Escondida - Escondida Norte, Escondida Este, Zaldivar, Pinta Verde, Pampa Escondida, Chimborazo, Baker, Pamela, Rincones |
|
Chile |
Main commodities:
Cu Mo
|
|
 |
|
 |
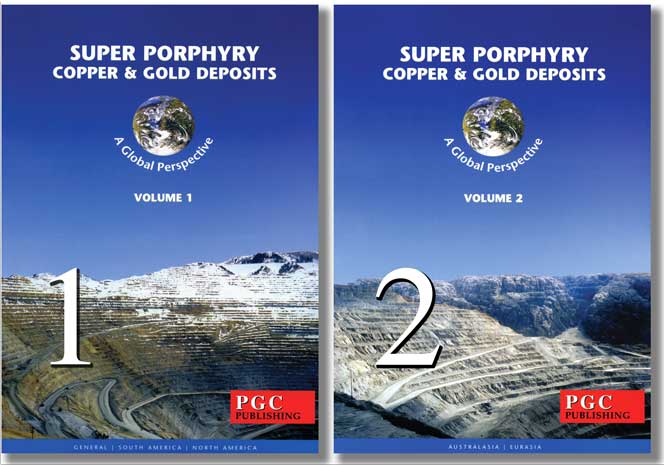 |
Super Porphyry Cu and Au

|
IOCG Deposits - 70 papers
|
All papers now Open Access.
Available as Full Text for direct download or on request. |
|
 |
Escondida is a giant supergene enriched and hypogene open pit porphyry copper deposit located in northern Chile, some 160 km to the ESE of Antofagasta (#Location: 24° 16' 17"S, 69° 4' 13"W).
Escondida is the largest of a cluster of related porphyry deposits in the surrounding district. The others include Escondida Este, Escondida Norte, Zaldívar, Pinta Verde and Pampa Escondida (Fig. 2). The latter was found to underlie the earlier Carmen and Ricardo prospects as known in 2001. Ricardo was investigated because of supergene chalcocite, rimming pyrite within rhyolites, emplaced along the Zaldívar Fault, with, at that stage, no hypogene copper minerals having been encountered. The Carmen mineralisation was largely masked by gravel, with insufficient drilling information to define its extent (Padilla-Garza et al., 2001). Three other, principally supergene blanket deposits/prospects, Chimborazo, Baker and Rincones are external to the main central cluster, while the Pamela Oxide resource lies between Escondida Norte and Pampa Escondida, partially overlain by Escondida Norte dumps.
Reserves and resources for all of these deposits/prospects are listed at the end of this record.
This cluster of deposits falls within a north-south elongated area of 12 x 6 km, localised within a transtensional sigmoidal structure, the 'Escondida-Sierra de Varas shear lens' coinciding with the intersection of north-south trending, sinistral, strike slip faults of the Domeyko Fault System, and a regional NW trending structural corridor, the Archibarca Lineament. The Domeyko Fault System is also known as the Falla Oeste, or West Fissure Fault Zone that localises other deposits such as Chuquicamata and Collahuasi to the north (Fig. 1; Richards et al., 2001; Padilla-Garza et al., 2001).
These deposits are all centred on composite multistage biotite granodiorite porphyry stocks and are closely associated with volumetrically minor, but commonly high-grade, magmatic-hydrothermal breccias. The earliest granodiorite porphyry phase at each deposit invariably hosts the highest grade mineralisation.
There is a well developed alteration-mineralisation zonation developed across the cluster of deposits, from a deep potassic overprinted by grey sericite assemblages containing chalcopyrite and bornite; to more pyritic chlorite-sericite and sericitic zones at intermediate levels; and shallow advanced argillic assemblages regarded as remnants of extensive lithocaps. The latter are characterised by high-sulphidation, copper-bearing sulphides, much of it as enargite-rich, massive sulphide veins.
Significant mineralisation within the district is associated with three main events, namely: i). the earliest, ~41 Ma intrusions at Chimborazo; followed, between ii). ~38 and 36 Ma by the main mineralisation at the Escondida and Escondida Norte-Zaldívar deposits in particular; and iii). from ~36 to 34 Ma at the Escondida Este and Pampa Escondida deposits. Hervé et al. (2102) conclude the Escondida, Escondida Norte-Zaldívar and Baker deposits, were substantially telescoped subsequent to deposition, whilst the earlier Chimborazo and later Escondida Este and Pampa Escondida deposits, were only subjected to minor telescoping. For instance, at Chimborazo, the chlorite-sericite alteration at the base of the main lithocap lies ~600 m above the underlying potassic zone. In contrast, at the Escondida, Escondida Norte-Zaldívar, and Baker deposits, advanced argillic alteration and accompanying high-sulphidation copper mineralisation overprints quartz-veinlet stock-worked porphyry intrusions. However, this conclusion is mainly based this on the vertical separation between the main porphyry mineralisation and the lithocap at each. However, the lithocap at Escondida appears, in part at least to be related to the underlying Escondida Este, which is a separate later porphyry complex. A similar relationship may exist between Escondida Norte and the deeper, but laterally separated Pampa Escondida related event. Hervé et al. (2102) show the high sulphidation alteration and mineralisation cutting Escondida to also overprint the northern mineralised centre of Escondida Este, suggesting the latter has also been significantly telescoped (Fig. 7). Never-the-less, assuming they were both emplaced at similar depths below the surface, Escondida and Escondida Norte-Zaldívar had been significantly uplifted prior to the emplacement of Escondida Este and Pampa Escondida. However, that uplift resulted in exposure of the former pair, such that deep, mature supergene profiles are were developed at both, whilst the latter pair have not been uplifted to sufficiently close to the surface to produce other than minor supergene mineralisation. This has been suggested to imply Escondida and Escondida Norte-Zaldívar were emplaced during the maximal rates of Incaic uplift and unroofing, whilst the younger Escondida Este and Pampa Escondida deposits coincided with declining uplift from ~36 to 34 Ma, and Chimborazo preceded the major uplift event (Hervé et al., 2102)
The hydrothermal activity responsible for the hypogene mineralisation at the largest of the deposits, Escondida, is closely associated with a late Eocene to Oligocene dioritic to quartz-monzonite to granodiorite stock complex composed of at least three intrusive stages. This complex intrudes a sequence of Palaeozoic andesite, cut by late Palaeozoic (265 to 270 Ma) rhyolites, marine-continental Mesozoic sedimentary rocks (calcareous sandstone, red sandstone and conglomerate) unconformably overlain by late Cretaceous to Paleocene andesites. The mineralised intrusive complex is overlain and cut by Oligocene to Miocene rhyolitic sub-volcanic intrusives and extrusives.
At Escondida, the mineralised NW-SE trending, 4.5 x 2.5 km quartz-monzonitic to granodioritic porphyry stock complex cuts Paleocene andesites. The complex is composed of at least three phases, the first two of which are distinguished on their phenocryst size, composition and quantity while the third is a porphyry breccia which contains mineralised clasts but is cut by copper rich veins.
Alteration commenced with an early potassic (biotite rich, particularly within the andesites) stage grading out into a propylitic periphery and a silicified shell around the intrusive, with associated magnetite, chalcopyrite and bornite and a hypogene grade of generally <0.2% Cu. The second phase corresponded to sericite-chlorite and then to quartz-sericite alteration with chalcopyrite, pyrite and molybdenite carrying 0.4 to 0.6% Cu and chalcopyrite:pyrite ratios of 3:1. The third and final phase comprised an acid sulphate episode which over-printed the previous assemblages, with a younger advanced argillic alteration and a range of sulphides including bornite, chalcopyrite, pyrite, chalcocite, covellite, enargite, sphalerite tennantite and galena, producing grades of 0.6 to 1.0% Cu where overprinting the earlier pulses of mineralisation, and likely related to the younger Escondida Este event.
Supergene enriched ore represented 65% of the total resource at Escondida in 2005. The upper surface of the supergene blanket is sub-horizontal and is displaced by faulting, while the base is irregular, with thicknesses varying from a few metres to 400 m. The leached cap extends from the present surface to a depth of around 200 m above the chalcocite blanket, to only a few metres over the local copper oxide zone. It carries hematite over the deeper higher grade chalcocite blanket and jarosite over shallower, lower grade sections of the blanket. Over the copper oxides, goethite dominates. The copper oxide zone represents around 5% of the resource and is composed of oxides, silicates, sulphates and carbonates of copper, mainly brochantite, antlerite, atacamite, chrysocolla, wad and tenorite.
Sulphides within the zone of supergene enrichment occur as disseminations and in veins and include chalcocite, covellite with minor digenite and idaite (Cu5FeS6) replacing pyrite, chalcopyrite and bornite. Chalcocite occurs throughout the blanket, while covellite and digenite appear in the middle, and increase downwards. Copper grades vary from 0.3 to >2%, but are highest in the thickest part of the blanket over the best developed hypogene mineralisation, in the zone where strong quartz-sericite and subsequent advanced argillic alteration overprints early biotite.
Regional Setting
The Escondida district, in the Cordillera Domeyko, is located within the Andean Mountains of northern Chile, near the present day western margin of South America. This margin has been an active, destructive plate boundary since the Mesozoic when it formed the western seaboard of Gondwana. The geology is dominated by Mesozoic and Cenozoic sedimentation, magmatism and tectonic deformation of the Andean cycle, superimposed on and over a Proterozoic to Palaeozoic basement (Camus, 2005).
For background on the continental and regional scale tectonic, geologic and metallogenic setting of Central Chile, see the Central Andes and Bolivian Orocline record.
The Escondida deposit falls within the 50 km wide Cordillera de Domeyko morpho-tectonic province of the northern Chilean Andes, characterised by north-south elongated ranges and intervening shallow gravel filled basins at elevations varying from 2000 to 4500 m asl. It is one of a string of deposits associated with the >1000 km long, narrow Middle Eocene to Lower Oligocene (43 to 33 Ma) porphyry copper belt of northern Chile, largely between latitudes 21 and 31°S (Sillitoe, 1988; Camus, 2003; Sillitoe and Perelló, 2005).
The basement sequence below the Cordillera de Domeyko represents a complex continental margin of accreted continental fragments and Palaeozoic to Mesozoic volcanic arcs, but overall coincides with the transition from continental Precambrian and Palaeozoic crust to the east and thick Palaeozoic turbidites and volcanic-sedimentary sequences most likely resting on oceanic crust, to the west. During the Mesozoic a magmatic arc developed to the west, while the Cordillera de Domeyko was within an extensional back arc basin. This sequence was, in turn, followed by three continental margin Andean-type magmatic arcs from the late Cretaceous to late Cainozoic, with associated large porphyry deposits such as Escondida, formed mainly between 42 and 31 Ma, during the Eocene-Oligocene phase. From 31 Ma onwards, uplift, climatic change and low rates of denudation have favoured the development and preservation of supergene enriched blankets over these porphyry copper deposits.
The Cordillera de Domeyko province is dominated by the Domeyko fault system, which in the Escondida district is characterised by cymoid shapes producing elongated, north-south basins in a 200 x 20 to 50 km zone, probably representing long lived zones of structural activity, varying in character over time. Both Chuquicamata (200 km to the north) and Escondida lie on strike-slip faults of the Domeyko fault system.
Dextral displacement is recorded in the Domeyko Fault Zone until the late Eocene and, in places, probably into the early Oligocene. However, by the late Oligocene, the movement on this structural zone was markedly sinistral, persisting into the Miocene. This change, which may have taken affect at different times along the length of the Domeyko Fault Zone, coincides in general with the cessation of volcanism and the subsequent emplacement of the giant Eocene to Oligocene porphyry copper deposits of northern Chile, including Escondida. It is also most likely to have taken place during an interval of stress relaxation or reversal, promoting the introduction and emplacement of voluminous shallow level magmas (Richards et al., 2001, quoting other sources).
Richards et al., (2001), also consider it likely that transtension developed where oblique structures intersected the main strike-slip fault, causing deflections, points of weakness, and the development of pull part basins. They also utilised the structural interpretations of Salfity (1985) and Salfity and Gorustovich (1998) who identified a number of systems of NW and NE trending lineaments, and lineament corridors (plotted on Fig. 1) that cut across the entire Andean Orogen in northern Argentina and Chile and intersect the Domeyko Fault Zone. The intervals of intersection of these cross trending lineaments with the Domeyko Fault Zone exhibit a good correlation with the location of many of the known major late Eocene to Oligocene porphyry Cu-Mo deposits within the Domeyko Fault Zone (Fig. 1).
One such cluster of deposits is that of the Escondida district, at the intersection of the Domeyko Fault Zone and the NW trending Archibarca Lineament of Salfity (1985) and Salfity and Gorustovich (1998). Figs. 1 and 2 illustrate the intersection in a regional and on a district scale respectively. On Fig. 2, the Domeyko (West Fissure) Fault Zone is represented by the north-south set of faults that include the Ferrocarril, Portezuelo, Panadero and Zaldívar Faults, while the prominent set of NW oriented structures encompassing Zaldívar and Chimborazo belong to the Archibarca Lineament. These latter NW oriented faults have a sinistral displacement, as is indicated for the regional Archibarca Lineament by Salfity (1985).
Richards et al., (2001), speculate on the nature of the basement configuration reflected by the observable cross lineaments. These same cross lineaments also appear to control the location of major ore deposits in the other narrow, parallel north-south trending Cenozoic mineral belts in the central Andes, each of which reflects a separate pulse of the eastward progressing magmatic arc.
The main faults of the eastern Domeyko fault system are interpreted to represent inverted normal structures that previously defined the eastern margin of the backarc basin to the Mesozoic La Negra Arc of the Chilean Coastal belt to the west. These faults are interpreted to have been subjected to sinistral transpression prior to 41 Ma and emplacement of the Escondida porphyries, and produced the clockwise block rotation responsible for generation and initial synorogenic filling of the San Carlos depocentre (see below) between 41 and 38 Ma. The Escondida district was then subjected to a transient dextral transpression stress regime between ~38 and 34.5 Ma, which explains the systematic NNE- to NE orientation of the early, inter- and late mineral porphyry copper intrusions, and the associated alteration and mineralisation generated during that period. However, this dextral transpression was only incipient, as none of the porphyry copper deposits of the district has apparently undergone appreciable lateral or vertical offset since 41 Ma. This dextral regime is interpreted to have waned by the emplacement of the north-south trending, late mineral rhyolite porphyry at Escondida Este and was replaced by transient sinistral transpression during end-stage formation of NW-striking, high and intermediate sulphidation, massive sulphide veins and phreatic breccia dykes. Renewed uplift and denudation characterised the late Oligocene to early Miocene, during which the extensive lithocap was largely stripped and incorporated as detritus in a thick piedmont gravel sequence. The development of a hematitic leached capping and associated chalcocite enrichment blanket, accompanied by subsidiary oxide copper mineralisation, was active beneath the topographic prominences at Escondida, Escondida Norte-Zaldívar, and, to a lesser degree, Chimborazo from ~18 to 14 Ma. However, supergene activity was much less important at the topographically lower, gravel-covered more deeply emplaced Pampa Escondida and Escondida Este deposits. After ~14 Ma, supergene processes were terminated by the onset of hyperaridity throughout much of northern Chile (Hervé et al., 2102).
The Escondida District
Fig. 2 is an interpreted summary of the solid geology of the Escondida district, based on published mapping in Richards et al., (2001), and Padilla-Garza et al., (2001), modified from a publicly available presentration by Minera Escondida on the Pampa Escondida discovery (Hervé, 2010) and from Hervé et al. (2012) and Hao et al. (2019). The main rock units may be summarised as follows:
Permo-Carboniferous La Tabla Formation - These are the oldest rocks mapped in the immediate Escondida district and represent the local basement. They outcrop as fault bounded massifs to the east of the main faults of the West Fissure Zone (Domeyko Fault Zone), in the eastern half of the Escondida district. The most widespread exposed lithology of the La Tabla Formation in the district is a resistant quartz-feldspar porphyritic vitrophyre (i.e., a porphyritic igneous rock with a glassy groundmass) showing very little macroscopic structure, and occurring as units which rarely have non-faulted contacts with other units. It is composed of abundant phenocrysts (typically <5 mm across) of quartz and feldspar (saussuritised plagioclase) with lesser mafic minerals (chlorite altered biotite and hornblende) in a matrix of devitrified glass. This lithology is believed to represent an original glassy or pumiceous rock of volcanic origin, but also includes sub-volcanic intrusives. It ranges from a high-K rhyolitic affinity to dacitic and andesitic compositions. The chemistry is characteristic of arc magmas, with an I-type calc-alkaline magmatic affinity. Local units of Palaeozoic calcareous siltstones and mudstones are found overlying the volcanics with no skarn or hornfels development, suggesting the porphyries are mainly extrusive (Richards et al., 2001).
