Los Bronces - Disputada, Rio Blanco - Andina, Sur Sur |
|
Chile |
Main commodities:
Cu Mo
|
|
 |
|
 |
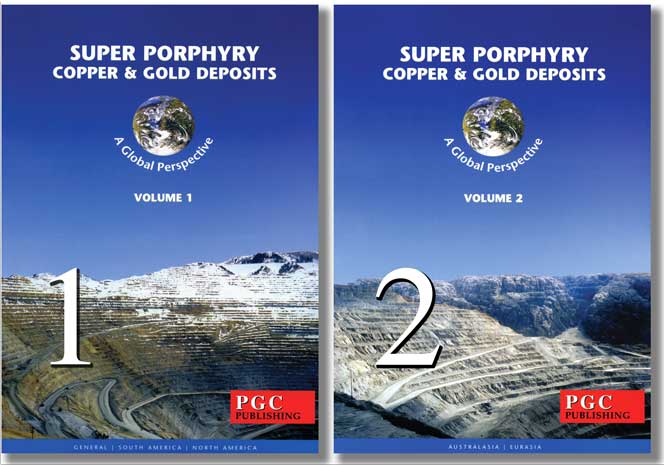 |
Super Porphyry Cu and Au

|
IOCG Deposits - 70 papers
|
All papers now Open Access.
Available as Full Text for direct download or on request. |
|
 |
Los Bronces-Rio Blanco is one of three giant Miocene to Pliocene Cu-Mo breccia/porphyry copper deposits in central Chile. It is located at an altitude of from 2700 to 4100 m, some 45 km (70 km by road) northeast of Santiago de Chile.
(#Location: Los Bronces - 33° 9' 1"S, 70° 16' 47"W; Andina - 33° 9' 5"S, 70° 15' 19"W).
The deposit is exploited by both the Anglo American Sur (previously Disputada) Los Bronces mine (~0.225 Mt of Cu per annum in 2011) and by the Codelco Andina Division Rio Blanco operation (~0.20 Mt Cu per annum in 2011). The Sur-Sur pit is a southern extension of the Rio Blanco mine.
These two mining operations are both part of the Miocene to Pliocene Río Blanco-Los Bronces porphyry system in which mineralisation extends for at least 5 km from north to south, and up to 2 km from east to west, but in 2015 was open at depth and laterally.
Regional Setting
The Rio Blanco-Los Bronces district is located on the continental margin of South America, underlain by a largely concealed Neoproterozoic basement composed of rocks with metamorphic ages of almost 1 Ga. These rocks are part of the Chilenea Terrane, the last of a collage of at least two late Mesoproterozoic to Neoproterozoic exotic slivers that, during the late Cambrian to Early Devonian Famatinian Tectonic Cycle, were progressively accreted to the Proterozoic Pampia terrane to the east. The latter was, in turn, amalgamated with the Rio de la Plata Archaean craton further to the east in the late Mesoproterozoic (Ramos, 2008; 2010). This Famatinian cycle was accompanied by subduction and magmatism that progressively migrated to the west. The Chilenia terrane is masked by a Lower Palaeozoic foreland wedge, although minor erosional windows through this cover reveal the older schists and gneisses (Ramos and Basei, 1997).
This accretionary stage was followed by the Gondwana Tectonic Cycle, which commenced in the Mid-Devonian and continued to the Late Permian. At the close of the Famatinian Cycle, subduction had retreated to near the current coastline, the subducting plate dipping east below the now amalgamated Chilenia terrane, accompanied by the development of a suprasubduction magmatic arc, a complex sequence of both 'I' and 'S' type granitoids and a growing fore-arc accretionary prism (Mpodozis and Cornejo, 2012).
The Pre-Andean Tectonic Cycle that followed, persisted from the latest Permian to earliest Jurassic and marks a 55 m.y. hiatus in orogenic magmatism between the amalgamation of Gondwana/Pangea and the commencement of its break-up. This pause favoured the accumulation of heat in the upper mantle, melting of the lower crust, the generation of large volumes of 'S' and 'A' type silicic magma, granitoid and mantle related mafic and felsic intrusion and volcanism which lasted from the middle Triassic to earliest Jurassic (240 to 190 Ma). It also led to the onset of extensional tectonics in the upper crust, producing rift sedimentation intercalated with decreasing volumes of bimodal magmatism (Charrier et al., 2007; Mpodozis and Cornejo, 2012).
The Andean Tectonic Cycle lasted from the late Early Jurassic to the present and corresponds to the break-up of Gondwana, with the eventual renewal of subduction activity. The associated sequence comprises continental sedimentary rocks of Late Jurassic age, and an Early Cretaceous succession of fossiliferous marine limestones and calcareous sandstones, deposited in a back-arc basin setting in the east, and a Late Cretaceous volcanic, mainly pyroclastic, continental arc to the west (Gana and Wall, 1997; Fuentes et al., 2002).
Rock units in central Chile that are associated with the evolution of the South American margin are distributed in three main north-south structural domains, from west to east, the Coastal Cordillera, the Central Depression and the Principal Cordillera. The Coastal Cordillera is composed of late Palaeozoic to Triassic intrusive and metamorphic rocks on its western flank and east dipping Jurassic to Early Cretaceous volcanic, intrusive and sedimentary rocks in the eastern half, which persist to the middle of the Central Depression. The Central Depression is a longitudinal valley, that varies from almost 0 to >50 km in width, flanked by the two cordillera, and filled by recent unconsolidated sedimentary and pyroclastic deposits. Its eastern contact with the Principal Cordillera is defined by high-angle, west-vergent reverse faults (Cembrano et al., 2007; Armijo et al., 2010).
The Principal Cordillera has been further subdivided into a western and an eastern domain. The eastern domain comprises Jurassic to Early Cretaceous back-arc basin sedimentary rocks, which are strongly deformed, and constitute part of the Miocene Aconcagua fold and thrust belt (Ramos, 1996). The western (Chilean) domain is composed of Eocene to Pliocene volcanic rocks with Miocene to Pliocene intrusive rocks. This Miocene to Pliocene intrusive magmatism is closely related to the formation of the porphyry Cu-Mo deposits of central Chile, and were emplaced during the opening and subsequent inversion of the intra-arc Abanico volcano-tectonic basin, which extends as far west as the middle of the Central Depression. This basin is reflected by the 30 to 40 km wide, north-south trending, band of Eocene to Pliocene rocks in the western domain, that have been grouped into the late Eocene to early Miocene syn-extensional Abanico Formation, and the early to late Miocene syn- to post-inversion Farellones Formation (Charrier et al., 2002, and references therein). Both the Rio Blanco-Los Bronces and El Teniente (80 km to the south) porphyry Cu-Mo deposits lie within the core of this basin.
Abanico Formation - In the Rio Blanco-Los Bronces district, this formation represents the sequence deposited in the extensional phase of the intra-arc Abanico basin during the late Eocene to early Miocene (Thomas, 1953; Aguirre, 1960; Charrier et al., 2002; Piquer et al., 2010; 2015). They are the oldest Tertiary rocks in the Andes of central Chile, and comprise andesitic and basaltic-andesitic lava flows and volcaniclastic deposits, intercalated with pyroclastic and sedimentary rocks (Charrier et al., 2002). They also contains several coeval subvolcanic intrusions (Vergara et al., 2004). Three facies are recognised, specifically lava flows, pyroclastic deposits and volcano-sedimentary accumulations. These facies are highly discontinuous and commonly fault bounded, although both the pyroclastic and volcano-sedimentary intercalations are more common and thicker toward the top. The pyroclastic and volcano-sedimentary intercalations have a combined thickness of up to 800 m, whilst the minimum thickness of the entire Abanico Formation is estimated at ~5000 m (Piquer et al., 2015).
