of Israel, Report GSI/15/2013, 35p.
|
Cavanagh, M. Ben‑Yosef, E. and Langgut, D., 2022 - Fuel exploitation and environmental degradation at the Iron Age copper industry of the Timna Valley, southern Israel: in Nature Scientific Reports 2022 15p. /doi.org/10.1038/s41598-022-18940-z.
|
Lehner, J.W., Dumitru, I.A., Buffington, A., Dollarhide, E., Nathan, S., Paulsen, P., Young, M.L., Sivitskis, A.J., Wiig, F. and Harrower, M.J., 2023 - Iron Age Copper Metallurgy in Southeast Arabia: A Comparative Perspective: in Ben-Yosef, E and Jones I.W.N. (Eds.), 2023 And in Length of Days Understanding (Job 12:12) Springer Nature Switzerland AG Interdisciplinary Contributions to Archaeology pp. 1391-1417. doi.org/10.1007/978-3-031-27330-8_59 .
|
Segev, A., Beyth, M. and Bar-Matthews, M., 1992 - The Geology of the Timna Valley with Emphasis on Copper and Manganese Mineralization - Updating and Correlation with the Eastern Margins of the Dead Sea Rift: in Ministry of Energy and Water Resources, Geological Survey of Israel, Report GSI/14/92, 36p.
|
Shlomovitch, N., Bar-Matthews, M., Segev, A. and Matthews, A., 1999 - Sedimentary and epigenetic copper mineral assemblages in the Cambrian Timna Formation, southern Israel: in Israel Journal of Earth Science, v.48, pp. 195-208.
|
Porter GeoConsultancy Pty Ltd (PorterGeo) provides access to this database at no charge. It is largely based on scientific papers and reports in the public domain, and was current when the sources consulted were published. While PorterGeo endeavour to ensure the information was accurate at the time of compilation and subsequent updating, PorterGeo, its employees and servants: i). do not warrant, or make any representation regarding the use, or results of the use of the information contained herein as to its correctness, accuracy, currency, or otherwise; and ii). expressly disclaim all liability or responsibility to any person using the information or conclusions contained herein.
|
Top | Search Again | PGC Home | Terms & Conditions
|
|