Urzua (2009) describes the formation as comprising a bimodal volcanic sequence of ignimbrltes, rhyolites and interlayered andesites and sedimentary rocks. The base of the formation is not exposed in the immediate district, although a gently angular unconformity separates it from the underlying Devonian to Late Carboniferous Zorritas Formation 60 km to the east (Niemeyer et al., 1985; Urzua, 1988). Weathered exposures of the upper part of the formation are unconformably overlain by Late Triassic conglomerates (Fuenzalida, 1986; Marinovic et al., 1995). It is up to 800 m thick (Marinovic et al., 1995), with massive beds having been deformed into open folds with kilometre-scale wavelengths. It has been tentatively divided into the following units, as described by Urzua (2009):
• Western Volcanic Sub-Unit - comprising a massive series of reddish-grey and coarse-grained, crystal-rich rhyolitlc ignimbrites, with rare autobrecciated lava flows. Several sub-volcanic rhyolites, possibly domes, and andesite plugs are part of the sequence (Marinovic et al., 1995; Richards et al., 2001). SHRIMP U-Pb zircon dates suggest an age between 294.2 ±2.4 and 290 ±4 Ma (Richards et al., 1999; Pollard and Taylor,
2002). In the Escondida Norte-Zaldívar area, it has been intruded by syenogranites and granodiorites dated at 298.9 ±2.6 and 293 ±6 Ma,
respectively (SHRIMP U-Pb zircon Pollard and Taylor, 2002).
• Central Volcano-Sedimentary Sub-Unit - which is poorly exposed and has a low relief. It forms the wall rock to part of the Escondida deposits and comprises a green to greenish-brown, crudely bedded suite of andesites, dacites and basaltic andesites, with layers of vitric and crystal tuffs, and interbedded volcanic sandstones. It has been dated at 287 to 282 Ma (U-Pb zircon; Urzua (2009).
• Eastern Volcanic Sub-Unit - which stratigraphically overlies the Central Volcano-Sedimentary Sub-Unit and is similar in composition to the Western Volcanic Sub-Unit. It is composed of massive, reddish-brown, flow-banded, welded and non-welded, coarse-grained crystal-rich tuffs of rhyolitic to dacitic composition. Associated flow-banded and massive, porphyritic dacite is encapsulated by a brecciated carapace. No reliable age dates are available, although they are intruded by 290 ±2 Ma granodiorite (U-Pb zircon) from the Imilac Plutonic Complex.
Late Triassic Agua Dulce Formation - Regionally, this is the first formation of the Andean cycle, and is part of the back arc basin to the La Negra Arc of the Chilean Coastal belt (straddling the Atacama Fault shown on Fig. 1). The back arc basin also contained widespread volcanic rocks, which, where interpreted to occur in the Escondida district, are largely characterised by a subdued topography, covered by a gravel pediment to the east of the Domeyko (West Fissure) Fault Zone. Due to the extensive cover and some lithological similarities with neighbouring units, both the eastern and western contacts of the formation are unclear and conjectural. It is composed of andesitic lavas, and rhyolitic tuffs and domes, and is cut by quartz monzonite to quartz-diorite and tonalite intrusives. The andesitic volcanic rocks are porphyritic with altered hornblende, clinopyroxene and saussuritised plagioclase phenocrysts set in a fine grained matrix which is sometimes trachytic in composition. The rhyolitic rocks contain phenocrysts of quartz, feldspar (plagioclase with lesser K feldspar) and locally, minor biotite in a glassy, usually devitrified, matrix. Some have textures suggesting a pyroclastic origin, while others appear to represent flow domes. The geochemistry of the andesites and rhyolites are quite different and are suggestive of separate sources, specifically, arc magmatic andesites and rhyolites with a crustal melting component (Richards et al., 2001). Padilla-Garza et al., (2001) did not differentiate the Triassic Agua Dolce Formation. They included all rocks mapped by Richards et al., (2001) in that formation as belonging to the La Tabla Formation, as shown on Fig 2. The rocks differentiated as being of the Agua Dolce Formation form a north tapering wedge shaped graben immediately to the east of the north-south corridor of La Tabla Formation hosting the Escondida cluster and the major NNW trending fault zone east of the Baker deposit. Hervé et al. (2102) also map these as La Tabla Formation, while Hao et al. (2019) show them as Agua Dulce Formation. The lithologies of the two formations are similar, although those mapped as the latter by Richards et al. (2001) form a more subdued topography compared to the bounding La Tabla Formation strips to the east and west.
Jurassic to Cretaceous Profeta and Santa Ana Formations - These units were developed in a subsiding back-arc basin, and represent an apparently continuous sequence, subdivided into the older, dominantly calcareous Profeta, and younger, mainly arenaceous Santa Ana Formations. The Profeta Formation unconformably overlies andesites of the Agua Dulce Formation to the north of Fig. 2, above a basal rubbly conglomerate and overlying silty carbonate. The sequence is up to 1 km thick in the north, and is composed of fossiliferous, micritic limestones, limestone breccias, and sandy and/or silty lenses. The Santa Ana Formation is characterised by orange- and yellow weathering sandstone and siltstones with subordinate carbonate bands. The contact between the two units outside of the mapped area is gradational. Within the area of Fig. 2, voluminous rhyolitic and minor andesitic rocks of the same age are mapped. Some are intercalated with the sediments, though most of the felsic rocks appear to represent flow domes from which the conformable volcanics extend (Richards et al., 2001). Padilla-Garza et al., (2001) consider the Profeta Formation to represent Triassic to Jurassic marine sediments and the Santa Ana Formation to be Cretaceous continental-subaqueous rocks.
Cretaceous Monzonite and Gabbro Intrusives - Approximately 5 km west of the Escondida pit, a north-south elongated composite body of quartz monzonite and lesser hornblende gabbro intrudes the volcano-sedimentary rocks of the Profeta and Santa Ana Formation. It is surrounded by a halo of hornfels and calc-silicate alteration, and separates the two intruded units in the map area. The gabbros cross-cut and postdate the quartz-monzonite. The quartz-monzonites are equigranular and medium grained, with rare quartz and plagioclase. The hornblende gabbros are dated at 76.9 to 74.0 Ma, giving a minimum age for both the Santa Ana Formation and the quartz-monzonites. Herve et al, 2012 quote an age range for these intrusives as 77 to 72 Ma. They comprise porphyritic to ophitic rocks with brown hornblende crystals to more than 1 cm across, intergrown with clinopyroxene and plagioclase laths with interstitial biotite and minor quartz, and have an alkalic geochemistry (Richards et al., 2001).
Late Paleocene to Early Oligocene Augusta Victoria Formation - The progressive eastward movement of the Cenozoic volcanic arc resulted in widespread Paleocene to Early Eocene andesitic and felsic volcanism of the Augusta Victoria Formation in the Cordillera Domeyko, accompanied by intercalated arenaceous and calcareous sedimentary rocks. The volcanic and sedimentary rocks of this unit, like the possible Agua Dulce Formation, form a subdued topography, masked by gravels, although the more felsic units occur as low, poorly exposed ridges. The unit is dominated by calc-alkaline andesitic flows which appear to largely comprise a gently folded, sub-horizontal, alternating sequence of locally vesicular, crystal rich andesitic lavas which have devitrification and eutaxitic textures, while more felsic units occur farther west. They overlie the Profeta and Santa Ana Formations with an angular unconformity (Richards et al., 2001). Marinovic et al., (1992) quote a biotite K-Ar age of 55 ±1.4 Ma from volcanics at the base of the unit. Urzúa (2009) determined 5 U-Pb zircon ages for andesite flows within the Augusta Victoria ranging from 57.0 ±0.6 to 53.9 ±0.8 Ma.
Late Eocene to Early Oligocene Dioritic Intrusions - Aeromagnetic data indicates clusters of magnetic diorite intrusions regionally distributed along the West Fissure (Domeyko Fault) Zone. One such cluster of stocks occupy an area of ~45 km2 surrounding the Escondida-Zaldívar-Chimborazo deposits and near Escondida Este and Baker, with the greatest density being to the NE of Chimborazo (Fig. 2). Intrusion commenced with fine-grained hornblende diorite and monzodiorite which occur as dykes and stocks. The diorite, which characteristically carries abundant hornblende phenocrysts and magnetite, intrudes both the Augusta Victoria and the Profeta Formations, and progresses to quartz diorite, monzodiorite and lesser quartz monzonite intrusions (Hervé et al., 2012). 40/39Ar dating of samples has returned ages of between 38.28 ±0.32 and 39.94 ±0.46 Ma, similar to the ages of syn-mineral porphyries at Escondida, Zaldívar and Chimborazo (see below). However, U-Pb zircon ages indicate most of these rocks were emplaced between ~43 and 41 Ma (Urzúa, 2009), although those at Baker and Pampa Escondida returned U-Pb zircon ages of 38.2 ±0.5 and 37.6 ±0.5 Ma, respectively. Similarly hornblende diorite and monzodiorite stocks in the southern section of Escondida Este are dated at ~36 and ~36 to ~38 Ma (U-Pb zircon; BHP Billiton, 2014). This suite of diorites have a very similar geochemical signature to the Augusta Victoria Formation andesites, with both lithologies being characterised by arc-like trace and major elements (Richards et al., 2001). These diorites and monzodiorites (including those at Baker, Escondida Este and Pampa Escondida) are regarded as precursors to the ore-related intrusions (Richards et al., 2001).
Eocene San Carlos strata - which comprise a sequence of siliciclastic sedimentary and andesitic volcanic rocks that occur immediately east of both Escondida Este and Escondida Norte, unconformably overlying the Augusta Victoria Formation. The sequence was deposited both before and during porphyry copper deposit formation at Escondida (Fig. 2). To the SE, it is exposed in the immediate footwall of the NW-vergent Hamburgo reverse fault (Fig. 2), and was informally designated as the San Carlos strata by Urzúa (2009). It has a maximum thicknesses of >1200 m and is composed of a crudely layered and moderately consolidated succession of grey-green and red sandstone and conglomerate (Hervé et al., 2012). In the upper sections of the sequence, these sedimentary rocks are interbedded with a cumulative thickness of as much as 500 m of andesitic to dacitic laharic breccia, coarse- to fine-grained lapilli-tuff, volcaniclastic sandstones, ignimbrite and subsidiary flows, which yielded two ages of 38.0 ±2.1 and 37.7 ±0.6 Ma (40/39Ar and U-Pb zircon respectively; Urzúa, 2009).
Late Eocene to Early Oligocene Porphyry Intrusions - A multiphase episode of late Eocene to early Oligocene porphyries accompanied mineralisation at the deposits of the Escondida district, as individually described later in this record. At the main Escondida deposit, mineralisation is associated with the composite Escondida Stock, which is composed of at least four phases of intrusion that have an overall granodioritic composition (combined on Fig. 3, but differentiated on Fig. 6). The NE-trending, early phases have traditionally been referred to as the Colorado Grande and Escondida porphyries (Ojeda, 1986, 1990; Padilla-Garza et al., 2001, 2004; Quiroz, 2003a), and are variously dated at 37.9 ±1.1, 37.7 ±0.8, and 37.2 ±0.8 Ma (Richards et al., 1999; Padilla-Garza et al., 2004). At a depth of >500 m below the main Escondida open pit, but exposed in the pit on its peripheries, the early granodiorite porphyries are cut by a much larger, 35.4 ±0.7 Ma stock of late intermineral biotite granodiorite porphyry (Fig. 6). The latter constitutes a low-grade floor to hypogene mineralisation beneath the high-grade supergene enrichment zone on the flanks of the deposit, a feature recognised by previous workers (e.g., Ojeda, 1986). The copper mineralised early granodiorite porphyry stock is cut towards the north by a 37.5 ±0.6 Ma, low grade inter-mineral biotite rhyolite dome, which is notably more silica-enriched with >10 vol.% quartz phenocrysts (Ojeda, 1986, 1990; Padilla-Garza et al., 2001). The latter is the Colorado Chico Rhyolite Dome of Padilla-Garza et al. (2001; Fig. 3 and 6).
These intrusions and the country rocks are cut by numerous small magmatic-hydrothermal breccia bodies which make up ~5% of the Escondida deposit, but invariably host the highest grade hypogene and supergene copper mineralisation. These breccias are commonly made up of polymict clasts which are set in varied proportions of quartz-sulphide cement and rock-flour matrix (Ojeda, 1986, 1990; Véliz, 2004). Late, poorly mineralised pebble dykes of phreatic origin, most of which strike NW, are also evident (cf. Sillitoe, 1985, Ojeda, 1986, 1990).
The Escondida deposit is bounded to the east by an upward flaring, late mineral biotite rhyolite porphyry with surface dimensions of 3 x 1.5 km, contracting to a 200 to 500 m thick dyke below surface, referred to by Padilla-Garza et al. (2004) as the Cerro Sureste Rhyolite (Fig. 3). This body occurs to the east of the trace of the north-south striking Panadero fault of the Domeyko Fault Zone (Figs. 6 and 7). At shallow depths, it exhibits local flow foliation and partly broken quartz phenocrysts, and was emplaced at 34.2 ±0.7 Ma. Padilla-Garza et al. (2004) noted it was overprinted by shallow high-sulphidation mineralisation, which as predicted by those authors, was subsequently found to be related to the deeper late mineral biotite granodiorite porphyry complex of the Escondida Este porphyry copper northern centre, ~800 m beneath the rhyolite porphyry (Fig. 7). This latter centre, which is described in more detail later, comprises an early porphyry, dated at 34.5 ±0.6 and 34.5 ±0.5 Ma, and at least two intermineral phases, and is ~2 m.y. or more younger than the main Escondida centre, but overprints and upgrades mineralisation in the latter. Some 2 km to the south, still within the Escondida Este deposit, similar intrusives are associated with the southern mineralised centre, but are overprinted by a ~33.3 Ma late inter-mineral granodiorite porphyry stock (Fig. 8).
Later still, dacitic and quartz-latite to rhyodacite porphyry dykes were intruded along NW trending, post-mineralisation faults, cutting the earlier intrusives, and are particularly abundant in the rhyolite porphyry. These dykes are weakly altered, with sericite K-Ar dates of ~31 Ma.
At Escondida Este, Escondida Norte and Pampa Escondida (Fig. 2), also described in more detail later in this record, mineralisation is mostly hosted by Palaeozoic rhyolitic porphyries of the La Tabla Formation. However, mineralisation is controlled by dykes of granodiorite and dacitic feldspar-biotite-quartz porphyry which outcrop at Escondida Norte-Zaldívar, and occur below the pit floor at Pinta Verde (Figs. 9 and 10). These dykes have been dated at 38.7 ±1.3 Ma (U-Pb in zircon) and 37.4 ±0.18 Ma (40Ar/39Ar). At Chimborazo, small, poorly altered late-mineral dykes of hornblende diorite and andesitic feldspar-biotite-quartz porphyry, similar to the mineralisation associated intrusive at Zaldívar, have been dated at 38.09 ±0.30 Ma (40Ar/39Ar; Richards et al., 2001; Padilla-Garza et al., 2001 and 2004).
At Pampa Escondida, a 37.6 ±0.5 Ma precursor hornblende diorite occurs as dykes cutting the La Tabla Formation (Figs. 9 and 12). These were followed by several phases of biotite granodiorite porphyry that intrude all the Early Permian rocks, the first phase of which has been dated at 36.1 ±0.7, 36.0 ±1.2, and 35.0 ±0.6 Ma, occurring as a NE-oriented, 2 x 0.5 km stock, the core of which is intruded at depths of >1000 m, by an inter-mineral granodiorite porphyry phase dated at 35.0 ±0.8 and 34.5 ±0.4 Ma. These are, in turn, cut by late inter-mineral, 35.2 ±0.8 Ma hornblende dacite porphyry dykes (Hervé et al., 2012; Hervé, 2010).
Late Eocene to Early Oligocene Silicified Breccias and Veining - Veining is widespread in faults of both the north-south and NW structural corridors, producing trails of quartz, jasper, carbonate or barite float which indicate concealed faults in areas of little of no outcrop. Barite veins more than a metre thick, some with copper colours, were pitted by early prospectors in the NW fault corridor between Zaldívar and Chimborazo. Closer to the porphyry centres, these vein are characterised by epithermal-style chalcedonic and vuggy quartz, passing into extensive zones of brecciation and silicification, as at Chimborazo and to the north and east of Zaldívar (Fig. 2). Alteration associated with these breccias is exemplified by the breccias some 10 km ENE of Escondida. At this location, pervasive silicification of fragmented volcanic country rock is accompanied by coarse grained hypogene alunite, granular diaspore and kaolinite, within a broader zone of argillic alteration. These breccias have some associated Cu mineralisation. Similarly, at Chimborazo, the deposit is adjacent to and over a prominent hill of silicified and brecciated andesitic lavas and felsic tuffs of the Augusta Victoria Formation, with potassic, propylitic and late advanced argillic alteration, and associated dykes of altered porphyry, as described above. Mineralisation at Chimborazo is mainly of supergene origin, hosted by the brecciated country rock volcanics (Richards et al., 2001).