Geochemical and isotopic signatures of these rocks suggest a tholeiitic differentiation trend and primitive, mantle-like isotopic signatures (Nystrom et al., 1993). Several 40Ar/39Ar whole-rock and plagioclase ages for Abanico Formation rocks in the western Rio Blanco-Los Bronces district, are all between 34 and 22 Ma (Gana and Wall, 1997; Fuentes et al., 2002; 2004). A pyroclastic flow from the thickest and most continuous intercalation, was dated at 25.06 ±0.18 Ma (U-Pb in zircon).
Further to the north of the Rio Blanco-Los Bronces district, a broadly equivalent 33 to 18 Ma sequence is referred to as the Los Pelambres Formation.
Three main north striking, high-angle fault systems near the margins of the Abanico Basin, the Infiernillo and Pocuro-San Ramon faults in the west and El Fierro fault in the east, have been interpreted to represent inverted basin-margin normal faults that were active during extension (Fock et al., 2006; Farías et al., 2010). The unconformity between the Abanico Formation, interpreted to have been accumulated on top of a previously deformed and eroded Mesozoic sequence during the opening of the Abanico Basin, is marked by a hiatus of ~37 Ma on both the western and eastern margins of the basin (Gana and Wall, 1997; Charrier et al., 2002; Piquer et al., 2015).
Salamanca Formation, which is mapped to the north of Rio Blanco-Los Bronces, overlying the Abanico Formation with angular unconformity. It comprises continental andesitic lavas and andesitic to dacitic volcaniclastic rocks with intercalated rhyolite, and has maximum and average thicknesses of 2500 and ~1300 m, respectively (Rivano, 1995). It has been dated at 30.1 to 11.4 Ma (whole-rock K-Ar; Hollings et al., 2005). The younger Farellones Formation unconformably overlies the Salamanca and older formations, but is locally conformable with the Abanico Formation, as in the Rio Blanco-Los Bronces area, where the Salamanca Formation is not mapped (Godoy et al., 1999; Charrier et al., 2002; Nyström et al., 2003).
Farellones Formation - This unit was deposited during and after the inversion of the Abanico basin, over the period from the early Miocene to early Pliocene. It can be traced over a north-south interval of ~400 km (Hollings et al., 2005), and is composed of up to 3500 m of andesitic to rhyolitic lava flows, minor pyroclastic and volcaniclastic deposits, with very limited sedimentary intercalations in the western Principal Cordillera (Thomas, 1953; Aguirre, 1960; Klohn, 1960; Thiele, 1978; Vergara et al., 1988). In contrast to the Abanico Formation rocks, which have typically been subjected to tight, asymmetric folding, the Farellones Formation sequence is subhorizontal or gently folded. However, there is some evidence of progressive unconformities and syn-tectonic deposition in the transition from the Abanico Formation into the basal section of the formation (e.g., Charrier et al., 2002; Fock et al., 2006), where rocks have been dated at 21.6±0.2 and 20.1±0.3 Ma (40Ar/39Ar on plagioclase; Aguirre et al., 2000) ~12 km to the south. In the Rio Blanco-Los Bronces district, the formation has been dated at 21 to 18 Ma (Fuentes et al., 2002), whilst the andesitic lava flows hosting the Rio Blanco-Los Bronces intrusive suites returned ages of from 17 to 16 Ma (U-Pb in zircon; Deckart et al., 2005). K-Ar and U-Pb ages show plutonic activity in the Rio Blanco-Los Bronces district was continuous throughout the early to middle Miocene, and was broadly coeval with Farellones Formation volcanism (Warnaars et al., 1985; Deckart et al., 2005; 2010). The Miocene volcanic and plutonic units were then cut by late Miocene to early Pliocene subvolcanic porphyries and hydrothermal breccias that are related to the formation of the Rio Blanco-Los Bronces porphyry Cu-Mo cluster (Warnaars et al., 1985; Serrano et al., 1996; Vargas et al., 1999; Deckart et al., 2013). The emplacement of this subvolcanic magmatic-hydrothermal complex occurred between 7 and 4 Ma (Deckart et al., 2013).
Three well-defined members have been defined in the commonly flat lying Farellones Formation:
i). a lower pyroclastic sequence deposited over Abanico Formation rocks, punctuated by progressive unconformities. This member, dated at 22.7±0.4 and 21.73±0.27 Ma (Piquer et al., 2015) has a maximum thickness of 600 m in the north and west, thinning to the south and east until it finally pinches out a few kilometers north of the Rio Blanco-Los Bronces deposit.
ii). a middle member composed of andesitic lava flows with minor, lenticular volcanosedimentary intercalations, is the stratigraphic level at which the Rio Blanco-Los Bronces porphyry cluster was emplaced. It is up to 800 m thick, unconformably overlies the lower pyroclastic sequence, and has been dated at ~17 Ma at the Rio Blanco deposit (Deckart et al., 2005), and nearby at 16.88±0.05 Ma (Piquer et al., 2015).
iii). an upper pyroclastic sequence, intercalated with andesitic lava flows, that has a minimum thickness of 600 m, and conformably overlies the intermediate member. Its minimum age is determined by crosscutting 10.7±0.6 Ma andesitic dykes (K-Ar in whole rock; Rivera and Navarro, 1996, unpub. report for CODELCO, cited in Piquer et al., 2015).
Plutons and Porphyry Intrusions - Miocene intrusions are concentrated into two belts within the inverted Abanico Basin, on its eastern margin and central core respectively. Only minor subvolcanic bodies are exposed between these two defined intrusive belts, emplaced along NE-striking faults, with compositions ranging from basaltic-andesitic to rhyodacitic (Piquer et al., 2015).
The first of these two belts, on the eastern margin of the basin, occurs as a minor, near north-south corridor, which is spatially related to the El Fierro fault and other parallel, north-south trending reverse faults.
The main belt is located in the central part of the basin, ,and in the Rio Blanco area, is represented by a complex of intrusions that are exposed over a rhombic shaped area with dimensions of ~16 x 22 km, where is is known as the Rio Blanco-San Francisco batholith, and a narrower NNW-SSE trending arm to the north, the Rio Colorado plutonic complex. The shape and margins of these batholiths are strongly controlled by NNW-striking, west dipping high-angle faults with composite reverse and sinistral strike-slip movements, and by a NE-SW fault set. The Rio Blanco-Los Bronces deposits overlap the southern margin of the Rio Blanco-San Francisco batholith and the enclosing Farellones Formation.
• Rio Blanco-San Francisco batholith, which covers an area of >200 km2, and is bounded by NNW and NE trending faults, intruding volcanic rocks of the Abanico Formation and the lower and middle members of the Farellones Formation. In several locations, the roof of the batholith is preserved as a subhorizontal intrusive contact with the overlying Farellones Formation, which covers parts of the batholith, obscuring its complete extent. The western and eastern batholithic contacts have local steps and variations in orientation, but generally dip at ~30° outward.
Major element chemical analyses of samples of the lavas and plutons of the San Francisco Batholith reveal them to be medium to high K20 calc-alkaline in composition (Serrano et al., 1996).