Late Oligocene to Middle Miocene Pampa de Mulas piedmont gravels form a sub-horizontal, north and westward thickening wedge that is locally >300 m thick. They unconformably overlie the volcanic sequence and intrusions of the Escondida District and beyond, and were deposited in discrete tectonic basins associated with the Domeyko Fault System. They have the form of alluvial fans flanking areas of positive relief. The alluvial fans are dominated by braided rivers alternating with high energy non-channeled torrential flows of debris and sheet flow. In the Escondida District they contain abundant limonitic clasts of advanced argillic lithocap. Lateral transport of copper to form exotic copper also occurs in channels within the pile (Hervé et al., 2012; Centellas et al., 2013; Mort, 2019). These are part of the superficial cover omitted from Fig. 2.
Return to Top
ESCONDIDA DEPOSIT
Hypogene porphyry copper mineralisation at the main Escondida deposit is associated with an elliptical, 4.5 x 2.5 km, late Eocene to early Oligocene, composite granodiorite-porphyritic stock. This stock is elongated in a 140 to 150° direction and intrudes andesites correlated with the Paleocene to early Eocene Augusta Victoria Formation (Padilla-Garza et al., 2001; Padilla-Garza et al., 2004).
The composite granodiorite-porphyritic intrusive is known as the Escondida Stock and comprises at least four phases which collectively constitute the 'early granodiorite porphyry' (undifferentiated on Figs. 3 and 6). The two earliest are porphyritic and have a similar mineralogy. They may be distinguished however, on the basis of their phenocryst content, vein continuity and alteration intensity. The older of this pair is the Colorado Grande intrusion, which has been dated at 37.9 ±1.1 Ma (U-Pb zircon; Richards et al., 1999), and 37.7 ±0.8 and 37.2 ±0.8 Ma (U-Pb zircon; Padilla-Garza et al., 2004), and is cut by the second, the Escondida intrusion. The Escondida intrusion has been dated at 37.7 ±0.8 Ma. The Colorado Grande intrusion is a crowded porphyry with an average of 60% phenocrysts, while the Escondida intrusive has less than 40% (Padilla-Garza et al., 2001).
Phenocrysts from both the Colorado Grande and Escondida intrusions comprise quartz, orthoclase, plagioclase and biotite and, in general, range for 1 to 5 mm across, although they may occasionally be up to 8 mm. The quartz is subrounded, from 0.5 to 3 mm in diameter, and totals 2 to 8% of the phenocrysts. Plagioclase however, comprises 60 to 70 vol.% of the total phenocrysts, is of oligoclase to andesine composition, is euhedral to subhedral with concentric zoning, and may be 0.5 to 5 mm across. Subhedral, Carlsbad twinned orthoclase comprises 20 to 30% of the phenocrysts, and ranges from 0.5 to 3 mm in length. Euhedral biotite phenocrysts occur as brown books with a diameter of 0.5 to 1.5 mm and comprise around 1 to 3% of the phenocrysts. The groundmass is mainly composed of plagioclase, orthoclase, quartz and biotite as smaller crystals of <0.5 mm diameter (Padilla-Garza et al., 2001).
The third phase of the Escondida Stock is a 350° elongated, 1000 x 250 m zone which envelopes numerous relatively small porphyry breccias (Fig. 6), which have a similar composition and phenocryst content to the Escondida intrusion, but incorporates mineralised fragments of the previous two phases, while being crosscut by later mineralised veins. The overall fragment content averages 8%, although this may locally be as high as 60% where it has the texture of an intrusive breccia. The fourth phase comprises narrow granodioritic dykes containing copper and iron sulphides and associated quartz-sericite alteration, cutting all of the three previous intrusive phases (Padilla-Garza et al., 2001).
As detailed previously, the Colorado Grande and Escondida intrusions and associated copper mineralisation are cut in the north by the inter-mineral Colorado Chico biotite rhyolite dome with abundant quartz phenocrysts which has been dated at 37.5 ±0.6 Ma (U-Pb zircon; Hervé et al., 2012). All of these are, in turn, cut at depth by a larger mass of 35.4 ±0.7 Ma (U-Pb zircon; Hervé et al., 2012) late inter-mineral biotite granodiorite porphyry. Note, this lithology is not shown on Fig. 3 which is a surface map as known when published by Padilla-Garza et al. (2004), whilst it appears on Fig. 6 (after Hervé et al., 2012) when exposed in the deeper pit and intersected in peripheral drilling.
porphyry
Approximately 3 m.y. after the emplacement of the Escondida Stock and the development of the mineralised porphyry system, both were intruded and bounded to the east within the Escondida mine area by the large Cerro Sureste or Late Mineral rhyolite porphyry upward flaring dyke, dated at 34.2 ±0.7 Ma U-Pb zircon; Hervé et al., 2012). This rhyolite post dates the main Escondida mineralisation associated potassic and sericitic alteration, but is accompanied by a moderate to intense advanced argillic phase (Padilla-Garza et al., 2001 and 2004) related to the underlying Escondida Este porphyry copper complex, as described later.
The final intrusive episode is represented by narrow, copper-barren, but weakly sericite altered, rhyodacitic dykes that cut the rhyolite dome. Dating of the sericite alteration (K-Ar) yielded dates of 31.5 ±2.8 Ma, while whole rock samples gave 31.0 ±2.8 Ma (Padilla-Garza et al., 2001).
At least four types of breccia have been recognised within the Escondida Stock, namely: i). the intrusive breccias of the third phase of the stock, as described above; ii). cooling breccias at the contacts between the rhyolite dome and andesite country rocks;iii). mineralised and barren pebble dykes that post date the rhyolites; and iv). tectonic breccias of various ages (Padilla-Garza et al., 2001).
The mineralised Escondida Stock is cut by two main fault systems, namely: i). mineralised faults, comprising both a north-south to NNW-SSE set and an associated secondary WNW trend; and ii). NE trending, dextral displacement post-mineralisation faults (Padilla-Garza et al., 2001).
The mineralised faults of the north to NNW trending set have an average strike of 350° (varying from 340 to 20°), parallel to the main Domeyko Fault Zone. There are five main faults in this system in the mine area, the Ferrocarril, Portezuelo, Ortiz, Panadero and Zaldívar faults (Figs. 2 and 3). The two faults to the west, the Ferrocarril and Portezuelo, dip east, while the remainder, which are to the east, dip west (Fig. 3). At around the level of Fig. 3, these faults are represented by corridors of brittle, cataclastic deformation, ranging from 50 to 250 m in width. Each has associated parallel zones of strong alteration carrying veins with early quartz-sericite and late advanced argillic alteration. In addition, the majority of the pebble dykes and late barren rhyodacitic dykes have the same NNW preferred orientation. Each of the main fault zones is composed of a series of discrete, parallel, undulose planes of fractured rock and gouge which have maximum strike lengths of <1 km and are up to 0.5 m thick. The continuity of each of the main fault zones results from the persistent development of the parallel, overlapping planes throughout its length. These faults are more continuous within the andesitic country rocks than within the intrusives. The secondary WNW trending set of mineralised faults strike at between 290° and 340°, dip to the south and are parallel the cross-orogen Archibarca lineament (Figs. 1, 2 and 3) (Padilla-Garza et al., 2001; Richards et al., 2001).
The NE trending, dextral displacement post-mineralisation faults dip at 60° to 70°S and are characterised by a series of thin, 0.3 m thick, continuous and highly fractured zones of red clay gouge with sharp contacts. Some are continuous over strike lengths of more than 2 km and are current pathways of meteoric water. Lateral displacement on these fault zones is generally less than 50 m (Padilla-Garza et al., 2001). A major example of this set is the 70°SE-dipping Hamburgo reverse fault (Fig. 2), the southernmost of several such structures along the eastern side of the deposit area, that were produced by clockwise rigid-block rotation about vertical axes coeval with a component of sinistral motion on the N-striking faults (Mpodozis et al., 1993; Arriagada et al., 2000). The accommodation space generated incrementally during this rotation was filled by the San Carlos strata (Hervé et al., 2012).
Stress analysis suggests that both the mineralised and post-mineralisation faults are related to the dominantly transcurrent Domeyko Fault system, with NW-SE and WSW-ENE directed maximum compressive stress axes respectively (Padilla-Garza et al., 2001).
Hypogene Mineralisation
Hypogene mineralisation and alteration at Escondida went through three stages of progressive development. Each overprinted the preceding stage and corresponded to a separate phase of hydrothermal activity. Understanding of the early stages in particular, is complicated by the high level, deeply penetrating effects of the third stage, a late advanced argillic event, and by the subsequent supergene oxidation, leaching and concentration. Both of these late events were accompanied by pervasive alteration which modified both shallow and deep hydrothermal characteristics. In addition, the fact that specific alteration minerals may occur in different stages, and different zones within those stages, further confuses the issue. The characteristics of the stages may be summarised (Fig. 4) as follows, from Padilla-Garza et al., (2001) and Padilla-Garza et al., (2004).
Stage A Alteration and Mineralisation - Stage A hydrothermal activity is reflected as both pervasive and fracture controlled alteration and mineralisation. It is also complicated by the overprinting and partial to strong replacement associated with the Stage B phyllic alteration and mineralisation, only being preserved as relicts and overprinted zones of partial replacement, e.g., the stage B biotite to chlorite zone (Fig. 4), where stage A pervasive fine grained biotite is partially replaced by stage B chlorite.
Two zones of Stage A pervasive alteration are evident, specifically, an inner potassic and an outer propylitic zone.
The potassic phase, is lithologically controlled, as follows: i). Within the Escondida Stock, weak, disseminated K feldspar replaces plagioclase phenocrysts and groundmass to produce a pink tinge in the rock. Locally, significant masses (of the order of cubic metres) have been altered to orthoclase, although much of the K feldspar alteration is found in the vicinity of discontinuous quartz and quartz-orthoclase stockwork veins. ii). Within the andesitic country rock, mafic minerals in both the phenocrysts and groundmass are extensively, and locally completely altered to biotite, accompanied by minor and vein controlled K feldspar and anhydrite. Most of the biotite is fine grained (<0.1 mm) and developed in the groundmass, while the larger phenocrysts are commonly only altered in their outer fringes. This zone of biotite development partially fringes the intrusive with a semi-elliptical/crescent shape, grading outwards into propylitic alteration. iii). At the contact between the Escondida Stock intrusives and the andesitic country rock, there is a 20 to 40 m wide shell of pervasive texture destructive silicification which has altered both lithologies, and is interpreted to represent a contact metasomatic phenomena. It takes the form of an aphanitic, hard and strongly fractured rock, comprising fine grained aggregates of <50 µm quartz grains. This silicification is one of the earliest alteration events, cut by veins associated with all of the following alteration assemblages described below (Padilla-Garza et al., 2001).
Propylitic alteration at Escondida is characterised by the i). sporadic conversion of plagioclase to grossular and more frequently to epidote and montmorillonite; ii). modification of hornblende and biotite to chlorite; iii). partial albitisation of plagioclase; and iv). by widespread carbonate and zeolite veining. The resultant assemblage comprises epidote, with lesser chlorite, montmorillonite, minor biotite and grossular, and widespread carbonate and zeolite veins (Fig. 4; Padilla-Garza et al., 2001). The propylitic zone forms a wide halo enveloping the deposit (Fig. 4) and the larger mineralised system of the district, encompassing all of the significant deposits (including Zaldívar-Escondida Norte-Pinta Verde and Chimborazo as well as Escondida) over an area of some 25 x 15 km (Fig. 2; Richards et al., 2001). The latter authors describe this semi-regional propylitisation, more distal from the immediate deposits, as being characterised by chlorite, developed as pseudomorphs after biotite or hornblende. Hervé et al. (2012) do not define a propylitic alteration zone at Escondida, but rather outline broad halos of chlorite-sericite alteration in the La Tabla Formation to the east, and chlorite>K feldspar in the Escondida inter-mineral granodiorite porphyry to the west, which are all proximal to the major deposit (Fig. 7).
Veining associated with stage A, which compares closely with the A-type veins of Gustafson and Hunt (1975), represent the transition from the pervasive phase of alteration that dominates stage A, to the more vein controlled alteration of stage B. The earliest veins are composed of quartz or quartz-orthoclase ±biotite ±anhydrite, and are currently best preserved at depth to the northwest, although relicts can be seen throughout the deposit, reopened by later vein stages. These stage A veins can be sub-divided into (Padilla-Garza et al., 2001; 2004):
i). Substage A-a - which are strongly sinuous, discontinuous (generally <100 mm long, but 1 to 20 mm thick), have no alteration halo and are filled with quartz, occurring mainly within the Escondida Stock, but also just beyond the contact within andesite country rock.
ii). Substage A-b - which are still irregular, but more continuous and less sinuous than substage A-a veins, and are filled with quartz-K feldspar ±biotite-anhydrite. They are 1 to 30 mm thick and are characterised by halos of pink feldspar making the vein margins diffuse and very irregular.
iii). Substage A-c - although still irregular, these veins are more continuous than either of the preceding veins, with lengths of up to 700 mm and thicknesses of from 10 to 50 mm. Their walls are well defined with no alteration selvages. They are filled by quartz, accompanied by chalcopyrite and bornite.
The three vein stages exhibit multiple pulses of emplacement, although in most cases substage A-b and A-c postdate substage A-a veins (Padilla-Garza et al., 2001).
Mineralisation associated with the stage A hydrothermal activity was characterised by a magnetite-bornite-chalcopyrite assemblage with generally <0.5 vol.% sulphides in the potassic zone. However, at a microscopic scale, it is common to see stage A veins opened and crosscut by younger stage veins. Copper grades in zones of potassic alteration and only weak overprinting by stage B and C alteration and mineralisation, normally have grades of <0.3% Cu, although values are dependent upon the alteration assemblage. For example, andesites which have been pervasively biotite altered, contain up to 2 vol.% magnetite as generally <60 µm grains, <0.5 vol.% sulphides and <0.2% Cu. As the proportion of K feldspar increases, the magnetite decreases and the sulphide content becomes higher. Where K feldspar predominates, the host-rock contains closer to 0.5 vol.% sulphides, comprising 90%
chalcopyrite and <10% bornite of 20 to 600 µm grain size, and grades of <0.1 to 0.3% Cu (Padilla-Garza et al., 2001). Within the propylitic zone, pyrite is the dominant sulphide, although locally there are small volumes of veins carrying both pyrite and chalcopyrite. Grades are generally <0.1% Cu (Padilla-Garza et al., 2001).
To date no high grade potassic core has been encountered, with all holes below the zones of overprinting stage B and C veining being low grade (~0.2% Cu) and low fracture density (0.2/cm), in contrast to the high fracture densities (1/cm) where the system is cut by the veins of stages B and C (Padilla-Garza et al., 2001).
Stage B Alteration and Mineralisation - Stage B hydrothermal activity produced predominantly fracture controlled alteration and mineralisation, with the alteration concentrated in selvages adjacent to veinlets. Two successive alteration assemblages were developed, specifically an earlier chlorite-sericite ±quartz, and a later quartz-sericite stage.
The assemblages produced by chlorite-sericite alteration are dependent upon the pre-alteration mineralogy of the intrusive and country rocks. Within biotite altered andesite, disseminated and vein controlled chlorite-sericite replaces biotite. Within the Escondida Stock, there is a selective alteration of biotite and other mafic minerals and of stage A K feldspar to chlorite-sericite, accompanied by the introduction of smaller volumes of chlorite in fractures and re-opened earlier veins. In general, this process is texture preserving, except in the cores of veins and their immediate inner alteration halos. Towards the outer margin of the chlorite-sericite zone, mainly in andesites, chlorite predominates, while sericite is more prevalent closer to the stock. The chlorite-sericite zone is 1 to 2 km wide and open to the NE and SE of the deposit. The fracture density in this zone is moderate and the alteration is a dark to pastel green colour.