On the basis of lithology, petrography and age, 14 different facies have been recognised within this composite batholith, divided into a number of groupings.
i). The oldest are of early Miocene age and comprise coarse grained, equigranular granodiorites, quartz monzonites (>20.1 Ma, K-Ar in hornblende; Warnaars et al., 1985), diorites and syenogranites (16.3±0.15 Ma, U-Pb in zircon; Piquer et al., 2015), which are coeval with the lower and middle members of the Farellones Formation.
ii). A set of younger, middle Miocene equigranular to coarse porphyritic plutons of dioritic, monzonitic, quartz monzonitic and granodioritic composition that cut the previous intrusions. They range from 14.7±0.1 (U-Pb in zircon; Deckart et al., 2010) to 8.16±0.45 Ma (U-Pb in zircon; Deckart et al., 2005), and are coeval with early uneconomic phases of hydrothermal alteration and mineralisation in the district (15 to 8 Ma, 40Ar/39Ar ages of sericite, biotite and hypogene alunite; Toro et al., 2012). Some of these plutons (e.g., the Rio Blanco and Cascada granodiorites, dated at 11.96±0.40 and 8.40±0.23 Ma, respectively; U-Pb in zircon, Deckart et al., 2005), host part of the Cu and Mo mineralisation of the Rio Blanco-Los Bronces cluster. The Cascada granodiorite is restricted to the mine area, but the Rio Blanco granodiorite extends for at least 6 to 7 km to the NNW of the deposit cluster (Piquer et al., 2015).
iii). A set of subvertical andesitic dykes of middle Miocene age that are coeval with the plutons above, and have both NE and NW preferred trends. These dykes have been dated at 11 to 10 Ma (Rivera and Navarro, 1996, unpub. report for CODELCO) and 9.23±0.33 Ma (U-Pb LA-ICP-MS zircon age; Piquer et al., 2015). The latter is from a dyke that cuts sericite alteration accompanying tourmaline, specular hematite, quartz and sulphide veining associated with early sub-economic mineralisation that is ~3 Ma younger than the main Rio Blanco-Los Bronces cluster deposits. Piquer et al. (2015) suggest this early event may be contemporaneous with unproductive hydrothermal centres elsewhere in the district, as at San Manuel and El Plomo (Toro et al., 2012).
iv). A Late Miocene to early Pliocene subvolcanic complex of dacitic to rhyolitic porphyries, diatremes and coeval hydrothermal breccias that are the youngest intrusions in the composite batholith, dated at 7.12±0.19 and 4.69±0.23 Ma (U-Pb in zircon; Deckart et al., 2013). The mineralisation at the Rio Blanco-Los Bronces cluster is temporally and genetically related to a suite of porphyries and breccias of this age range (Serrano et al., 1996; Vargas et al., 1999; Deckart et al., 2005). However, other isolated early Pliocene rhyolitic porphyries are found along major faults at least 7 to 11 km distal to the ore deposits where a 4.69±0.22 Ma (U-Pb in Zircon; Piquer et al., 2015) dyke is found, identical to the La Copa rhyolitic complex associated with the Rio Blanco-Los Bronces cluster deposits (4.69±0.23 Ma, U-Pb in zircon; Deckart et al., 2013).
• Rio Colorado plutonic complex, is a less extensive, NNW trending polyphase composite intrusive complex to the north of the Rio Blanco-San Francisco batholith. Some 7 intrusive facies are recognised, all with the same NNW trend, most likely reflecting the Matancilla fault system which straddles the intrusive complex. Limited dating of a coarse grained, equigranular granodiorite gave an age of 21.76±0.53 Ma (U-Pb in Zircon; Piquer et al., 2015), coeval with the lowermost pyroclastic flows of the Farellones Formation. A dacitic breccia from a suite of younger amphibole and biotite-bearing dacitic and rhyolitic porphyries that cut these granodiorites yielded an age of 18.4±1.4 Ma (K-Ar in whole rock (Munizaga and Vicente, 1982).
• Eastern intrusive belt, which comprises a series of stocks, dykes and sills of equigranular to coarse porphyritic plutons of dioritic and granodioritic compositions which show a strong spatial relationship to the north-south El Fierro and Alto del Juncal faults that are interpreted to be related to the marginal structures of the Abanico Basin. These intrusions are, in turn, cut by andesitic, dacitic and rhyodacitic subvolcanic porphyries. Middle to late Miocene ages of 11.53±0.19 Ma age for a granodiorite, and 12.28±0.15 Ma for a diorite were obtained from the northern part of the belt (U-Pb in zircon; Montecinos et al., 2008).
The Rio Blanco-Los Bronces district is located in a major transition zone between two different segments of the Andean subduction system, to the south and north, which developed in the late Cenozoic (Mpodozis and Ramos, 1989; Piquer et al., 2015). The Southern volcanic zone is associated with a normal subduction angle and active volcanism. To the north is the Chilean flat slab, characterised by a widening of the orogenic system and the absence of active volcanism (Kay et al., 1999). The transition zone broadly coincides with the subduction of the aseismic Juan Fernandez ridge, a buoyant, trench normal, linear bulge in the subducting plate that causes it to flatten under the continental plate, such that the edge of the asthenospheric wedge has migrated substantially inland (Yañez et al., 2002; Hollings et al., 2005).
Geology, Mineralisation and Alteration
Basement to the Tertiary volcanic package in the Rio Blanco-Los Bronces district, is exposed in the easternmost part of the district, in the footwall of the north-south trending, west dipping El Fierro fault, where it comprises continental sedimentary rocks of the late Jurassic Rio Damas Formation, overlain by the Early Cretaceous Lo Valdes or its equivalent San Jose Formation, made up of fossiliferous marine limestones and calcareous sandstones. Mesozoic rocks are also exposed ~10 km to the west of the deposits, in the footwall of the north-south trending, east dipping Infiernillo fault, where they comprise volcanic, mainly pyroclastic, continental rocks of the Late Cretaceous Lo Valle Formation (Gana and Wall, 1997; Fuentes et al., 2002).
Following deformation, uplift and erosion, these basement rocks were discordantly overlain by the late Eocene to early Miocene Abanico Formation andesitic and basaltic-andesitic lava flows and volcaniclastic deposits, intercalated with pyroclastic and sedimentary rocks. These were discordantly followed by the early Miocene to early Pliocene Farellones Formation andesitic to rhyolitic lava flows, minor pyroclastic and volcaniclastic deposits, as described above.
These late Eocene to early Pliocene volcanic rocks were intruded by the extensive San Francisco Batholith, which in part hosts the Los Bronces-Rio Blanco ore deposit along its southeastern margin, with the remainder of the mineralisation within the intruded Miocene volcanics of Farellones Formation.
In this area, the Miocene volcanics of the Farellones Formation comprise 700 to 1200 m of sub-horizontally dipping andesitic lavas, some of which occur as large stoped blocks of these lavas occur up to 500 m below the generally sub-horizontal contact within the batholith. Within the immediate Rio Blanco-Los Bronces district, the San Francisco Batholith is a multi-phase intrusive with compositions including quartz-diorite, granodiorite, quartz-monzonite and quartz-monzodiorite with lesser granite and syenite. The three main phases of the batholith at Los Bronces-Rio Blanco are the Rio Blanco granodiorite, Cascada granodiorite and Diorite, dated at 11.96±0.40, 8.4±0.23 and 8.19±0.16 Ma respectively, all of which preceded the main mineralising events (Deckart et al., 2005).