Substage B-a veining is associated with the chlorite-sericite alteration phase. These veins cut or re-opened stage A veins and have lengths of from a few tens to 500 mm, with thicknesses of <10 to 30 mm. As such they are more continuous than the stage A veining, although their margins are still irregular and diffuse, and they are sinuous with branching geometries. Within andesite, substage B-a veins have a <10 mm core filled by sulphides and only minor quartz, sandwiched by a halo of sericite with subordinate chlorite, grading out into predominantly dark green chlorite (Padilla-Garza et al., 2001).
Substage B-b alteration is vein-controlled, occurring as halos of a pale green to grey quartz-sericite alteration assemblage. This alteration is best developed within intrusive rocks and is stronger along fault zones. Associated sulphides are chalcopyrite ±pyrite-mobybdenite.
Substage B-c veins occur in association with the last sericitic event which produced selvages of white sericite with disseminated pyrite. This alteration is also vein controlled and occurs as strong halos to pyrite ±chalcopyrite veins.
The quartz-sericite assemblage represents the dominant alteration within the core of the deposit and is located mainly in the southeastern portion of the Escondida Stock, and in the adjacent andesites, occurring mainly in veins and in their selvages. Where most strongly developed, it is a texture destructive alteration, with intense substage B-b and B-c veining cutting a white sericite-quartz mass in which only the quartz phenocrysts of the original porphyry are recognisable. These veins, which are composed of quartz and sulphides, are usually >200 mm up to 1 m in length, and vary from 10 to 150 mm in thickness. They have irregular selvages, with a mottled pastel green, dark grey and white colouration, and grade from intense (texture destructive) to moderate to weak (where original rock textures are recognisable) quartz-sericite alteration (Padilla-Garza et al., 2001).
Within the stage B alteration zone, the average sulphide content increases to as much as 2 vol.%, with chalcopyrite being dominant, accompanied by lesser pyrite and minor molybdenite, for a chalcopyrite:pyrite ratio of 3:1. Where best developed, hypogene grades vary from 0.4 to 0.6% Cu. Substage B-a chlorite-sericite ±quartz veins in andesite carry up to 20% of 50 to 500 µm sized sulphides in thin veins, while their halos have <2% sulphide which are <30 µm. Within the Escondida Stock, quartz of the quartz-sericite substage B-b and B-c veins carries very fine sulphides in <20 µm cracks between grains, and as up to 900 µm diameter sulphides within quartz crystals. Within the intense quartz-sericite halos there are up to 10% sulphides that vary from 50 to 600 µm across, grading outwards to 2% as 30 to 300 µm grains in the weakly altered fringes. The substage B-c veining represents the final phase of the stage B hydrothermal activity. It is characterised by dominant white sericite and pyrite within the core of the deposit and along the main fault zones, and has up to 5% sulphides, with the chalcopyrite:pyrite ratio being reduced to 1:3. The vein filling is commonly subhedral to euhedral pyrite with diameters up to 3 mm (±chalcopyrite), embraced by a quartz-sericite halo (Padilla-Garza et al., 2001; Padilla-Garza et al., 2004).
Stage C Alteration and Mineralisation - Stage C is the final, two pulse hypogene hydrothermal event at Escondida, dated at 36 and 34 Ma respectively. It is characterised by polymetallic sulphide veins that vary in width from a few millimetres to 3 m, and are oriented at 320°. Both pulses post date the emplacement of the Colorado Chico and the Sureste rhyolites, while stages A and B were entirely prior to these rhyolite intrusions (Padilla-Garza et al., 2001; Padilla-Garza et al., 2004).
Stage C represents acid-sulphate (advanced argillic) alteration that affected the andesites, Escondida Stock and the two rhyolites. Its distribution is controlled by the main fault zones, and by the contacts between the rhyolites and the intruded rocks. The altered zones are narrow and closely controlled by faulting at depth, but flare outward at shallower depths. The alteration is defined by pyrophyllite, alunite and quartz, the presence of banded quartz veins and abundant sulphides of a high sulphidation assemblage.
The mineral assemblages encountered within this stage, commenced with substage C-a comprising quartz-pyrite-enargite, followed by substage C-b of bornite-chalcopyritepyrite, then substage C-c of sphalerite-pyrite, and locally developed substage C-d containing pyrite-molybdenite. Padilla-Garza et al., (2001) note that each of these substages largely involves the re-opening of veins mineralised during the previous substages to emplace a new layer. They further added, that not all of the stage C veins carried the full paragenetic assemblage, although all contain pyrite. At the margins of the veins there are bands of fine grained (up to 500 µm) sugary quartz and fine grained, semi-translucent quartz (<70 µm) that are <10 to 100 mm thick.
Beyond the walls of these veins, finely disseminated pyrite is found within the pervasive acid-sulphate alteration. All of the preceding were deposited in association with sericite and pyrophyllite, but in the absence of alunite. The terminal advanced argillic phase is substage C-e, marked by pyrite-alunite, in veins that are locally brecciated and cemented with alunite (Padilla-Garza et al., 2001; Padilla-Garza et al., 2004).
Where stage C veining intersects veins containing copper mineralisation emplaced during an earlier stage, the copper grade is normally enhanced to be from 0.6 to >1% Cu and the pyrite:chalcopyrite ratios are >5:1 (Padilla-Garza et al., 2001; Padilla-Garza et al., 2004). Stage C veins have been compared to the D-type veins of Gustafson and Hunt (1975) (Ojeda, 1990).
Stage C is interpreted to largely be related to the deeper Escondida Este porphyry system that was emplaced below Escondida and immediately to the east during this same time interval. See the Escondida Este description below and Fig. 7
Chronology and Paragenesis - Field relationships show that the stage A alteration and mineralisation, overprints the Colorado Grande and Escondida intrusives of the Escondida Stock. The ages of the intrusives and the stage A alteration are in the approximate range of 38 to 37.5 Ma although 40Ar/39Ar dating of hydrothermal biotite alteration at Escondida by Padilla-Garza et al. (2004) yielded ages of 37.5 ±0.6, 36.0 ±0.4 and 37.5 ±0.6 Ma. The older limit of emplacement of the inter-mineral Colorado Chico rhyolite dome, which has been dated at 37.5 ±0.6 Ma (U-Pb zircon; Hervé et al., 2012), overlaps the younger age limit of the Colorado Grande porphyritic granodiorite, suggesting the system evolved over a relatively short time frame. Stage B hydrothermal activity is shown by field relationships to post-date stage A, but to predate intrusion of the Colorado Chico rhyolite dome (Padilla-Garza et al., 2004).
Dating (40Ar/39Ar) of alunite samples from stage C polymetallic veins cutting the Colorado Chico rhyolite dome has provided ages of 35.7 ±0.3 and 35.2 ±0.3 Ma, while Re-Os dating of molybdenite of substage C-d yielded an age of 33.7 ±0.3 Ma indicating that stage C represented a separate hydrothermal event at the Escondida deposit. Emplacement of the late-mineral Cerro Sureste rhyolite has returned an age of 34.2 ±0.7 Ma (U-Pb zircon; Hervé et al., 2012). The latter is cut by barren rhyodacite dykes which confirm its emplacement near 34 Ma (Padilla-Garza et al., 2004).
Hypogene copper grades associated with stage A potassic alteration, with little input from stages B and C, are typically <0.3% Cu. However, in areas of intense chlorite-sericite and quartz-sericite overprint, grades are, on average, between 0.4 and 0.6% Cu, and locally where stage C veins cut earlier stage mineralisation, the hypogene grades may reach 1% Cu (Padilla-Garza et al., 2004).
Isotopic data indicate that stages A and B alteration and mineralisation was related to progressively evolving magmatic hydrothermal fluids, and that stage B introduced new copper, rather than redistributing stage A metal. Stage A represents prograde porphyry style mineralisation characterised by a potassic core and propylitic periphery, whilst stage B is the subsequent retrograde phyllic alteration overprint as the hydrothermal system waned and cooled and fluids cooled higher in the system are refluxed back down to mix with the rising hotter fluids. The phyllic alteration is represented by sericite in felsic hosts and is more chloritic in protoliths with a higher proportion of mafic lithologies/earlier alteration. Isotopic data also suggest the early phases of stage C, particularly the pre-alunite pyrite-enargite and sphalerite-pyrite substages, involved leaching and redeposition of metal from the earlier stages of mineralisation. However, the isotope data from galena in alunite bearing veins imply both leaching of earlier sulphides and the addition of sulphide from outside of the system. It seems likely that as the Escondida system waned, fluids escaping from the ~2 m.y. younger Escondida Este at greater depth and ~1 km to the east, produced a lithocap, represented by stage C, overprinting the Escondida stages A and B mineralisation. This lithocap is represented by advanced argillic alteration and a high sulphidation assemblage.
This interpretation is consistent with the conclusion by Hervé et al. (2004) that the cluster of Escondida deposits appears to have formed in two separable, but partly overlapping stages: i). early Escondida, Escondida Norte-Zaldívar and Baker porphyries at ~38 to 37 Ma, involving stages A and B, followed by the ii). later Pampa Escondida and Escondida Este porphyries at ~36 to 34.5 Ma and Stage C alteration and mineralisation. Other than relatively rare dykes, no further significant magmatism is recorded in the district after ~34 Ma.
Supergene Mineralisation
The Escondida deposit had a thick, areally extensive, and well developed supergene sulphide enrichment blanket, with an average grade of more than 1.1% Cu, based on a 0.3% Cu cutoff. In 1999, this blanket accounted for 65% of the total resource at Escondida, whilst the earlier initial mineable reserve comprised 662 Mt @ 2.12% Cu, parts of which averaged >3% Cu (Ojeda, 1990). These ratios have substantially changed as cut-off grades have been lowered and as further drilling has outlined larger tonnages of hypogene mineralisation. This enrichment blanket is overlain by a leached cap with associated zones of oxide ore and perched/residual supergene sulphide blankets (Padilla-Garza et al., 2001).
The major Domeyko Fault (West Fissure) Zone structures were reactivated after deposition of the hypogene mineralisation to downthrow a block between the Portezuelo and Panadero faults (Figs. 3, 4 and 6) containing the core of the hypogene orebody. Uplift, erosion, oxidation and lowering of the water table in an arid climate led to the development of a downward migrating leached cap and underlying supergene sulphide enrichment blanket between 18.0 and 14.7 Ma, possibly principally controlled by reactivation of the NW trending set of faults (Ojeda, 1990).
Supergene Enrichment Blanket - The supergene enrichment blanket has dimensions of ~4.5 x 1 km, and varies from a few metres to a maximum of 400 m in thickness (Figs. 3 and 5), averaging ~200 m, with the long axis oriented NW, parallel to the main direction of cross faulting and vein system direction (Ojeda, 1990). The upper surface of the blanket is subhorizontal, offset vertically in several places across fault zones, while the lower limit is irregular, making the thickness variable (Padilla-Garza et al., 2001). Highs and depressions in the base of the blanket tend to be elongated parallel to the same NW fault trend, although secondary north-south and east-west elongations of highs and lows are also observed (Ojeda, 1990). Depressions in the base of the blanket are also related to fault associated fracturing, influencing the downward access of meteoric water. In general, grades within the blanket vary from 0.3% to >2% Cu, and in places may exceed 3.5% Cu. The high grade enrichment ore resource averages over 1.1% Cu, with substantial additional tonnages of lower grade 0.6 to 0.7% Cu (Padilla-Garza et al., 2001; BHP Billiton, 2003). The highest hypogene copper grades appear to have been the main controls on both the form and depth of the enrichment zone (Ojeda, 1986, 1990; Padilla-Garza et al., 2001).
Enrichment ore has been defined by the presence of chalcocite and covellite equal to, or greater than, 10% of the total contained sulphides (Ojeda, 1990). Sulphides occur as disseminations and in veins and include chalcocite, covellite, with minor digenite and idaite, replacing grains of bornite, chalcopyrite and pyrite. Chalcocite is found throughout the full thickness of the blanket, with the upper portion of the zone of strong enrichment being dominated by whitish grey chalcocite, probably mostly djurleite. Covellite and digenite appear near the middle and increase in volume downward. As the abundance of supergene digenite(-anilite; Cu7S4) increases downwards, chalcocite(-djurleite; Cu31S16) rims on pyrite decrease in thickness. This assemblage distribution results in the division of the blanket into an upper, higher grade zone dominated by chalcocite-group minerals, with lower grade covellite and remnant hypogene sulphides becoming dominant downward. The generalised zonation of sulphides from hypogene ore at the base of the blanket to the top is as follows: chalcopyrite → covellite → digenite(-anilite) → djurleite(- chalcocite) (Alpers and Brimhall, 1989).
Alpers and Brimhall, (1989) noted that supergene alteration at Escondida has resulted in the development of kaolinite, gypsum and alunite from the destruction of feldspars, chlorite, biotite and anhydrite. They add that supergene kaolinite is rare to absent from the zones of original pervasive advanced argillic alteration and mixed quartz-sericite and advanced argillic assemblages. However, it increases with depth in the mixed quartz-sericite - potassic zones, probably as a result of the absence of the feldspars, chlorite and biotite that kaolinite replaced during hypogene alteration. They also reported that supergene kaolinite alteration and dissolution of hypogene anhydrite persisted to depths several hundred metres below the top of the enriched sulphide blanket, and well below the base of strong copper enrichment. The influence of these supergene processes persisted into zones of only poorly enriched protore, which have never-the-less, experienced measurable changes to characteristics, such as mineralogy, density and porosity. Supergene alunite, which ranges in age from ~18
to 14 Ma (K-Ar alunite; Alpers and Brimhall, 1988) is distributed throughout the advanced argillic and quartz-sericite zones. It occurs as cryptocrystalline veins and fracture coatings up to 10 mm thick, and as fine grained disseminations, and persists to 50 m below the top of the enriched sulphide blanket. Supergene alunite has been extensively replaced by jarosite within the leached cap (Alpers and Brimhall, 1989).
The zone of highest grade and thickness of enriched ore corresponds to the interval of highest hypogene grade, associated with the greatest intensity of hypogene stockwork mineralisation, as detailed above. This zone is located within the downthrown block between the Portezuelo and Panadero faults, where strong quartz-sericite and advanced argillic events overprint early potassic alteration (Ojeda, 1990; Padilla-Garza et al., 2001). In this interval, chalcocite reaches 7 to 8% of the rock volume, and accounts for 90% of the copper sulphides (Ojeda, 1990). The chalcocite(-djurleite) has virtually replaced all of the hypogene chalcopyrite and bornite as well as some of the pyrite. The content of pyrite is very variable through the profile due to the presence of pyritic veining (Alpers and Brimhall, 1989). The floor to the higher hypogene and hence supergene grades is due to the intrusion of the late intermineral biotite granodiorite porphyry into the host porphyry at depths of >500 m (Hervé et al., 2012).
Leached Capping - The supergene sulphide enrichment blanket at Escondida is overlain by a thick zone of leaching, the upper limit of which is essentially the current land surface, below a thin veneer of superficial gravels. Its base varies from a few metres below the surface to depths of more than 200 m over the main supergene enrichment blanket. The peaks of the higher hills prior to mining were originally more than 350 m above the enrichment blanket. The leached capping, as currently exposed, represents an earlier supergene enriched blanket which has been leached as uplift, oxidation and leaching progressed and the zone of enrichment moved correspondingly downwards. Strong leaching of the preceding hypogene and supergene copper sulphide mineralisation has left residual values of <100 to 600 ppm Cu and 10 to 480 ppm Mo in the capping, within a porous rock devoid of hypogene sulphides, but containing variable amounts of limonite in their place. The composition of the limonite has a good correlation with the supergene grades and thicknesses of the underlying supergene blanket. Hematite generally predominates above the thicker and higher grade central sections of the enrichment blanket, indicating leaching from an earlier chalcocite enrichment blanket. Jarosite is the principal oxide over the thinner zones of lower grade, which are found mostly on the margins of the blanket, overlying the pyritic fringe of the hypogene mineralised system. Goethite overlies the oxide ores in the western part of the deposit (Alpers and Brimhall, 1989; Ojeda, 1990; Padilla-Garza et al., 2001).
A study of the limonite of the leached cap allows the reconstruction of the earlier, now leached enrichment blanket. This former blanket contained a number of restricted zones of >1.5% Cu, with a cumulative surface area of <0.2 km2. By comparison, the area averaging >1.5% Cu in the current enrichment blanket is more than 2.0 km2, emphasising the process of cumulative downward enrichment, from the original lower grade hypogene mineralisation, through progressive stages of supergene enrichment and leaching (Alpers and Brimhall, 1989).
The leached capping at Escondida, like many developed over porphyry copper mineralisation in the central, most arid portion of the Atacama desert, have been subjected to super-leaching. This process involves significant modification by the remobilisation of quartz and other rock constituents, and the removal of limonite from relict sulphide cavities. The process is believed to be related to the cyclic migration of the salts abundant in the upper 1 to 3 m of the soil profile in that part of the Atacama desert. These salts are apparently transported by capillary action in the soil and the upper parts of weathered bedrock, being dissolved and then re-precipitated. The crystallisation within fissures and cavities causes a fracturing of the rock mass, much like “ice-wedging”, the modification of these openings, and the “plucking” of limonite after sulphides. The salt dissolution is also believed to promote chemical modification of the rocks involved. In the leached cap at Escondida, this process is interpreted to have resulted in the destruction of much of the surface evidence of hematitic “live limonite”, which is diagnostic of leached chalcocite (Lowell, 1991).
Perched Enriched Sulphide Lenses - Intervals of perched or residual supergene sulphide mineralisation have been encountered within the leached cap, and are the result of incomplete leaching of the earlier supergene blanket. Thicknesses encountered in drill holes vary from 0.1 to 30 m and usually correspond to enriched thick stage C massive sulphide veins or to fault zones above the current sulphide blanket (Ojeda, 1990).
Oxide Ore - A NW oriented 1500 x 200 m zone of copper oxide ore was developed on the southwestern margin of the Escondida Stock (Fig. 3), over a thickness of 50 to 200 m. Grades vary from 0.2 to 1.5% Cu (Padilla-Garza et al., 2001), with the oxide reserve grade in 2002 being 0.65% Cu (BHP Billiton, 2003). The 'oxide' mineralisation comprises a grouping of non-sulphide minerals, predominantly oxides, silicates, sulphates and carbonates which include brochantite, antlerite, atacamite, chrysocolla, 'copper-wad' and tenorite. It occurs mainly along fractures within andesites that have been subjected to hypogene biotite and chlorite-sericite alteration, although it also occur in lesser volumes over zones of hypogene K feldspar alteration within the Escondida Stock (Padilla-Garza et al., 2001; Ojeda, 1990). This mineralisation is characterised by the absence of sulphides and occurs within the zone of oxidation above the supergene sulphide enrichment blanket normally occupied by the leached cap (Padilla-Garza et al., 2001; Ojeda, 1990).
Return to Top
ESCONDIDA ESTE DEPOSIT
The Escondida Este deposit, as defined by the >0.3% Cu contour, occurs over a depth extent of >300 m to ~2000 m beneath the surface at its centre. The main mineralised zone has a north-south elongated lateral extent of ~4.5 x 1 km, bounded to the west by the Panadero Fault. The same structure also defines the eastern margin of the shallower, older, main Escondida deposit. This fault zone broadly corresponds to the regional structural contact between the Late Paleocene to Early Oligocene Augusta Victoria Formation into which the Escondida Stock is intruded, and the Permo-Carboniferous La Tabla Formation to the east that includes the country rocks enveloping the Escondida Este Intrusive Complex, although the latter in part also cuts the younger formation.
The ~34 Ma granodioritic porphyry suite of the Escondida Este Intrusive Complex occurs as two connected centres of mineralisation to the east of the ~38 to 37 Ma mineralised granodioritic porphyries associated with the main Escondida deposit. The northern of these two centres lies beneath a weakly mineralised inter- to late-mineral quartz porphyry dome (the Cerro Sureste rhyolite; Fig. 7) that post-dates the main Escondida mineralisation, whilst the southern zone is concealed by a sequence of Miocene gravels (Pizarro et al., 2015).
Precursor amphibole diorite and monzodiorite intrusions occur to the east of the southern mineralised centre at Escondida Este. These have been dated at ~36 Ma and older (U-Pb zircon; Pizarro et al., 2015). These may represent either a pre-mineral phase of the Escondida Este Intrusive Complex, or a waning phase of the earlier, generally 43 to 38 Ma precursor hornblende diorites found across the district.
The Escondida Este Intrusive Complex is characterised by multiple pulses of granodioritic porphyry stocks between 34.5 and 33.7 Ma (U-Pb zircon) and a 33.3 Ma (U-Pb zircon) late-mineral porphyry stock of granodioritic composition, as illustrated on Figs. 7 and 8 (BHP Billiton, 2014; Pizarro et al., 2015). To the west, the complex is bounded by a 34.2 ±0.7 Ma, upward-flared, late-mineral rhyolite porphyry (Ojeda, 1986, 1990), the Cerro Sureste rhyolite of Padilla-Garza et al. (2001), which exhibits local flow foliation and partly broken quartz phenocrysts (Hervé et al., 2012). The north-trending, dyke-like form of this rhyolite porphyry has been interpreted to suggests control by the Panadero Fault, although post-mineral fault displacement is relatively minor (Hervé et al., 2012). A late inter-mineral granodiorite porphyry dated at ~33.3 Ma intrudes the southern mineralised centre to produce a low-grade core (Fig. 8; BHP Billiton, 2014). It is uncertain whether a direct correlative phase is also present in the northern mineralised centre, although diagrams in BHP Billiton (2014) infers this is the case.
Small late-stage magmatic-hydrothermal breccias are widespread at Escondida Este, with most being clast supported, monomict, and cemented by high-sulphidation sulphide assemblages and anhydrite. Even later, barren, NW-striking pebble dykes are also mapped.
In the deposit area, the La Tabla Formation comprises a Permo-Carboniferous succession of bimodal andesitic and rhyolitic volcanic and coeval intrusions. The latter include a large, 296.8 ±3.2 Ma stock of quartz monzodiorite porphyry, 296.6 +4.4/-3.8 Ma monzogranite porphyry dykes, and, at a depth of ~2000 m, a foliated phaneritic tonalite, dated at 300.1 ±3.5 Ma (Hervé et al., 2012). These intrusive phases appear to be better developed around the northern mineralised centre, fading to the south.
The earliest major alteration is potassic, and includes both K feldspar and biotite assemblages which are found at depths of more than ~1800 m below surface. This alteration is cut by a range of veinlet types, including early biotite and sparse early dark micaceous veinlets. The potassic zone is overprinted between ~1800 and 1000 m depth by grey sericite (±andalusite) alteration dated at 31.8 Ma (Ar-Ar sericite) that is paragenetically and spatially related to the bulk of the copper mineralisation. This sericite alteration is the strongest hydrothermal event, and is centred at the top of the early-mineral porphyry stocks. It occurs as centimetre scale halos to discontinuous quartz-anhydrite veinlets that, where densely developed, partly coalesce to produce pervasive sericitisation. Outward, this zone grades into chlorite-sericite alteration. The ~34 Ma late-mineral rhyolite porphyry immediately to the west (Fig. 7), has undergone white sericitic and advanced argillic alteration, which completely dominate shallow levels above the main Escondida Este deposit. The grey and white sericite zones differ in that the former is richer in magnesium and iron (phengite) than the shallower, lower temperature white muscovite variety (Hervé et al., 2012).
Hypogene copper mineralisation is disseminated and vein-hosted within the deep, potassic-altered rocks where it occurs as chalcopyrite-pyrite, whilst the grey sericite zone is bornite rich at depth, with chalcopyrite increasing in its upper sections. Both copper sulphide minerals mainly occur as disseminated grains in veinlet halos. The highest copper and molybdenum grades are localised within the grey sericite zone, with the latter hosted within pre-existing B-type quartz and later pyrite veinlets. Overall, molybdenum was deposited in three pulses, with spatial and temporal relations between 34 and 33 Ma (Re-Os molybdenite; Padilla-Garza et al., 2004), associated in part with the early potassic alteration stage and in part with subsequent intrusion of the ~33.3 Ma late-mineral granodioritic porphyry of the southern mineralised centre. The top of the high grade hypogene mineralised zone is ~900 m below the current surface.
The grey sericite zone is overlain and overprinted by disseminated, high-sulphidation assemblages with higher grades than the surrounding hypogene mineralisation. These assemblages occur in association with a series of NW-trending, steeply dipping, banded, massive sulphide veins that are up to 3 m thick, cutting the granodiorite porphyry, as well as in faults and fractures farther east and west. The veins contain a range of high-sulphidation assemblages, including abundant pyrite accompanied by one or more of enargite, tennantite, chalcopyrite, bornite, covellite and chalcocite. They also include minor amounts of gold. Sphalerite is particularly abundant in the youngest vein fillings (Padilla-Garza et al., 2001, 2004; Quiroz, 2003). Vein halos are up to 1 m wide and include pyrophyllite, dickite, kaolinite and alunite, with subordinate diaspore, andalusite and corundum (Padilla-Garza et al., 2001; Quiroz, 2003; Véliz, 2004), which are transitional to sericitic alteration at depth.
The distribution of hypogene mineralisation and alteration at the northern mineralised centre at Escondida Este (Fig. 7) would appear to bear a close relationship to the Early Permian quartz monzonite porphyry and Late Carboniferous tonalite, and may reflect an influence of either competence or composition on alteration and mineral precipitation. Superficially, the higher grades in the southern mineralised centre appear to more closely spatially coincide with the rhyolitic facies of the La Tabla Formation. However, in both, mineralisation is centred on the ~34 Ma granodiorite porphyry.
Hypogene mineralisation dominates at Escondida Este, which is too deep to have allowed ingress of meteoric waters. However, an immature supergene chalcocite blanket is developed in the uppermost part of the shallower southern mineralised centre, 150 m beneath the current surface, where it underlies Miocene gravel cover, shallowly underlying the palaeosurface.
As shown on Fig. 7, Escondida and Escondida Este are connected by a neck of >0.3% Cu that transects the strongly sericite altered late-mineral rhyolite (Cerro Sureste) porphyry. While Escondida and Escondida Este are temporally separate porphyry systems, the advanced argillic alteration at both appears to belong to the same event (see the Escondida section above), while the pattern shown on Fig. 7 infers the phyllic alteration in the two deposits may well overlap.
Mineral Resoures at Escondida Este are not differentiated, but are amalgamated with that of the Escondida and Escondida Norte estimate. However, in 2015, BHP Billiton in a US SEC Form 6-K report quoted a JORC 2012 compliant potential size at February 2012 of between 9 and 19 Gt @ 0.4 to 0.6% Cu, 150 to 200 ppm Mo, at a 0.3% Cu cut-off. An update advised that, of this, 4 Gt had been converted to Inferred Mineral Resources in March 2014.
The information in this Escondida Este summary is largely drawn from Hervé et al. (2012) and BHP Billiton (2014).
Return to Top
ESCONDIDA NORTE DEPOSIT
The Escondida Norte porphyry copper deposit is located ~5 km north of the main Escondida pit and is contiguous with the Zaldívar deposit immediately to the west (Fig. 2). Mineralisation is hosted by volcanic rocks of the latest Carboniferous to Early Permian La Tabla Formation and coeval intrusive rocks. At Escondida Norte, the La Tabla Formation comprises a lower suite of biotite-hornblende bearing andesitic lavas and tuffs dated at 294.4 ±4.6 Ma (Jara et al., 2009). These are overlain to the west by a rhyolitic sequence that includes welded ignimbrite, variously dated at 290.0 ±4.0, 294.2 ±2.4, and 298.2 +5.5/-4.9 Ma (Richards et al., 1999; Jara et al., 2009). These rocks are intruded by coarse-grained monzogranite dated at 298.8 ±2.6, 293.0 ±6.0, 291.1 ±2.3, 289.9 ±3.5 Ma (U-Pb zircon; Morales, 2009), 287.1 ±4.4 Ma granodiorite porphyry (U-Pb zircon; Jara et al.; 2009) and diorite. To the southeast, Early Permian quartz-diorite and quartz-monzodiorite porphyry have been defined, dated at 293.0 ±4.3 and 287.6 ±3.3 Ma, respectively at the nearby Pampa Escondida (Fig. 9). To the west, in the Zaldívar deposit, across the west vergent Panadero reverse fault, andesitic volcanic rocks at depth have been correlated with the La Tabla Formation, but are overlain by andesites of the Augusta Victoria Formation (Navarro et al., 2009). The Pandero Fault marks the boundary at surface between the La Tabla and Augusta Victoria formations (Fig. 9).
At Escondida Norte and Zaldívar, the La Tabla Formation is intruded by a multistage igneous complex of dykes and larger lensoid stocks with a pronounced NE to NNE orientation. This complex is composed of at least three principal pulses that are related to copper mineralisation, comprising early-, inter- and late-mineral phases. The early and inter-mineral phases are coarse grained porphyries with rhyolitic to granodioritic to dioritic compositions (Urzua 2009) and have been dated at 38.0 ±0.5 and 37.5 ±0.5 Ma, whereas the late mineral phase is a dacitic porphyry which to the west at Zaldívar has yielded ages of 36.0 ±0.8, 35.7 ±0.7, and 35.5 ±0.8 Ma (U-Pb zircon; Jara et al., 2009).
Cross-cutting polymict magmatic-hydrothermal breccias are found as small, rod-like bodies associated with the early- and inter-mineral porphyries, and have undergone sericitic or chlorite-sericite alteration. They are cemented by quartz, pyrite and varied amounts of chalcopyrite at shallow depths, but by a pegmatoidal quartz-biotite-anhydrite ±K feldspar ±magnetite assemblage along with chalcopyrite and bornite at depth (e.g., Monroy, 2000; Navarro et al., 2009). The breccias typically contain higher copper grades than surrounding rocks.
Both hypogene and supergene alteration and mineralisation is exploited at Escondida Norte.
Supergene mineralisation and alteration occurs below 100 to 200, up to as much as 350 m, of hematitic leached capping, and comprises an enriched blanket that ranges from 20 to 250 m in thickness that constitutes the main orebody. This enrichment blanket covers a NE elongated area of ~2 x 1.5 km, roughly paralleling the present topography (Monroy, 2000; Williams 2003). It comprises an upper high-grade, chalcocite-dominated sheet, and a covellite-bearing lower sheet in which pyrite is only poorly replaced. The supergene mineralisation is accompanied by ubiquitous kaolinite, whilst associated supergene alunite returned ages of ~17 to 14 Ma (Morales, 2009). Sheets of copper oxides are irregularly developed above the sulphide enrichment zone. These are mainly composed of antlerite and brochantite in the central, highest grade parts of the deposit (Maturana and Saric, 1991; Monroy, 2000; Williams, 2003), although chrysocolla and atacamite occur peripherally. To the south of Escondida Norte, a 5 km long palaeochannel contains low-grade, exotic copper mineralisation, hosted by both the basal Pampa de Mulas gravels and channels in the immediately subjacent bedrock.
Hypogene mineralisation and alteration. Early alteration is represented by a potassic assemblages that are developed at depth throughout the deposit. A biotite-K feldspar assemblage dominates in felsic host rocks, whilst biotite with minor magnetite characterises andesitic volcanic rocks and diorite. Potassic alteration includes veinlets of early biotite and magnetite as well as abundant A-type K feldspar and quartz-K feldspar veinlets. The potassic phase is overprinted by grey sericite veinlets, although these do not constitute a coherent, mappable zone(s) as at Escondida Este.
The potassic zone is overlain at shallower levels by extensive developments of chlorite-sericite alteration, largely occurring as chlorite-sulphide veinlets that overprint and largely destroys the potassic assemblage. This, in turn, passes upward into a zone where sericite dominates, locally overprinted by quartz-pyrophyllite ±alunite alteration that is closely associated with NW-striking, high-sulphidation veinlet zones, some of which are sheeted.
The bulk of the hypogene sulphide mineralisation comprises chalcopyrite and pyrite, with only localised pockets of chalcopyrite-bornite ±chalcocite in the potassic zone, predominantly in the Zaldívar deposit to the west. Copper tenors tend to drop-off at depth in the potassic zone, interpreted to imply that appreciable copper was introduced during the chlorite-sericite stage. Molybdenum grades average ~100 ppm (Romero, 2008). Pyrite, enargite, tennantite and sphalerite are found in the high-sulphidation veinlet zones (Williams, 2003).
The information in this Escondida Norte summary is largely drawn from Hervé et al. (2012).
Return to Top
ZALDÍVAR DEPOSIT
The Zaldívar porphyry copper deposit is contiguous with, and immediately west of the Escondida Norte pit, with the boundary between the two being based on mining title ownership. That boundary is located just to the east of the Portezuelo Fault, which separates the Paleocene to Eocene Augusta Formation to the west, from the Early Permian La Tabla Formation to the east (Fig. 9).
As at Escondida Norte, mineralisation at Zaldívar is localised within a NE-striking corridor of multi-stage, early- and late- inter-mineral granodioritic to dacitic porphyry intrusions and associated magmatic-hydrothermal breccias. Mineralisation is largely centred on these intrusions, collectively referred to at Zaldívar as the Llamo Porphyries. In the west, these porphyries intrude andesites of the Paleocene to Eocene August Victoria Formation, dated at 66.6 and 41.2 Ma (Marinovic et al., 1995) and 57 to 53.9 (Urzúa 2009), and rhyolites of the Early Permian La Tabla Formation in the east. These intrusives also cut across the north-south striking, steep reverse Portezuelo Fault that largely separates the two host formations.