The main Los Bronces and Rio Blanco ore zones occur within and below a ~1.75 km wide, NNW trending roof pendent of andesite, interpreted to belong to the Farellones Formation, flanked by granodiorite of the San Francisco Batholith.
The structural trends in the three main deposit areas is variable. The Sur-Sur Tourmaline breccia is aligned along a north-south trending structure, the Rio Blanco Fault, which is cut by a 60° striking dextral strike-slip fault systems in the Sur Sur open pit. In the Rio Blanco underground mine, a major 150° fault trend with unknown sense of movement has been identified. The latter is also mapped in the Sur-Sur mine. However, only the NE striking faults show evidence of postmineral reactivation (Skarmeta et al., 2004). Both the NW and NE faults were active during intrusion and mineralisation, as they control the geometry of intrusive bodies and Cu isograds. The frequency of the NW and NE trending faults in the Sur-Sur Tourmaline breccia varies along strike and is proportional to the fracture density, with zones of the most intense fracturing corresponding to high copper grade intervals, high frequency of cross cutting veins and an abundance of hydrothermal mineral cement in the breccia. The converse is true. Silva and Toro (2009) noted that mineralisation and alteration in the Los Bronces mine was syntectonic, based on the geometry and alignment of hydrothermal minerals. According to these authors, fluids were channeled by an NNW-striking structural corridor. Based on 40Ar/39Ar ages from syntectonic biotite crystals, they concluded that this fault zone was active at 7 to 6 Ma.
The hydrothermal history of the Rio Blanco-Los Bronces complex is considered to have commenced during the late Miocene, with development of an extensive alteration system, entirely within granitoid rocks, characterised by actinolite-magnetite dominant assemblages, with mineralogically similar veins and breccia cements, but no economic Cu-Mo mineralisation (Skewes et al., 1994, 2003).
A subsequent event resulted in deposition of ~50% of the Cu ore in the deposit, which occurs as breccia-matrix, stockwork and disseminated mineralisation in a zone of intense potassic alteration, characterised by secondary biotite that formed during the emplacement of biotite-rich breccias of the Rio Blanco breccia complex (Serrano et al., 1996).
Following a period of uplift and erosion, a further event characterised by younger tourmaline-rich breccia pipes, mainly in the Sur-Sur and Los Bronces mines, accompanied the introduction of the other 50% of the Cu in the deposit complex. Clasts within the tourmaline breccias were sericitised during the emplacement of these breccias, later than, and independent of, the earlier potassic alteration (Serrano et al., 1996).
After further uplift and erosion, early Pliocene porphyries were emplaced as subvolcanic plugs, domes, diatremes and extrusions, both within and peripherally to the earlier zone of biotite breccias, potassic alteration and tourmaline breccias. These porphyries are only locally weakly altered and mineralised and disrupt the earlier orebodies (Serrano et al., 1996).
The history of alteration, brecciation, intrusion and mineralisation is as follows:
• Actinolite-magnetite alteration and breccias - The hydrothermal evolution of the Rio Blanco-Los Bronces complex is interpreted to have commenced with development of an extensive alteration system, characterised by disseminated hydrothermal actinolite ±magnetite ±titanite ±plagioclase, narrow veins of actinolite±magnetite with selvages in which secondary actinolite ±magnetite ±clinopyroxene ±titanite ±plagioclase replaced the original igneous minerals, and by breccias with a matrix assemblage of magnetite ±clinopyroxene ±actinolite± plagioclase, largely within granitoid rocks (Skewes et al., 1994, 2003). These breccias, veins and disseminated alteration assemblages are iron-rich, but sulphur and sulphide poor. Fluid inclusion data suggest these breccias, veins and disseminated alteration zones are related to circulation of highly saline fluids that were at high-temperatures, but were not boiling (Skewes et al., 1994). A range of observations suggest this alteration developed at relatively great depths of from >6 to 3 km (Serrano et al., 1996).
This is the most widely distributed alteration type, and is developed within the plutons of the San Francisco Batholith only, surrounding the subsequently developed potassic alteration zone that hosts the deposit (Serrano et al., 1996). No dates relating to this alteration have been encountered.
• Biotite breccias and potassic alteration - This zone represents the earliest economic mineralising event and hosts the high-grade disseminated and stockwork mineralisation, affecting both the granodiorite pIutons of the San Francisco Batholith and the andesitic lavas that they intrude. It resulted in the replacement of original igneous minerals by biotite ±magnetite ±sulphides and addition of K feldspar, as well as the formation of stockwork veins with biotite ±K-feldspar ±quartz ±magnetite ±anhydrite ±sulphides (Serrano et al., 1996).
At Rio Blanco, chalcopyrite predominates, with subordinate bornite, pyrite and molybdenite. As in the actinolite-magnetite domain, this part of the potassic zone, is also characterised by abundant iron oxides. Laterally, to the east and west of the Rio Blanco underground mine, the intensity of disseminated alteration and mineralisation decreases, chlorite and epidote become more common, chalcopyrite:pyrite ratios decrease, and sulphide mineralisation is increasingly composed of stockwork veining rather than disseminations. The lavas above the intrusive contact contained high-grade copper ore that supported the Andina block-cave mine. However, upward from this high grade zone, the intensity of biotite alteration and the chalcopyrite:pyrite ratio decrease, and at higher levels still these lavas have only been subjected to propylitic alteration, including chlorite, epidote, calcite, specularite, pyrite and siderite (Serrano et al., 1996).
A high proportion of the potassic alteration zone is composed of a range of steep mineralised breccias, known as the Rio Blanco Breccia complex. This complex comprises multiple mineralised biotite ±anhydrite ±tourmaline ±specularite breccias which were emplaced in, and contain clasts of, both granodiorite and andesite. The volumetrically most significant of these is a Magmatic Breccia, where clasts occur in a dark matrix dominated by fine-grained biotite along with tourmaline ±quartz ±anhydrite ±feldspar ±magnetite ±sulphides (chalcopyrite, bornite, molybdenite and minor pyrite). Frikken (2003) notes that Magmatic Breccia is a mine term, and is not technically a magmatic breccia, but a breccia with a rock flour matrix that has been partially altered to an assemblage of microgranular hydrothermal minerals. Other breccias in this complex have matrices dominated by either tourmaline, anhydrite and/or specularite, along with sulphides.
Heterogenous brecciation resulted in the degree of attrition and the proportion of rock flour matrix and clasts to be spatially variable across the Magmatic Breccia. The clasts are also vary in size (2 mn to >5 m wide), with smaller clasts generally being more rounded, although, throughout the breccia, angular to sub-angular clasts predominate. The clasts of andesite and granodiorite have been altered, with primary igneous minerals replaced by secondary biotite ±magnetite ±sulphides ±K feldspar. Overall, the intensity of alteration of the clasts is much weaker than that of rock flour contained in the breccia matrix (Frikken, 2003).
In general, the margins of the Magmatic and other breccias are generally defined by a gradual decrease in brecciation and alteration over tens of metres, although in some areas, sharp contacts with coherent granodiorite have been recognised.
The roots of these breccias are >800 m below the roof of the granodiorite and the overlying lavas (Serrano et al., 1996; Frikken, 2003; Deckart et al., 2005).
Late Miocene to early Pliocene ages of 7.4 to 4.2 Ma been determined for different phases of the Magmatic Breccia and a tourmaline breccia of the complex, and for biotite of the altered granodiorite from the potassic alteration zone.