The Early Permian rhyolites, which are dated at ~290 Ma (Richards et al., 1999) are locally referred to as the 'Zaldívar porphyry', and are typically composed of grey rhyolitic feldspar-quartz porphyry. Phenocrysts are predominantly quartz, K feldspar and plagioclase. The quartzo-feldspathic groundmass is variably replaced to obliterated by the prevalent phyllic quartz–sericite alteration in the immediate deposit area. The rhyolites are accompanied by comagmatic granitoids.
The andesites of the August Victoria Formation, are greenish-grey to dark-grey and have a fine-grained porphyritic texture within an aphanitic groundmass.
The Llamo porphyry is the most recent intrusive event at Zaldívar, and is mainly of granodioritic composition, but includes dacite porphyries dated at 38.7 ±1.3 Ma (U-Pb zircon; Richards et al., 1999), 38.7 ±0.4 and 37.5 ±0.5 Ma (U-Pb zircon; Pollard and Taylor 2002), 35.5 ±0.7 to 37.4 ±0.2 Ma (40Ar/39Ar; Campos 2002). A limited exposure of post-mineral late Llamo porphyry, known as the 'Latellamo Porphyry' has been mapped straddling the Portezuelo Fault. It occurs as irregularly shaped dykes to small, elongated stock-like bodies that are 50 to 200 m wide, and typically comprises a light greyish-green, fine grained feldspar-biotite-quartz porphyry.
Numerous hydrothermal and tectonic breccias recognised in the deposit area are closely associated with major structures, such as the Portezuelo Fault and along intrusive contacts of the Llamo Porphyry.
Thick Quaternary alluvium/colluvium covers much of the lower slopes and valley floors in the deposit area. Most are locally derived and generally comprise dry, loose to dense, well-graded silt, sand and gravel. Pockets of aeolian silt and fine sand are also found in the area.
The deposit is located at the intersection between i). steeply dipping north-south to NNE-SSW striking faults of the ~10 km wide Domeyko Fault System, the principal of which locally is the Portezuelo Fault, and ii). a set of NW-SE structures (Fig. 9). Both sets appear to be pre-intrusive and are interpreted to control the emplacement of subsequent intrusions, but have also later offset them. The intersection of these faults similarly marks the best developed copper mineralisation. A secondry set of less obvious, weakly to moderately developed NE-SW trending faults with steep NW and SE dips appear to control the location and emplacement of the Llamo porphyry.
The alteration developed at Zaldívar largely comprises an early potassic event, characterised by secondary K feldspar, which overprints the Llamo Porphyry and the Palaeozoic rocks below the 3050 m elevation, accompanied by secondary biotite, that particularly affects the andesitic rocks.
This alteration is overprinted by two stages of retrograde quartz-sericite hydrothermal alteration: i). an extensive early sericite-chlorite, followed by ii). the principal phase that comprises more penetrative quartz-sericite-pyrite alteration, focused in the core of the deposit, with a close association with the Llamo Porphyry intrusions. Strong sericite alteration occurs as discontinuous bodies in the core of the quartz-sericitic alteration zone and typically destroys primary rock textures. Propylitic alteration, with chlorite as the principal mineral, overprints both the andesitic rocks and the Latellamo Porphyry.
The final hydrothermal pulse is represented by a very restricted advanced argillic suite. This was followed by supergene argillic alteration, resulting from the leaching and oxidation of the rock column of the deposit. The supergene argillic alteration affects all of the lithologies with variable intensity, but is typically stronger in the andesitic rocks.
The mineralisation at Zaldívar also follows a NE-SW trend reflecting the Llamo Porphyry intrusions and comprises overprinting hypogene and supergene processes. The latter involved leaching and enrichment, producing a characteristic vertical profile with an upper leached capping, then, underlying oxide copper, and lower secondary sulphide enrichment zones, which, in turn, grades down via a mixed intervall into the hypogene sulphides.
The leached capping occurs as an almost continuous, up to 300 m thick upper layer from the surface downward, typically represented by an assemblage that includes hydroxides and sulphates of iron (hematite-goethite-jarosite) accompanied by copper phosphate (turquoise).
The underlying copper oxide zone is distributed virtually continuously from the Portezuelo Fault in the east, extending towards the SW, over an area of ~2 x 1.5 km, with an average thickness of ~90 m. This zone is locally incised by vertical wedges of leaching centred on steep NW-SE faults. Within the andesites, whilst oxidation breaks down the hypogene sulphides, the mafic content of the host largely inhibits Cu from going into solution and results in the precipitation of Cu-oxide minerals with very little vertical transport. The mineral assemblage within the oxide zone varies according to lithology and dominant alteration, e.g., brochantite-antlerite is found in the rhyolitic rocks and chrysocolla, neotocite ('black copper wad') and copper phosphate are found in the andesitic rocks with chlorite-biotite alteration.
The secondary sulphide blanket that separates the oxide and hypogene sulphides is more extensive, covering an area of ~2.5 x 1.5 km, extending into the rhyolites to the east of the Portezuelo Fault. It has a variable thickness, ranging from a few metres or less in the southwestern extremity within the andesites, up to >300 m to the northeast, in the rhyolites. It is characterised by an assemblage of pyrite, chalcocite, covellite, chalcopyrite and minor sphalerite and molybdenite. Chalcocite is the dominant (>80%) supergene sulphide mineral, grading down into a covellite-rich layer in the transition to the underlying hypogene sulphides. Alunite dating suggests an age for the supergene enrichment process of ~18 to 14.7 Ma (Monroy 2010).
The underlying hypogene sulphide zone follows the Llamo Porphyry trend, with >70% of the mineralisation occurring in veinlets. The primary sulphide body has a bornite-rich core with minor chalcopyrite, located within the Llamo Porphyry and in late magmatic breccias. This core is enveloped by a chalcopyrite-pyrite zone that is, in turn, surrounded by a halo of pyrite. The hypogene mineralisation has been dated at 37.2 Ma (Morales 2010).
The bulk of the hypogene mineralisation was emplaced synchronously with the potassic alteration and both A- and B-type veinlets during the development and evolution of multiple centres of biotite-bearing, early-mineral porphyry and breccia bodies. Late-mineral, hornblende-bearing dacite porphyry phases and associated breccia bodies post-dated the A- and B-veinlets, but contributed additional, though lower grade, mineralisation, and pre-dated multiple generations of D-type veinlets. The latter also emplaced additional lower grade, mineralisation. Late-mineral dacite dykes are barren (Perelló et al., 2018).
The porphyry system extends both to the east and NE, connecting Zaldívar with Escondida Norte, although Perelló et al. (2018) regard these to be separate, coalescing porphyry copper deposits.
The information in this summary is drawn from Evans, L. and Lambert, P.E., 2012 - Technical report on the Zaldívar Mine, Region II, Chile; an NI 43-101 Technical Report prepared by Roscoe Postle Associates Inc., for Barrick Gold Corporation, 114p. and from Hervé et al. (2012).
Return to Top
PINTA VERDE DEPOSIT
The Pinta Verde deposit is located on the southwestern extremity of the known Escondida Norte-Zaldívar mineralised corridor and contains both hypogene sulphide and oxide resources. Mineralisation is said to be associated with an intrusive described by Urzúa (2009) as dacite porphyry, and by Hervé et al. (2012) as a porphyritic biotite tonalite. This intrusion is apparently markedly different to those related to the other deposits of the corridor, and does not appear to have yet to be dated (in 2021). It has been intruded into andesites of the Late Paleocene to Early Eocene Augusta Victoria Formation. Hydrothermal alteration include potassic, predominantly biotite, phyllic quartz-sericite, sericite-clay-chlorite intermediate argillic and propylitic assemblages (Sandoval, 2002; Urzúa, 2009). The copper oxide mineralisation is dominated by an assemblage of chrysocolla, atacamite, black oxide/Neotocite phases and copper-bearing clay developed from the hypogene low-grade chalcopyrite mineralisation.
Little information has been found on this deposit in publicly available sources. No significant mining has been undertaken, and the deposit is quoted as containing Measured + Indicated + Inferred Mineral Resources at 30 June, 2020 of 188 Mt @ 0.57% Cu as oxides and 60 Mt @ 0.47% Cu as sulphides (BHP Annual Report, 2020).
Return to Top
PAMPA ESCONDIDA DEPOSIT
Pampa Escondida is a giant porphyry copper deposit that occupies much of the interval between Escondida Norte and Escondida Este (Fig. 2). The deposit lies below a flat valley that separates the more positive topography at Escondida and Escondida Norte, and is almost completely concealed by 10 to 130 m of piedmont gravel of the Pampa de Mulas Formation, with minimal outcrop of any copper oxides. Mineralisation is associated with a deep, multiphase, Eocene porphyry stock, most of which is at depths of >600 to 700 m below the surface. This stock intrudes Early Permian volcanic rocks of the La Tabla Formation that is the host to much of the deposit (Fig. 9).
The La Tabla formation comprises 288.0 ±2.4 Ma andesitic tuffs and flows that are intruded at depth by dark quartz diorite (Pórfido Negro) and quartz monzodiorite porphyries (Pórfido Temprano) that at Pampa Escondida have been dated at 293.0 ±4.3 and 287.6 ±3.3 Ma, respectively. The upper part of this formation contains intercalated, shallowly dipping, lenses of calcareous sedimentary rock, dacitic tuff and andesitic lava (Fig. 12).
The earliest phase of the Eocene stock is a 37.6 ±0.5 Ma precursor hornblende diorite which occurs as dykes cutting the La Tabla Formation. Copper mineralisation was introduced during the emplacement of several phases of biotite granodiorite porphyry that intrude all the Early Permian rocks. The first phase of this porphyry has been dated at 36.1 ±0.7, 36.0 ±1.2, and 35.0 ±0.6 Ma, and comprises a NE-oriented, 2 x 0.5 km stock. The core of the stock is intruded at depths of >1000 m, by an inter-mineral porphyry phase dated at 35.0 ±0.8 and 34.5 ±0.4 Ma. The early and inter-mineral phases are, in turn, cut by a 20 to 30 m thick, late inter-mineral, 35.2 ±0.8 Ma hornblende dacite porphyry dyke in the northeast of the deposit. Several NW striking dykes of barren, polymict breccia as well as minor monomict magmatic-hydrothermal breccias cut the granodiorite porphyry stock in the NE of the deposit area. The polymict breccias, which are cemented by rock-flour, are considered to be phreatic in origin, and postdate all alteration and mineralisation.
Alteration zonation at Pampa Escondida occurs as dome shaped shells, draped over the early granodiorite porphyry stock (Fig. 12). The deep core of the composite Eocene stock, occupied by the inter-mineral porphyry phase, has only undergone weak K felspar alteration as a chlorite-biotite assemblage. The older surrounding early granodiorite porphyry in the mid to outer core of the deposit has been subjected to potassic alteration where K feldspar is >biotite. Locally this assemblage persists inward and down into the intermineral porphyry. Outward and upward, the Early Permian porphyries are altered to biotite>K feldspar, whilst intense biotite alteration characterises the Early Permian andesitic volcanic rocks. Calcareous lenses within the upper part of the andesitic sequence have been locally converted to garnet-diopside skarn. External to the potassic core zone, the andesitic sequence and late mineral dacite porphyry dykes are extensively altered to an chlorite-sericite assemblage. Sericitic alteration is not abundant and is largely restricted to the shallowest levels of the deposit.
Mineralisation zonation closely follows the alteration pattern, with the weakly potassic altered intermineral porphyry phase being more weakly mineralised, characterised by grades of generally <0.2% Cu as a pyrite-chalcopyrite assemblage forming a low grade core. The surrounding, more strongly potassic altered shell of K feldspar>biotite contains the highest grade mineralisation, ranging from 0.5 to 1.0% Cu, as chalcopyrite and bornite in a 2:1 ratio. These sulphides are also accompanied by lesser hypogene digenite, chalcocite, covellite and molybdenite, and occur in early K feldspar and later A- and B-type quartz veinlets, and as disseminations. Minor grey sericite veinlets are also observed. The peak molybdenite concentrations occupy an annular zone, partly overlapping and external to the copper-rich shell.
The chalcopyrite-bornite mineralisation grades outward to chalcopyrite-pyrite, averaging 0.2 to 0.5% Cu, which in turn, is transitional to a pyritic halo containing 2 to 3 vol.% pyrite and <0.1% Cu. The late mineral, chlorite altered dacite porphyry dyke is also pyritic.
The Pampa Escondida deposit contains more gold than the others in the district, with indicated resources averaging 0.1 g/t Au. The highest gold grades accompany the inner bornite rich zone.
Supergene mineralisation is only poorly developed at Pampa Escondida due to the depth of the deposit. A leached capping that averages ~40 m in thickness, locally encloses zones of oxide copper mineralisation, and is underlain by a zone of secondary sulphide enrichment that averages ~15 m in thickness. The oxide zone is characterised by an assemblage that predominantly comprises chrysocolla, malachite and brochantite, but also includes dioptase, atacamite and cuprite, which are largely confined to the calcareous horizons. Supergene montmorillonite, nontronite and vermiculite, partly copper bearing, accompany the oxide copper mineralisation.
The total published resources in 2020 amounted to 7.440 Gt @ 0.45% Cu, 0.05 g/t Au (see the Reserves and Resources section below for detail). The bulk of the higher grade mineralisation (i.e., >0.75% Cu) lies at depths of between 400 and 1200 m below the surface.
The information in this Pampa Escondida summary is largely drawn from Hervé et al. (2012 and 2010).
Return to Top
PAMELA RESOURCE
The Pamela Oxide resource is located between the Escondida Norte and Pampa Escondida deposits, partially underlying Escondida Norte waste dumps. Drilling has identified lithological and structurally controlled copper oxide mineralisation overlying portions of a deeply buried porphyry system. Deep sterilisation drilling to the east has delineated a limit in that direction, while to the west it is abutted by the Zaldívar mine. It is elongated east-west and lies within the northern 20% of the 0.3% Cu envelope that surrounds Pampa Escondida, as shown on Fig.s 2 and 9. Mineralised bedrock is completely masked by an average of 30 m of unconsolidated Late Cainozoic gravels. Host rocks comprise the Early Permian andesitic volcano-sedimentary unit, the UVS, underlain by dacitic and andesitic volcanic rocks, that together with the UVS, are part of the La Tabla Formation. These are intruded by the ~290 Ma Grueso Porphyry, Rhyolite Porphyry and Negro Porphyry (see the Pampa Escondida description above). All of these are, in turn, intruded at depth by the Pamela Porphyry, a granodiorite associated with mineralisation, and by two post-mineral dacite porphyry bodies. Mineralised late-stage hydrothermal breccias also cut the sequence.
Mineralisation comprises an upper zone of copper oxides hosted by weathered sedimentary units and dacite tuff of the UVS that are interbedded with the andesite volcanics. Limestone interbeds contain disseminated and fracture-controlled mineralisation that is dominated by chrysocolla-brochantite, with or without the accompanying formation of garnet-actinolite skarn. Oxide mineralisation in dacite tuff is predominantly fracture controlled chrysocolla. This oxide mineralisation is confined to the 100 to 200 m thick oxidised and leached cap, which is underlain by a thin erratic chalcocite-covellite enrichment zone, which grades downward as a series of structurally controlled tapering tongues into weak chalcopyrite-pyrite mineralisation at depth.
A JORC compliant 'Exploration Target' estimate suggest a potential tonnage ranging from a low of 30 Mt and high estimate of 50 Mt @ 0.5% Cu, using a 0.2% Cu cut-off (BHP Billiton, 2014).
This Pamela summary is drawn from a BHP Billiton presentation "Unlocking Shareholder Value" submitted to the ASX and NYSE, 19 August, 2014.
Return to Top
BAKER PROSPECT
The Baker Prospect is located ~7 km east of Escondida Norte. It comprises a shallow, NNE elongated, 1500 x 500 m supergene sulphide zone that overlies deeper low grade hypogene mineralisation that flairs outward with depth. Hypogene mineralisation is largely hosted by an elongate, composite, Eocene granodiorite porphyry stock, that comprises an early-mineral 37.1 ±0.7 Ma granodiorite porphyry and an inter-mineral monzodiorite. This stock was intruded into Late Paleocene to Early Oligocene Augusta Victoria Formation andesite and precursor fine-grained 38.2 ±0.5 Ma hornblende diorite and monzodiorite intrusions which occur as a series of stocks. The hypogene mineralisation is composed of veinlet and disseminated bornite-chalcopyrite occurring as a central core, passing out into lower-grade chalcopyrite-pyrite. The supergene enriched zone varies from 20 to 200 m in thickness, overprinting the hypogene sulphide zone below a 150 m thick leached cap. The secondary blanket is influenced by both NW and NE striking structures and the primary sulphide zone by NE-trends.
The deposit was estimated at 30 June 2014 to represent JORC compliant 'Exploration Targets' of 50 to 150 Mt @ 0.4% Cu (at a 0.2% Cu cut-off) of supergene mineralisation, whilst the hypogene mineralisation was estimated be within the range of 600 to 1100 Mt @ 0.3% (also at a 0.2% Cu cut-off).
This Baker summary is drawn from a BHP Billiton presentation "Unlocking Shareholder Value" submitted to the ASX and NYSE, 19 August, 2014.
Return to Top
CHIMBORAZO
Chimborazo is interpreted to be part of a broadly related, but separate and slightly older, ~41 Ma porphyry cluster, located ~10 km NW of the main Escondida mineralised system (Fig. 2). However, according to Richards et al. (2001) it falls within the same larger propylitic halo that also embraces the Escondida deposits. Chimborazo is interpreted to represent an epithermal system at shallow depths, overlying porphyry style mineralisation at a depth of ~1000 m. It is largely hosted by dacitic tuffs and lesser andesitic breccias and flows of the Paleocene to Oligocene Augusta Victoria Formation (Petersen et al., 1996). The only resource, which was delineated by 2014 and has not been mined to 2021, is within a supergene enrichment zone in the northern part of the Chimborazo system, developed within an advanced argillic lithocap (see resources and reserves section below). To the north of the exposed lithocap, the system is masked by a north-thickening wedge of Pampa de Mulas gravels (Herve, et al., 2012). Based on limited drilling, the potential for large low grade hypogene porphyry resources at depth have been investigated with JORC compliant 'Exploration Targets' as at 30 June 2014 of a low case of 1Gt @ 0.5% Cu, to a high case of 6.6 Gt @ 0.32% Cu (BHP Billiton presentation, 2014).
The uppermost sections of an early-mineral biotite granodiorite porphyry intrusion has been intersected at a depth of ~1000 m below surface, whilst ~39 to 37 Ma fine-grained, inequigranular diorite to granodiorite phases of the inter- to late-mineral Chimborazo Intrusive Complex are found to the NW. This intrusive complex is controlled and cut by NE-striking faults, some displaying ductile syn-intrusion deformation textures at depth (Herve, et al., 2012; BHP Billiton, 2014).
Phaneritic hornblende diorite and monzodiorite intrusions that appear to be both pre-mineral (~42 Ma) and late-mineral (38.1 ±0.3 Ma; Ar/Ar; Richards et al., 1999), cut the altered host volcanic succession both south and north of the alteration zone, as well as at depth. Of these, the late-mineral intrusions occur as minor bodies and dykes of predominantly hornblende diorite, which cut the lithocap immediately south of the supergene copper deposit, and appear to be closely related to the Chimborazo Intrusive Complex (Herve, et al., 2012; BHP Billiton, 2014). In the deposit area, the pre-mineral monzodiorite occurs as a gently NNW plunging spur of larger intrusion to the south. This spur is exposed immediately to the south of the argillic cap and plunges shallowly beneath the surface, with occasional sub-crops, with relatively steep flanks to the NW and SW, such that by the section shown on Fig 15, it occurs at depth (BHP Billiton presentation, 2014).
Both the monzodiorite and volcanic rocks are cut by a series of pipe-like, magmatic-hydrothermal breccias over a 1000 m vertical interval. These breccias are largely mineralised as open-space fill by a succession of mineral assemblages that vary vertically within the system-scale alteration-mineralisation pattern. At depth they are cemented by anhydrite with chalcopyrite and molybdenite, grading upwards through assemblages that include quartz, schorlitic tourmaline, sericite, pyrite and gypsum (after anhydrite), to quartz, alunite, pyrite, enargite, native sulphur, and abundant open space (produced by gypsum dissolution) in the advanced argillic lithocap. This gradation is consistent with the system-scale alteration-mineralisation zoning pattern (Herve, et al., 2012; BHP Billiton, 2014).
Hypogene mineralisation and alteration - Fine-grained quartz-alunite alteration dominates the topographically higher sections of the lithocap, below the upper slopes of Cerro Chimborazo. This suite grades downward, from near its exposed base, to quartz-alunite-kaolinite plus minor dickite. Crystalline hypogene alunite from a distal lithocap exposure, 6 km to the east, was dated at 42.4 ±2.0 Ma. A series of deep 'prongs' (Fig. 16) of the advanced argoillic lithocap extend downward, some reaching depths of 1000 m below the surface, immediately above the deep potassic porphyry-style alteration core described below. These 'prongs' grade from the shallow quartz-alunite-dickite assemblage to quartz-pyrophyllite-dickite at depth, the latter assemblage containing minor diaspore, topaz and andalusite. Green tourmaline coexists with lesser dumortierite throughout the advanced argillic prongs, the upper parts of which also contain native sulphur. Apart from these lithocap prongs, the advanced argillic alteration grades downward and outward through a sericitic zone to an earlier chlorite-sericite assemblage that contains minor schorlitic tourmaline. The chlorite-sericite alteration, in turn, grades downward and overprints a deep potassic zone, characterised by pervasive biotite within volcanic rocks and orthoclase, minor quartz and varying anhydrite in the deep granodiorite porphyry intrusion. A grey-green sericite event with associated quartz and andalusite locally overprints the potassic alteration (Herve, et al., 2012; BHP Billiton, 2014).
The rocks that have undergone potassic alteration contain minor chalcopyrite, pyrite and subordinate magnetite, whilst those overprinted grey sericite are characterised by chalcopyrite, bornite and elevated gold. Within the chlorite-sericite zone, mineralisation is dominantly pyritic with evidence of hypogene leaching of pre-existing chalcopyrite, whilst its peripheries contain a well defined zinc halo. The advanced argillic zone contains early pyrite and disseminated, veinlet and veins with enargite ±tennantite, whilst veins of late chalcopyrite-sphalerite ±pyrite ±galena tend to occur towards its peripheries. Veins have a consistent NE strike and follow minor faults and fractures (Petersen et al., 1996). The highest copper grades are developed within the grey sericite zone, whilst the porphyry-hosted potassic alteration generally only carries low-grade mineralisation (BHP Billiton, 2014). The potential size of the deep hypogene mineralisation was estimated in October 2011 to range from 1 to 6 Gt @ an average grade of 0.3 to 0.6% Cu (BHP Billiton, 2014).
Supergene mineralisation - The supergene chalcocite enrichment blanket is overlain by a hematitic leached capping that is up to 150 m thick, with only very minor brochantite-dominated oxide copper mineralisation at its base. The supergene blanket is laterally continuous over an area of ~2 x 1 km, and averages ~100 m in thickness. Grades are low, generally from 0.4 to 0.7% Cu, with chalcocite dominating at shallow levels, whilst covellite increases at depth. The distribution of grade is strongly controlled by NE-striking faults that host high-sulphidation pyrite-enargite veins, and in which enrichment extends considerably deeper (Petersen et al., 1996). Supergene alunite veins at and near Chimborazo have been dated at ~18 to 16 Ma (Herve, et al., 2012).
Return to Top
RINCONES PROSPECT
The Rincones prospect is located ~10 km west of Escondida Norte and ~6 km SSW of Chimborazo, and is considered to be part of a cluster of porphyry copper centres which include the latter. Mineralisation was discovered in 2004 through surface mapping, geochemistry and drilling, with delineation drilling continuing until 2009. Exposed host rocks include andesitic and rhyolitic volcanics of the Palaeocene to Eocene Augosto Victoria Formation that have also been intruded by composite late Eocene to Oligocene diorite to granodiorite stocks. The host units are largely covered by a veneer of Miocene to Pliocene post-mineral gravels. The main structural trends parallel the generally north-south lineaments that control the topography of the area, although NE and NW trending faults are also interpreted to be important to emplacement of the porphyry cluster. Drilling and trenching have outlined narrow and erratic intercepts of supergene oxide and chalcocite mineralisation to a depth of 300 m below surface (Fig. 17). This mineralisation is interpreted to represent a thin and discontinuous blanket overprinting structurally controlled enargite-pyrite mineralisation. On the basis of a range of assumptions on the continuity and extent of the supergene deposit, and average drill hole grades at a 0.2% cut-off, it is estimated at 30 June 2014 to represent a JORC compliant 'Exploration Target' of between 100 and 700 Mt of 0.5% Cu (BHP Billiton, 2014).
Return to Top
EXPLORATION AND DISCOVERY
The discovery drill hole that cut the Escondida deposit in March 1981 was the culmination of the 'Atacama Project', first proposed to senior management of Utah International Inc. and Getty Oil Co. by J D Lowell in July 1978 (Lowell, 1991).
The aim of the Atacama Project was to conduct a grass roots exploration program for supergene enriched porphyry copper deposits in areas of outcrop and shallow cover in the Pre-Andean Range of northern Chile. The program was localised over a 500 km interval between Calama (Chuquicamata) and Inca del Oro (just SW of El Salvador). The joint venture (JV) was negotiated and signed in January 1979 and work commenced soon after, under the management of Lowell, who reported to a management committee representing the partners (Lowell, 1991; Ortiz 1995).
The project was based on the premise that porphyry copper deposits are accompanied by predictable, concentric, zonal patterns in which the outer propylitic zone may cover an area of up to a hundred times that of the orebody it surrounds. It was also recognised that this target could be further enlarged, as many porphyry deposits are surrounded by a halo of peripheral polymetallic occurrences and deposits that form clusters (defined as 2 or more occurrences per square mile, or up to 1 per km2). This expanded the target area by a factor of several times. The concept was that, while the deposit may be concealed under cover, the peripheral alteration and polymetallic halo might be mapped, or detected in cheap, shallow, air-core scout drilling, and the predictive vectors followed to ore. In addition, the leached cap over a supergene enriched deposit would most likely be soft, recessive and concealed by younger cover. Within the project area there was, what was considered, a favourable mix of around 50% pre-mineral outcrop in which to search for halos, and 50% pre-mineral rocks sub-cropping below relatively shallow post-mineral gravels and volcanics in which a concealed deposit might lie (Lowell, 1991).
Initial work on the project included assembly of data, and both aerial and ground reconnaissance, using joint venture staff and local consulting geologists. The project proceeded with concurrent regional and local programs. The regional work comprised a geochemical survey over the entire interval targeted by the project, involving the collection of stream sediment samples (sieved to -80 mesh) at 1 km intervals along three parallel longitudinal traverses, each extending over the whole north-south length of the 30 km wide target porphyry belt. This produced some 1400 samples during 1979, each of which was assayed for Cu, Mo and Zn. Thresholds were established at 80 ppm Cu, 10 ppm Mo and 100 ppm Zn. Concurrently with the geochemical survey, a regional geological map was prepared from existing data and new reconnaissance mapping. Some 30 anomalies were delineated from the geochemistry, while other targets were identified and followed up based on known mineralisation, existing data and the previous experience of the project team (Lowell, 1991; Ortiz 1995).
The 30 regional geochemical anomalies were followed up by another 670 stream sediment and soil samples, as well as a study of float in sampled water courses. After elimination of 'cultural' anomalies (due to contamination from smelters, mine dumps, known workings, etc.), 10 anomalies remained, one of which was the Escondida district. As anomalies were identified by the regional work, they were progressively followed up by district and prospect scale investigations. To give protection to the better anomalies, ~1 million hectares of titles were pegged, one of which enclosed the Escondida deposit
(Lowell, 1991).
One of the prospects visited on the basis of previous experience of project staff was the Escondida district. A field visit in August 1979, prior to the results of the regional geochemistry, recognised the following favourable characteristics: i). the presence of a large, well-zoned porphyry copper system with an extensive phyllic zone, surrounded by strong propylitic alteration; ii). skarn mineralisation and peripheral polymetallic vein occurrences; iii). a well developed leached capping, hosted by sericite, alunite and silica altered quartz-feldspar porphyry, could be recognised on two hills, surrounded by post-mineral cover; iv). while limonite was very sparse in much of the capping, in about 20% of samples there was evidence of limonite after chalcocite. As with many of the other occurrences considered, following the field visit, a grid was established and 313 leached cap and shallow geochemical soil samples were collected on a 50 x 100 m grid. The grid covered an 1800 x 1300 m area of the leached cap, above the northwestern half of the Escondida supergene sulphide enrichment blanket. Copper values ranged from 10 to 660 ppm, 30% of which were >100 ppm and 9% >200 ppm. Molybdenum values varied from 1 to 480 ppm, with two samples yielding 3000 ppm. Of these, 40% were >20 ppm and 13% were >50 ppm Mo, the latter being regarded as highly anomalous (Lowell, 1991; Ortiz 1995). Soon after the August 1979 visit, data from the regional geochemical sampling also pointed to the Escondida district, and was followed up with a further 50 stream sediment samples over a larger area within the district. This follow-up indicated stream sediment anomalies of 10 to 22 ppm Mo in 7 samples over an area of 20 km2, 80 to 585 ppm Cu in 19 samples over an area of 45 km2, and 100 to 325 ppm Zn in 21 samples forming a halo around
the Cu and Mo anomalous zones. These samples confirmed the significance of the district, which would have attracted attention equally on the basis of either the regional geochemistry or from the field inspection based on previous knowledge (Lowell, 1991).
The area defined by the Cu, Mo and Zn stream sediment geochemical anomalism at Escondida appears to broadly coincide with the outline of the propylitic alteration zone shown on Fig. 2. A halo of Zn persisted to the outer margin of that alteration, surrounding the less extensive copper anomaly that took in the Escondida and southern Zaldívar deposits, while a molybdenum high was centred on Escondida (Lowell, 1991; Ortiz 1995). For plots of the results, see either of these two references.
The first drilling on the project was commenced in September 1979, nine months after the start of the project, to test for the primary source of an exotic copper target, elsewhere in the project area, not at Escondida. In fact some 16 200 m of drilling had been completed on 4 different targets in the first two years of the Atacama Project, prior to the discovery of Escondida (Ortiz 1995).
Following the field visits and follow-up sampling in late 1979, leached cap samples were studied by an expert consultant and a geological map was prepared over the Escondida-Zaldívar district during 1980. The leached capping study did not appreciate the possibility of superleaching (see the 'Leached Cap' section above), and concluded that the samples studied contained insufficient of the limonite characteristic of cappings associated with underlying blankets of high grade supergene chalcocite to be of significance. The study also concluded that the existence of broad areas of pervasive silicification, scarcity of quartz veinlets and the presence of alunite suggested the area was probably in the roof zone above a porphyry Cu- Mo deposit, with higher grade most likely at a depth of around 1 km (Lowell, 1991; Ortiz 1995).
The district scale mapping established the essential geological and structural framework of the district, although it overestimated the extent of the Tertiary intrusives, including some in the Zaldívar area now known to be highly altered Palaeozoic rhyolitic volcanics. The distribution of silicate alteration was also delineated and shown to cover an area of 80 km2, comprising an outer 2 to 3 km wide propylitic halo, which at Escondida, surrounded a 9 x 5 km nucleus of phyllic, phyllic-argillic and siliceous alteration, with a marginal block of biotite altered andesite on the northwestern edge. To the north, at Zaldívar, the alteration was less intense. The mapping suggested the centre of the mineralised system lay under the alluvial cover between Escondida and Zaldívar, bounded by zones of phyllic alteration found at each of these two centres. The mapping also showed that, with the exception of some fracture coatings of exotic copper minerals (chrysocolla) in a fresh diorite dyke, virtually all outcrop at Escondida was devoid of copper mineralisation. Copper sulphates were found at 200 to 300 mm depths in some isolated pits, and turquoise was occasionally noted in the southwestern part of the area. In addition, apart from a few shallow pits related to supergene aluminium sulphate mining, no historical workings were recorded at the time of discovery (Lowell, 1991; Ortiz 1995).
By early 1981, Escondida was under secure title and was scheduled for drill testing, although it was not the top priority prospect at that stage on the Atacama Project. Two targets areas within the district were selected for drilling. The first was the gravel covered interval between the phyllic zones at Escondida and at Zaldívar, with 5 drill holes at 1 km spacings planned on two lines. The second was the area of geochemical anomalism and scattered occurrences of good leached capping at Cerro Colorado, located over the northwestern section of the Escondida Stock and supergene sulphide enrichment blanket as now known. Four holes at 500 m spacings were planned in this area, from the NE to SW, to test for a supergene sulphide enrichment blanket. While the 1980 expert study of the leached capping advised against the probability of such a blanket, the project team’s emerging understanding of superleaching persuaded them to still test the possibility (Lowell, 1991; Ortiz 1995).