Fluid inclusion data indicate both breccia matrix minerals and potassic alteration resulted from high temperature, highly saline, boiling fluids at depths of 1 to >3 km below the palaeosurface (Kusakabe et al., 1990; Skewes, 1994), consistent with their location below ~1 km of lavas. Anhydrite-chalcopyrite sulphur-isotope geothermometry suggest associated mineralisation occurred at >460°C dominated by magmatic rather than meteoric sources (Kusakabe et al., 1984).
Sr and Nd isotopic data from the Magmatic Breccia matrix minerals and anhydrite breccia indicate these breccias were generated by fluids that are isotopically distinct from the granodiorite host plutons (Skewes and Stern, 1994; 1996). It is therefore suggested the magmatic fluids that generated the Rio Blanco breccia complex, and the younger mineralised tourmaline breccia pipes at Los Bronces and Sur-Sur (see below), exsolved from magmas crystallising at depths below the roots of the breccias (Skewes and Stern, 1994; 1996).
• Tourmaline breccias and sericitic alteration - A series of large, but younger mineralised tourmaline breccia pipes that contain ~50% of the copper in the Rio Blanco-Los Bronces deposits, are found peripherally to the main zone of potassic alteration and high-grade disseminated and stockwork mineralisation at Rio Blanco as described above. Most of these tourmaline breccias have matrix cement dominated by tourmaline and sulphides (chalcopyrite, pyrite, molybdenite and minor bornite) and locally >10% Cu contained both within the matrix and the enclosed altered and mineralised clasts. The most significant are the Donoso breccia at Los Bronces (Warnaars et al., 1985), to the west and the Sur-Sur breccia to the south (Stambuk et al., 1985).
Warnaars et al. (1985) recognised at least seven different breccia types in the Los Bronces open pit area, west of the main potassic alteration zone at Rio Blanco. These breccia types are differentiated on the basis of matrix assemblages, clast size and types, and degree of mineralisation. They cover an area of ~2000 x 700 m, and occur within and around the Los Bronces open pit, which is centred on the youngest and most strongly mineralised, the ~900 x 450 m tourmaline-sulphide Donoso Breccia in the north of this complex. The Los Bronces breccia complex has sharp contacts with the surrounding intrusive rocks and andesites, and dips inwards on the north, west and southern margins. The eastern contact is almost vertical, suggesting a 15° westward tilt of the breccia complex after emplacement. The internal breccia contacts are locally sharp, but elsewhere individual breccias coalesce, interfinger or have gradational contacts with each other (Frikken, 2003).
The Donoso Breccia contains the highest hypogene Cu grades of those at Los Bronces, other than local high-grade zones in the Infiernillo Breccia. It is the youngest (~5 Ma; Warnaars et al., 1985) and the northernmost breccia body, and shares textural and mineralogical similarities with the Sur-Sur Tourmaline Breccia (Serrano et al., 1996). The ore mineralisation is generally restricted to the breccia matrix, occurring as coarse aggregates or irregular patches of pyrite, chalcopyrite and rare bornite. The clasts on the southwestern side of the breccia contain minor stockwork and disseminated mineralisation. The mineral paragenesis in the breccia matrix is quartz → black tourmaline (dravite) → pyrite → chalcopyrite → specularite (Warnaars et al., 1985).
Another four distinct breccia types are found to the SE of Rio Blanco, where the Sur-Sur open pit, the southern extension of the Rio Blanco mine, has been developed in the Sur-Sur Breccia Complex (Stambuk et al., 1985). This breccia complex hosts ~25% of the total resource at Rio Blanco and takes the form of a dyke-like breccia zone that is at least 3 km long, 200 m wide and has a vertical extent of at least 1 km (Frikken et al., 2005).
The Sur-Sur Tourmaline breccia is a sulphide-iron oxide breccia that is host to the main Sur-Sur orebody, and locally intrudes the potassic phase Magmatic breccia to the north, and Specularite breccias at Rio Blanco and at Sur-Sur. It is monomictic and hosted in the Cascada Granodiorite phase of the San Francisco batholith. It contains variable sized clasts of granodiorite, supported by a milled rock flour matrix that has been cemented by early biotite, biotite alteration and anhydrite at depth, grading upwards via a transition zone between 3000 and 3100 m elevation, where biotite and tourmaline coexist as breccia cement, to dominant tourmaline cement with specularite and quartz-sericite-tourmaline alteration, with no anhydrite above 3600 m. This zonation is reflected by three tourmaline content subdivisions, containing <5%, 5 to 15% and >15% tourmaline.
These early-formed cements were overgrown and in some cases replaced during the main-stage mineralisation event, involving the deposition of quartz, chalcopyrite, magnetite, pyrite, tourmaline and minor hematite in open spaces. Chalcopyrite and magnetite appear to have been co-precipitated. A specularite dominant zone above 3600 m coincides with an increase in vugs, which are attributable to either the leaching of anhydrite and carbonates (Vargas et al., 1999) or residual voids that were never filled by mineral precipitation (Frikken, 2003). Locally, between 3600 and 4000 m elevation, magnetite replaces and pseudomorphs the specularite cement. This interval corresponds to higher grades of up to 2% Cu. Magnetite is not found above 4000 m elevation, where pyrite is the dominant sulphide mineral and specularite coexists with chalcopyrite (Frikken, 2003). Measured homogenisation temperatures are mostly between 450 and 300°C, and salinities range from 0 to 69 wt.% NaCl equiv..
Where the matrix content is low, clasts are generally angular to sub-angular, but with increasing matrix content they are more rounded, attributed to a greater degree of milling. Clast sizes range from <1 mm, up to several metres (Frikken et al., 2005). Unlike the other breccias of the Sur-Sur complex as described immediately below, it does not appear to incorporate clasts of the late Don Luis Porphyry.
The Castellana Breccia strikes north-south and truncates the Tourmaline breccia along its western boundary with an abrupt contact. It is heterolithic and contains rounded clasts of the Tourmaline and Monolito breccias, as well as andesite, granodiorite and the feldspar and Don Luis porphyries (see below) in a 50 to 90% grey coloured rock flour matrix and <10% tourmaline (Vargas et al., 1999). Even though it has undergone quartz-sericite alteration similar to the Tourmaline breccia, ore grades are generally <0.5% Cu (Stambuk et al., 1988). At the contact with the Tourmaline breccia, the abundances of tourmaline, sulphide and specular hematite increase, possibly due to assimilation (Frikken, 2003).
The Monolito Breccia, which occurs to the west of the Castellana Breccia across a coalescent, indistinct contact. It contains a rock flour matrix similar to the Castellana Breccia, although the clasts and matrix have been strongly altered to a chlorite assemblage with an intensity ranging from incipient to almost complete textural obliteration. It is heterolithic, containing fragments of Tourmaline breccia, as well as andesite, granodiorite and the feldspar and Don Luis porphyries (see below), and has uniformly low <0.5% Cu (Vargas et al., 1999). One K-Ar whole rock age of 5.2 Ma has been determined for (Serrano et al., 1996). However, as the breccia encloses clasts interpreted to be of the 4.9 Ma and 3.9 Ma Don Luis porphyry, this may be incorrect (Frikken, 2003).
The Paloma Breccia occurs as a north-south oriented body to the south of the Sur-Sur open pit, and as numerous dykes cutting the other breccia units. It is characteristically composed of small, well-rounded clasts in a strongly tourmaline altered matrix of rock flour with chalcopyrite, pyrite, quartz, magnetite and minor chlorite and siderite that comprises 50 to 80% of the breccia (Stambuk et al., 1988; Vargas et al., 1999). Clasts are moderately to intensely altered culminating in textural obliteration, as a result of multiple re-working through successive breccia events. It contains clasts of andesite, granodiorite, Don Luis porphyry and Tourmaline, Castellana and Monolito breccias. A K-Ar, whole-rock age determined for this breccia also resulted in an age of 5.2 Ma (Serrano et al., 1996). However, as for the Monolito Breccia, this is considered unlikely (Frikken, 2003).
Other breccias, with variable proportions of tourmaline, quartz, chlorite, sericite, anhydrite, specularite, sulphides and rock-flour in the matrix, are less strongly mineralised. Many of these, like the older potassic breccias and alteration, are also characterised by abundant iron oxides. All appear to have steep margins.
The clasts of these mineralised tourmaline breccias are generally monomictic, dominated by clasts of the enclosing plutonic host rocks, although some contain fragments of both plutonic and andesitic volcanic rocks, while others may include clasts of older breccias. Where breccias zones cross the contact between intrusive and extrusive rocks, there is generally a corresponding sharp transition in clast type. Clasts generally comprise as much as 70 to 90 vol.% of the breccia, and tend to be angular, with little evidence of significant vertical movement or abrasion. The size of clasts varies from centimetres to tens of metres.
These composite breccia pipe complexes, which outcrop over a belt that is >5 km long, and strike between 330 and 350°, generally have sharply defined and steeply dipping contacts with the surrounding intrusive and extrusive rocks. They have known vertical extents of >1000 m, and have been dated at 5.9 to 5.1 Ma in the late Miocene. Clasts containing earlier stockwork quartz veins and potassic alteration assemblages have been recognised in some of these breccias, consistent with their emplacement after the development of the potassic alteration zone.
Fluid inclusions in matrix minerals of the Donoso Breccia, at an elevation where the breccia is hosted by a granodiorite pluton of the San Francisco Batholith, indicate formation within only a few hundred metres of the palaeosurface (Skewes and Holmgren, 1993). This is taken to support emplacement of the mineralised tourmaline breccias as a second, post potassic phase of mineralisation, following significant uplift and erosional unroofing of the pluton.
Fluid inclusions in breccia-matrix minerals indicate the fluids that formed the mineralised tourmaline breccias were high-temperature, highly saline, boiling fluids (Holmgren et al., 1988; Skewes and Holmgren, 1993). Oxygen (total fluid δ180=+8.5‰), hydrogen (total fluid δD=-90‰) and sulphur (total fluid δ34S=+4‰) isotopes preclude the participation of an appreciable volume of meteoric water and instead imply these tourmaline breccias, like the older biotite breccias in the potassic domain were generated by fluids that were magmatic in origin (Holmgren et al., 1988).
The enclosing plutonic rocks across the generally sharp contacts exhibit little evidence of alteration related to the emplacement of these breccias. In contrast, plutonic clasts within the breccias are strongly sericite and silica altered, and may be mineralised as well. Where the clasts are sufficiently large, concentric zonation in the intensity of the sericitic alteration and mineralisation show that it occurred during breccia emplacement and was produced by the same magmatic fluids that generated the breccias. Andesite and, in some places, plutonic clasts are chloritised. However, the chlorite alteration of clasts may have preceded the sericite associated with breccia emplacement, as hydrothermal chlorite is seen to be pseudomorphically replaced by specularite ±tourmaline within strongly sericitised clasts (Warnaars et al., 1985).
• Late porphyries and diatremes - A suite of late Miocene to early Pliocene porphyritic rocks cut both the potassic domain of disseminated and stockwork mineralisation and some of the younger mineralised tourmaline breccia pipes. Some of these porphyries are, in turn, cut by later tourmaline breccias. These young porphyries include subvolcanic plugs, domes, diatremes, and locally extrusions, that sit directly on granodiorite with potassic alteration, indicating that, like the younger tourmaline breccia pipes described above, they were emplaced near the palaeosurface after a significant amount of erosion had unroofed the early stage mineralisation.
Several different porphyry bodies have been differentiated, including i). quartz monzonite porphyry dykes cutting the high-grade potassic domain disseminated and stockwork mineralisation at Rio Blanco (Blondel, 1980), and ii). a 6 km long north-south belt of dacitic to rhyolitic porphyry stocks, dykes, subvolcanic plugs, diatremes, domes and extrusions, collectively known as the Rio Blanco subvolcanic complex (Vergara and Latorre, 1984; Blondel et al., 1988).
The quartz monzonite porphyry dykes generally trend at 153°, and intrude the biotite altered and mineralised granodiorite and many of the mineralised breccias, particularly the Magmatic Breccia, in the high-grade ore zone at the Rio Blanco underground mine (Blondel, 1980). Porphyry dykes with similar textures and orientation are found up to 500 m east and 1000 m west of those in the high-grade mineralisation zone. All of these dykes increase in thickness downward and emanated from a quartz monzonite stock at depth (Blondel, 1980). Although quartz monzonite porphyry dykes are found within the zone of intense biotite alteration and high-grade mineralisation at Rio Blanco, they are not biotite altered themselves. They are either fresh, or are replaced by chlorite or sericite and silica, and both magmatic and hydrothermal biotite and feldspar of the surrounding altered and mineralised granodiorite are replaced by fine grained biotite and sericite at the contacts with porphyry dykes (Serrano et al., 1996).
The dykes, which are only weakly to moderately sericitised and silicified, are cut by quartz-sericite, and less commonly, K feldspar veins, but not biotite veins, suggesting the quartz monzonite porphyry dykes postdate the intense potassic biotite alteration (Skewes, 1994). The porphyry dykes are generally only relatively weakly mineralised, averaging <0.5% Cu (Blondel, 1980), compared to the surrounding granodiorite, with ~50% of this weak mineralisation being associated with quartz-sericite veining. The quartz monzonite porphyry dykes within the Rio Blanco area have been dated as 5.2±0.3 Ma (K-Ar on K feldspar; Blondel, 1980). This age is within the 5.2 to 3.9 Ma range determined for other late dacitic to rhyolitic porphyries of the Rio Blanco subvolcanic complex (Serrano et al., 1996).
The dacitic to rhyolitic porphyries of the Rio Blanco subvolcanic complex include, from south to north, the contiguous ~1800 x 650 m Don Luis dacite porphyry and associated dykes, which are oriented at 153°, parallel to the quartz monzonite porphyry dykes (Rojas, 1985; Blondel et al., 1988), the downward-tapering ~600 x 300 m Rio Blanco dacite plug, and the 2 km diameter La Copa rhyodacite diatreme and associated extrusions (Vergara and Latorre, 1984). A similar dacite plug and breccia complex is located ~1500 m SSE of the Don Luis dacite porphyry (Frikken, 2003).
The Don Luis Porphyry is a large, elongate porphyry body that crops out in the centre of the ore deposit complex, primarily enclosed by narrow zones of contact breccia considered to have formed during emplacement of the porphyry body (Serrano et al., 1996). However, it is also largely surrounded by a much larger area of mineralised tourmaline and related breccias, particularly those of the Sur-Sur breccia complex. The Don Luis Porphyry contains phenocrysts of plagioclase, quartz, K feldspar (up to 2 cm long) and minor (<10%) fine-grained biotite phenocrysts within a fine grained aplitic groundmass. Based on K/Ar age determinations, it was emplaced between 4.9 Ma and 3.9 Ma (Frikken, 2003).
The Rio Blanco dacite plug has the shape of an inverted cone, and is surrounded by zones of contact breccia. It contains phenocrysts of quartz and plagioclase with minor biotite in a fine grained quartz-feldspar matrix and auto-brecciated fragments, generally at contact zones. The matrix of the emplacement breccia generally contains secondary biotite, specularite, chlorite and minor chalcopyrite. Although grades are low (<0.3% Cu) in the emplacement
breccia, it appears to have been a focus for late-stage fluid flow and minor copper deposition (Frikken, 2003).
The La Copa Rhyolite is exposed as a large, circular body containing multiple felsic intrusive phases and an extrusive phase, and was the final intrusive complex to be emplaced into the ore deposit, resulting in the destruction of mineralised zones in the Rio Blanco sector (Serrano et al., 1996). Although it is dominantly composed of various intrusive phases, there is evidence of coeval eruptive facies at high elevations on the Los Bronces side of the
ore deposit (Wamaars et al., 1985). K/Ar age determinations of biotite and plagioclase from the intrusive phases in the La Copa Rhyolite range between 4.9 and 3.9 Ma (Frikken, 2003).
These intrusions post-date and disrupt the zone of potassic biotite alteration and associated high grade mineralisation, as well as the major mineralised tourmaline breccias peripheral to the potassic domain. The contacts between these late porphyries with the older plutons and mineralised breccias are commonly narrow zones of brecciation, some of which are cemented by tourmaline ±sulphides and rock flour, and are contact breccias related to the porphyry emplacement, as distinct from the large mineralised breccia pipes described above.
These late porphyries have undergone variable sericite and silica alteration, and are cut by quartz-sericite ±anhydrite ±sulphide veins, as well as narrow K feldpar veins, but are not biotite altered and are only weakly mineralised. Sericite alteration also occurs in the host rocks adjacent to the contacts with the young porphyries, overprinting host granodiorite that had been subjected to the older potassic alteration assemblages and pre-existing mineralisation is remobilised, and in some cases enhanced (Serrano et al., 1996).
Frikken et al. (2005) describe late veining within the Sur-Sur breccia complex, most likely associated with, but external to, the Don Luis Porphyry. The mineralised Sur-Sur tourmaline breccia is cut by two stages of post-breccia quartz sulphide veining. The first has quartz-sericite-pyrite alteration halos, and contains minor chalcopyrite, pyrite, bornite, anhydrite, tourmaline and fine blades of specularite. At lower levels, chalcopyrite cement in the breccia has been partly altered to bornite adjacent to these veins, and hematite has replaced magnetite cement, whilst fine blades of specularite surround and occur within the postbreccia veins. A second set of late-stage pyrite-rich veins with minor quartz, chalcopyrite, anhydrite and gypsum, and quartz sericite selvages, cut all other veins and breccias, up to and including the Don Luis porphyry.
The Cu-Mo sulphides internal to the late porphyries are interpreted as remobilisation of mineralisation in the intruded rocks (Rojas, 1985) and comprises pyrite and chalcopyrite plus minor bornite, molybdenite, galena, sphalerite, tennantite-tetrahedrite, luzonite, enargite, barite, calcite and alunite, generally occurring either in quartz-sericite veins or in a gangue of siderite, ankerite, gypsum and quartz. Minor arsenic is found in pyrite, arsenopyrite, luzonite and copper sulphoarsenides in the late porphyries (Serrano et al., 1996).
All of the late porphyries of the Rio Blanco subvolcanic complex have similar trace-element compositions, with lower total rare earth element contents and higher La/Yb ratios than the older host rocks of the deposit. This is taken to suggest the late porphyries involved more extensive crystal-liquid fractionation involving amphibole (Skewes and Stern, 1994; Stern and Skewes, 1995). The late porphyries also have higher 87Sr/86Sr and lower 143Nd/144Nd than the older host rocks, implying the incorporation of greater proportions of continental crust during their generation (Stern and Skewes, 1995).
• Supergene enrichment - Although supergene leaching and enrichment effects are not prominent at Rio Blanco-Los Bronces due to continuing erosion of the deposit and lower aridity, supergene enrichment has doubled the grade of copper along vertical fracture zones for up to 200 m below the surface. Leaching and enrichment also affected some highly permeable breccias where development of chalcocite and covellite, typically as thin films on hypogene sulphide grains, increased copper contents by two or more times to depths of up to 500 m below the surface (Warnaars et al., 1985). Supergene enrichment zones also occur along the margins of the late porphyries and diatremes in the Rio Blanco subvolcanic complex. The shape and depth of the enrichment zones suggest that they are related to the present groundwater regime and that leaching and enrichment are still active (Serrano et al., 1996).
Reserves and Resources
The combined Los Bronces - Rio Blanco porphyry system is estimated to contain more than 5 Gt @ 1% Cu, 0.02% Mo.
Los Bronces - At the Disputada Los Bronces mine, the reserves and resources in 2001 included:
Ore reserve - 475 Mt @ 1.03% Cu + 741 Mt @ 0.47% Cu leach ore, plus
Mineral resource - 200 Mt @ 0.95% Cu.
JORC compliant ore reserves and mineral resources at Los Bronces, as of 31 December 2011 (Anglo American plc, 2012) were:
Proved + probable reserves, flotation - 1.4984 Gt @ 0.62% Cu, 0.014% Mo, plus,
Proved + probable reserves, dump leach - 0.6837 Gt @ 0.33% Cu, plus,
Measured + indicated resources, flotation - 1.1339 Gt @ 0.43% Cu, 0.008% Mo,
Inferred resources, flotation - 3.1993 Gt @ 0.39% Cu,
Measured + indicated resources, dump leach - Nil,
Inferred resources, dump leach - 114.4 Mt @ 0.26% Cu.
JORC compliant ore reserves and mineral resources at Los Bronces, as of 31 December 2015 (Anglo American plc, 2015) were:
Proved + probable reserves, flotation - 1.2101 Gt @ 0.58% Cu, 0.014% Mo, plus,
Proved + probable reserves, dump leach - 0.3875 Gt @ 0.33% Cu, plus,
Measured + indicated resources, flotation - 2.5275 Gt @ 0.42% Cu, 0.009% Mo,
Inferred resources, flotation - 1.6393 Gt @ 0.39% Cu, 0.010% Mo,
Measured + indicated resources, dump leach - Nil,
Inferred resources, dump leach - 46.1 Mt @ 0.28% Cu.
Andina - Published reserves and resources at the Codelco Andina operation include:
Proved + probable ore reserves and "identified mineral resources" in 2011 - 5.888 Gt @ 0.78% Cu (Codelco presentation 2011).
Proved + probable ore reserves in 2015 - 986 Mt @ 0.75% Cu (Codelco reserves and resources report, 2015)
Measured + indicated + inferred in situ mineral resources in 2015 - 4.618 Gt @ 0.82% Cu, which included
inferred resources of 1.949 Gt @ 0.80% Cu (Codelco reserves and resources report, 2015)
Measured + indicated + inferred in situ geological resources in 2015 - 21.745 Gt @ 0.61% Cu.
Note: at both operations, resources are inclusive of reserves.
For more detail consult the reference(s) listed below which were the principal source of the information on which this summary was based.
The most recent source geological information used to prepare this decription was dated: 2015.
Record last updated: 10/7/2016
This description is a summary from published sources, the chief of which are listed below. © Copyright Porter GeoConsultancy Pty Ltd. Unauthorised copying, reproduction, storage or dissemination prohibited.
Los Bronces Andina
|
|
Covacevic, V.S., Serrano, L. and Vargas, R., 1985 - Geologia del sector Sur-Sur Yacimiento Rio Blanco: in IV Congreso Geologico Chileno, Agosto 1985, Universidad del Norte, Antofagasta Part 3 pp. 383-404. [in Spanish]
|
Crespo, J., Reich, M., Barra, F., Verdugo, J.J., Martinez, C., Leisen, M., Romero, R., Morata, D. and Marquardt, C., 2020 - Occurrence and Distribution of Silver in the World-Class Rio Blanco Porphyry Cu-Mo Deposit, Central Chile: in Econ. Geol. v.115, pp. 1619-1644.
|
Davidson P, Kamenetsky V S 2001 - Immiscibility and continuous felsic melt-fluid evolution within the Rio Blanco Porphyry System, Chile: evidence from inclusions in magmatic quartz: in Econ. Geol. v96 pp 1921-1929
|
Davidson, P., Kamenetsky, V., Cooke, D.R., Frikken, P., Hollings, P., Ryan, C., Van Achterbergh, E., Mernagh, T., Skarmeta, J., Serrano, L. and Vargas, R., 2005 - Magmatic Precursors of Hydrothermal Fluids at the Rio Blanco Cu-Mo Deposit, Chile: Links to Silicate Magmas and Metal Transport: in Econ. Geol. v.100, pp. 963-978.
|
Deckart, K., Silva, W., Sprohnle, C. and Vela, I., 2014 - Timing and duration of hydrothermal activity at the Los Bronces porphyry cluster: an update: in Mineralium Deposita v.49, pp. 535-546.
|
Deckart. K., Clark, A.H., Aquilar-A, C., Vargas-R, C., Bertens-N, A., Mortensen, J.K. and Fanning, M., 2005 - Magmatic and Hydrothermal Chronology of the Giant Rio Blanco Porphyry Copper Deposit, Central Chile: Implications of an Integrated U-Pb and 40Ar/39Ar Database: in Econ. Geol. v.100, pp. 905-934.
|
Frikken, P.H., Cooke, D.R., Walshe, J.L., Archibald, D., Skarmeta, J., Serrano, L. and Vargas, R., 2005 - Mineralogical and Isotopic Zonation in the Sur-Sur Tourmaline Breccia, Rio Blanco-Los Bronces Cu-Mo Deposit, Chile: Implications for Ore Genesis: in Econ. Geol. v.100, pp. 935-961.
|
Hohf, M., Trumbull, R.B., Cuadra, P. and Sole, M., 2023 - Tourmaline Breccias from the Rio Blanco-Los Bronces Porphyry Copper District, Chile: Constraints on the Fluid Source and the Utility of Tourmaline Composition for Exploration: in Econ. Geol. v.118, pp. 779-800. doi:https://doi.org/10.5382/econgeo.4991.
|
Hollings, P., Cooke, D. and Clark, A., 2005 - Regional Geochemistry of Tertiary Igneous Rocks in Central Chile: Implications for the Geodynamic Environment of Giant Porphyry Copper and Epithermal Gold Mineralization: in Econ. Geol. v.100, pp. 887-904.
|
McInnes B I A, Evans N J, Fu F Q, Garwin S, Belousova E, Griffin W L, Bertens A, Sukarna D, Permanadewi S, Andrew R L and Deckart K, 2005 - Thermal History Analysis of Selected Chilean, Indonesian and Iranian Porphyry Cu-Mo-Au Deposits: in Porter T M (Ed), 2005 Super Porphyry Copper & Gold Deposits - A Global Perspective, PGC Publishing, Adelaide, v.1 pp. 27-42
|
Mpodozis, C. and Cornejo, P., 2012 - Cenozoic Tectonics and Porphyry Copper Systems of the Chilean Andes: in Hedenquist J W, Harris M and Camus F, 2012 Geology and Genesis of Major Copper Deposits and Districts of the World - A tribute to Richard H Sillitoe, Society of Economic Geologists, Denver, Special Publication 16, pp. 329-360
|
Piquer, J., Skarmeta, J. and Cooke, D.R., 2015 - Structural Evolution of the Rio Blanco-Los Bronces District, Andes of Central Chile: Controls on Stratigraphy, Magmatism, and Mineralization: in Econ. Geol. v.110, pp. 1995-2023
|
Serrano, L., Vargas, R., Stambuk, V., Aguilar, C., Galeb, M., Holmgren, C., Contreras, A., Godoy, S., Vela, I., Skewes, M.A. and Stern, C,R., 1996 - The late Miocene to early Pliocene Rio Blanco-Los Bronces copper deposit, Central Chilean Andes: in Camus, F., Sillitoe, R.H. and Petersen, R., (Eds.), Andean Copper Deposits: New Discoveries, Minerlisation, Styles and Metallogeny, Soc. Econ. Geologists, Spec. Publ. No. 5, pp. 119-130.
|
Skarmeta, J., Ortiz, F. and Sole, M., 2024 - Geology and Structure of the Rio Blanco Cu-Mo Porphyry Deposit, Central Chile: in Econ. Geol. v.119, pp. 251-278. doi: 10.5382/econgeo.5043.
|
Skewes, M.A., Holmgren, C. and Stern, C.R., 2003 - The Donoso Copper-rich, Tourmaline-bearing breccia pipe in central Chile: petrologic, fluid inclusion and stable isotope evidence for an origin from magmatic fluids: in Mineralium Deposita v.38 pp. 2-21.
|
Stern C R and Skewes M A, 2005 - Origin of Giant Miocene and Pliocene Cu-Mo Deposits in Central Chile: Role of Ridge Subduction, Decreased Subduction Angle, Subduction Erosion, Crustal Thickening, and Long-Lived, Batholith-Size, Open-System Magma Chambers: in Porter, T.M. (Ed), 2005 Super Porphyry Copper & Gold Deposits - A Global Perspective, PGC Publishing, Adelaide, v.1 pp. 65-82
|
Toro, J.C., Ortuzar, J., Zamorano, J., Cuadra, P., Hermasilla, J. and Sprohnle, C., 2012 - Protracted Magmatic-Hydrothermal History of the Rio Blanco-Los Bronces District,Central Chile: Development of Worlds Greatest Known Concentration of Copper: in Hedenquist J W, Harris M and Camus F, 2012 Geology and Genesis of Major Copper Deposits and Districts of the World - A tribute to Richard H Sillitoe, Society of Economic Geologists, Denver, Special Publication 16, pp. 105-126.
|
Warnaars F W, Holmgren-D C, Barassi-F S 1985 - Porphyry Copper and Tourmaline Breccias at Los Bronces-Rio Blanco, Chile: in Econ. Geol. v80 pp 1544-1565
|
Porter GeoConsultancy Pty Ltd (PorterGeo) provides access to this database at no charge. It is largely based on scientific papers and reports in the public domain, and was current when the sources consulted were published. While PorterGeo endeavour to ensure the information was accurate at the time of compilation and subsequent updating, PorterGeo, its employees and servants: i). do not warrant, or make any representation regarding the use, or results of the use of the information contained herein as to its correctness, accuracy, currency, or otherwise; and ii). expressly disclaim all liability or responsibility to any person using the information or conclusions contained herein.
|
Top | Search Again | PGC Home | Terms & Conditions
|
|