Air core drilling of the first target was commenced in March 1981, with 5 holes to depths of 150 to 180 m which intersected andesites and porphyries with only weak phyllic and propylitic alteration, several percent pyrite and, in two holes, 0.2 and 0.25% Cu in the primary zone. The four holes into the second target, over Escondida were drilled to depths of 300 to 450 m for a total of 1376 m, from March 13 to 21, 1981. The first, RDH-6, on the northern slopes of the Cerro Colorado, intersected 241 m of strongly leached capping before passing through 51 m of supergene chalcocite averaging 1.51% Cu, underlain by an additional 0.68% Cu enrichment zone and into a high pyrite:chalcopyrite primary zone. The second hole, RDH-7, on the southern slopes of the hill passed through 137 m of leached cap, and then into 73 m of secondary chalcocite averaging 0.68% Cu, 55 m at 1.52% Cu and 37 m of 0.68% Cu. Hole RDH-8 was further south again and over the best of the surface leached capping indications. It detected a 22 m thick interval of copper oxides assaying 0.8% Cu and an underlying 25 m of 0.67% supergene copper sulphide. The final hole in this program, between RDH-6 and 7, and a little further east, penetrated 365 m of intensely leached capping, before intersecting 91 m of supergene sulphides averaging 1.30% Cu (Lowell, 1991; Ortiz 1995).
Drilling continued, extending the limits of the supergene blanket, culminating in hole RDH-61 encountering 250 m of 3% Cu in late 1981. Some 86 drill holes and 117 480 m of drilling was required for deposit delineation, and ore reserves estimation was completed at the end of 1983 with a figure of 1.7 Gt @ 1.59% Cu using a 0.7% Cu cut-off. In 1984, Utah International was purchased by BHP Minerals and Getty Oil by Texaco, which led a year later to reassignment of Texaco’s share to BHP Minerals who onsold a 30% interest to Rio Tinto Zinc and 10% to the Japanese JECO consortium, and the formation of Minera Escondida Limitada. By end 1984, an initial feasibility study was completed. The development of the mine was announced in July 1988 and construction commenced soon after. The first production from the concentrator was in December 1990.
In 1995, Minera Escondida resumed exploration at Escondida Norte and put a large open-pit mine to exploit the deposit into production 10 years later.
Earlier, the Utah-Getty JV had optioned claims covering the prominently altered Cerro Zaldívar from a local company in early 1981 and undertook geochemical rock-chip sampling outlining an extensive molybdenum anomaly (Ortíz et al., 1985, 1986). Subsequent surface exploration and drilling between 1981 to 1984 discovered and partially outlined the Escondida Norte-Zaldívar supergene blanket, although the deposit was given a lower priority than Escondida. Sociedad Minera La Cascada, a Chilean company, purchased the Zaldívar claims in early 1989, leaving only the Escondida Norte section of the deposit under Minera Escondida control. Sociedad Minera La Cascada undertook a percussion drill program which indicated the existence of a viable orebody. However, that company became insolvent and, in late 1989, creditor banks sold the property at public auction to Outokumpu Resources (Services) Limited (Sillitoe, 1995), who onsold a 50% interest in the deposit to Placer Dome Inc. in late 1992. The resulting JV brought the mine into production in 1995. In 1999, Placer Dome acquired Outokumpu’s share, and in 2006 was merged with Barrick Gold Corporation, who has remained the owner and operator until they sold a 50% interest to Antofagasta Minerals plc in 2015.
Cerro Chimborazo, 10 km to the NW, has a prominent exposed alteration zone and was mined historically for both copper and gold. It was not formally explored until 1968 when ITT Corporation focused on epithermal-style gold mineralisation outcropping near the crest of the hill (Petersen et al., 1996). The property was optioned by Chevron and then Freeport during the 1980s, with the latter, during its gold exploration program, intersecting chalcocite enrichment grading 2.2% Cu over 22 m beneath intermontane gravel on the northern flank of the hill (Petersen et al., 1996). In 1988, Minera Orion Chile, a JV between Echo Bay Mines and Coeur d'Alene Mines, optioned the property and confirmed the existence of the Freeport intersection. Minera Orion Chile was purchased by Cyprus Minerals in late 1989, who then delineated the chalcocite enriched deposit (Petersen et al., 1996). Minera Escondida acquired the Chimborazo property from Cyprus in 2000 and began a systematic development program in 2007, with the first announced Mineral Resources in December 2011. While Chimborazo mineralisation is largely a supergene chalcocite blanket, it was recognised that the deposit has several characteristics of an epithermal deposit, rather than those of a typical porphyry copper, triggering a drilling program to determine potential for deep porphyry mineralisation below or lateral to the near-surface manifestations.
A continuing district-wide brownfields program conducted by Minera Escondida Limitada resulted to discovery of the concealed Pampa Escondida deposit in late 2006 (Sillitoe, 2010) and the deep, hypogene Escondida Este northern and the southern mineralised centres beneath the eastern edge of the Escondida open pit in early 2009 and late 2010 respectively. All three discoveries were the result of relogging core from previously drilled holes that had intersected anomalously high copper values over their final intervals, leading to geologic reappraisals and the decision to drill deeper. Padilla-Garza et al. (2004) predicted that the existence of relatively younger shallow high-sulphidation mineralisation within the ~34 Ma Cerro Sureste rhyolite porphyry might reflect a deep porphyry copper centre to the east of the Panadero Fault. Deep drilling in that vicinity, as part of a district-wide brownfields exploration program conducted by Minera Escondida Limitada, subsequently encountered a new mineralised biotite granodiorite porphyry centre in 2009, at a depth of ~800 m beneath the rhyolite porphyry (Pizarro et al., 2015). This was the northern of the two centres of the deposit.
Return to Top
RESERVES AND RESOURCES
The total reserve + resource at Escondida and its contiguous mineralised systems amounted to approximately 2.62 Gt @ 1.15% Cu in 2000.
The Escondida deposit had Ore Reserves (Proved + Probable) + Mineral Resources (Measured + Indicated + Inferred) at the end of 2002 as follows (Rio Tinto 2002 Annual Report) - Note: reserves are additional to resources:
Sulphide ore - Reserve of 1548 Mt @ 1.21% Cu, + Resource of 579 Mt @ 0.9% Cu,
Low grade float ore - Reserve of 575 Mt @ 0.60% Cu, + Resource of 815 Mt @ 0.6% Cu,
Low grade leach ore - Reserve none, + Resource of 666 Mt @ 0.4% Cu,
Oxide ore - Reserve of 198 Mt @ 0.70% Cu, + Resource of 55 Mt @ 0.5% Cu,
Mixed ore - Reserve of 49.7 Mt @ 1.03% Cu, + Resource of 62 Mt @ 0.6% Cu,
TOTAL Reserve + Resource - 4.5477 Gt @ 0.82% Cu.
In addition, Escondida Norte had Mineral Resources (Measured + Indicated + Inferred) as follows (Rio Tinto 2002):
Sulphide ore - resource of 649 Mt @ 1.3% Cu,
Low grade float ore - Resource of 642 Mt @ 0.6% Cu,
Oxide ore - Resource of 142 Mt @ 0.8% Cu,
Mixed ore - Resource of 43 Mt @ 0.8% Cu,
TOTAL Resource - 1.476 Gt @ 0.93% Cu.
The Escondida and Escondida Norte mines were operated by Minera Escondida, the principal owners of which in 2003 were BHP Billiton 57.5% and Rio Tinto 30%.
The Zaldíva deposit was 100% owned and operated by Placer Dome, which in their Annual Report (2004) was quoted as involving the following:
Ore Reserves - Proved + Probable - 421.273 Mt @ 0.668% Cu; (additional to Mineral Resources)
Mineral Resources - Measured + Indicated - 61.551 Mt @ 0.545% Cu;
Mineral Resources - Inferred - 35.139 Mt @ 0.499% Cu;
TOTAL reserves + resources - 517.963 Mt @ 0.642% Cu.
JORC compliant Ore Reserves and Mineral Resources in the Minera Escondida Operation deposits at 31 December, 2011 (Rio Tinto, 2012) were:
Escondida sulphide ore - Proven + Probable Ore Reserves - 1.993 Gt @ 0.97% Cu,
Measured + Indicated + Inferred Mineral Resources - 5.762 Gt @ 0.53% Cu,
Escondida sulphide leach ore - Proven + probable ore reserves - 3.503 Gt @ 0.50% Cu,
Measured + Indicated + Inferred Mineral Resources - 75 Mt @ 0.53% Cu,
Escondida oxide ore - Proven + probable ore reserves - 0.111 Gt @ 0.86% Cu,
Measured + Indicated + Inferred Mineral Resources - 33 Mt @ 0.65% Cu,
Pampa Escondida sulphide ore - Measured + Indicated + Inferred Mineral Resources - 7.378 Gt @ 0.47% Cu,
Pinta Verde sulphide ore - Measured + Indicated + Inferred Mineral Resources - 45 Mt @ 0.51% Cu,
Pinta Verde oxide ore - Measured + Indicated + Inferred Mineral Resources - 130 Mt @ 0.70% Cu.
Note: Reserves are additional to resources and 'Escondida' = Escondida and Escondida Norte combined.
As of ~June 2012, resources quoted for the individual deposits compiled by Hervé et al. (2012), mainly from BHP Billiton and Barrick annual reports or stock exchange releases, were:
Escondida, Escondida Este and Escondida Norte combined:
Oxide ore: 213 Mt @ 0.63% Cu,
Mixed Oxide and Sulphide ore: 183 Mt @ 0.81% Cu,
Hypogene + Supergene (leach) Sulphide ore: 13.540 Gt @ 0.57% Cu,
Pampa Escondida:
Sulphide ore: 7.378 Gt @ 0.47% Cu,
Pinta Verde:
Oxide ore: 103 Mt @ 0.63% Cu,
Sulphide ore: 72 Mt @ 0.46% Cu,
Zaldiva:
Oxide + Supergene Sulphide ore: 829 Mt @ 0.53% Cu,
Hypogene Sulphide ore: ~1 Gt @ 0.27% Cu,
Chimborazo
Supergene Sulphide ore: 223 Mt @ 0.54% Cu.
These resources contain a total 123.89 Mt of copper metal. The same deposits had produced 19.74 Mt of Cu metal prior to 2012.
At 31 December 2020, the published Ore Reserves and Mineral Resources (Antofagasta Minerals, 2020) at the Zaldíva Operation amounted to:
Oxides + Supergene Sulphides,
Measured + Indicated Resource - 797.7 Mt @ 0.39% Cu,
Inferred Resource - 33.8 Mt @ 0.43% Cu,
Hypogene Sulphides
Measured + Indicated Resource - 409.9 Mt @ 0.40% Cu,
Inferred Resource - 27.4 Mt @ 0.36% Cu
TOTAL Measured + Indicated Resource - 1207.6 Mt @ 0.40% Cu,
TOTAL Inferred Resource - 61.2 Mt @ 0.40% Cu
Combined Ore Reserves included in Mineral Resources
TOTAL Proved + Probable Reserve - 467.5 Mt @ 0.45% Cu,
Ore Reserve cut-offs are 0.33% Cu for Heap Leach ore; 0.20% Cu for Dump Leach; Mineral Resources cut-offs are 0.16% Cu for Heap Leach and 0.10% Cu for Dump Leach. In 2021, the Zaldiva mine was operated by Compañía Minera Zaldívar SpA, a 50-50 joint venture between Barrick Gold Corporation and Antofagasta Minerals).
At 30 June 2020, the published Mineral Resources and Ore Reserves (BHP Annual Report, 2020) at the Minera Escondida Operation amounted to:
Escondida, Escondida Este and Escondida Norte combined
Mineral Resources
Sulphide ore:
Measured Resource - 5.280 Gt @ 0.61% Cu,
Indicated Resource - 3.510 Gt @ 0.50% Cu,
Inferred Resource - 10.200 Gt @ 0.51% Cu,
Total Sulphide Resource - 19.000 Gt @ 0.55% Cu.
Oxide ore:
Measured Resource - 113 Mt @ 0.65% Cu,
Indicated Resource - 42 Mt @ 0.58% Cu,
Inferred Resource - 5 Mt @ 0.59% Cu,
Total Oxide Resource - 160 Mt @ 0.63% Cu.
Mixed ore:
Measured Resource - 98 Mt @ 0.58% Cu,
Indicated Resource - 63 Mt @ 0.47% Cu,
Inferred Resource - 25 Mt @ 0.44% Cu,
Total Mixed Resource - 186 Mt @ 0.52% Cu.
TOTAL Mineral Resource - 22.46 Gt @ 0.55% Cu.
Proved + Probable Ore Reserves (included within Mineral Resources)
Sulphide ore - 5.210 Gt @ 0.66% Cu,
Oxide ore - 0.206 Gt @ 0.58% Cu,
Sulphide Leach ore - 1.66 Gt @ 0.42% Cu,
TOTAL Ore Reserve - 7.076 Gt @ 0.60% Cu.
Cut-offs were: Oxide Ore - 0.20% Cu as sulphide; Mixed Ore - 0.30% Cu Total; Sulphide Ore - 0.25% or 0.30%Cu Total, depending on metallurgical process.
Metallurgical Recoveries in 2020 were - Oxide: 59%; Sulphide: 83%; Sulphide Leach: 42%.
Pampa Escondida
Measured Resource - 0.294 Gt @ 0.53% Cu, 0.07 g/t Au,
Indicated Resource - 1.150 Gt @ 0.55% Cu, 0.10 g/t Au,
Inferred Resource - 6.000 Gt @ 0.43% Cu, 0.004 g/t Au,
TOTAL Resource - 7.440 Gt @ 0.45% Cu, 0.05 g/t Au.
Pinta Verde
Oxide ore:
Measured Resource - 109 Mt @ 0.60% Cu,
Indicated Resource - 64 Mt @ 0.53% Cu,
Inferred Resource - 15 Mt @ 0.54% Cu,
Total Oxide Resource - 188 Mt @ 0.57% Cu.
Sulphide ore:
Indicated Resource - 23 Mt @ 0.50% Cu,
Inferred Resource - 37 Mt @ 0.45% Cu,
Total Mixed Resource - 60 Mt @ 0.47% Cu.
TOTAL Resource - 248 Mt @ 0.54% Cu.
Chimborazo
Supergene sulphide ore:
Indicated Resource - 139 Mt @ 0.50% Cu,
Inferred Resource - 84 Mt @ 0.60% Cu,
Total Mineral Resource - 233 Mt @ 0.54% Cu.
The most recent source geological information used to prepare this decription was dated: 2018.
Record last updated: 12/9/2021
This description is a summary from published sources, the chief of which are listed below. © Copyright Porter GeoConsultancy Pty Ltd. Unauthorised copying, reproduction, storage or dissemination prohibited.
Escondida Zaldivar
|
|
|
Alpers, C.N., Brimhall, G.H., 1989 - Paleohydrologic evolution and geochemical dynamics of cumulative supergene metal enrichment at La Escondida, Atacama Desert, northern Chile: in Econ. Geol. v.84, pp. 229-255.
|
Campos E, Wijbrans J and Andriessen P A M, 2009 - New thermochronologic constraints on the evolution of the Zaldivar porphyry copper deposit, Northern Chile: in Mineralium Deposita v.44 pp. 329-342
|
Hao, H., Campbell, I.H., Richards, J.P., Nakamura, E. and Sakaguchi, C., 2019 - Platinum-Group Element Geochemistry of the Escondida Igneous Suites, Northern Chile: Implications for Ore Formation: in J. of Petrology v.60, pp. 487-514.
|
Herve, M., 2010 - Discovery and Geology of the Pampa Escondida Porphyry, Escondida, Chile: in SIMEXMIN 2010, May 23rd to 26th, Ouro Preto, Minas Gerais - Brazil, ADIMB, Conference Presentations, 26p.
|
Herve, M., Sillitoe, R.H., Wong, C., Fernandez, P., Crignola, F., Ipinza, Km and Urzua, F., 2012 - Geologic Overview of the Escondida Porphyry Copper District, Northern Chile: in Society of Economic Geologists, Special Publication 16, pp. 55-78.
|
Jensen, K.R., Campos, E., Wilkinson, J.J., Wilkinson, C.C., Kearsley, A., Miranda-Diaz, G. and Veliz, W., 2022 - Hydrothermal fluid evolution in the Escondida porphyry copper deposit, northern Chile: evidence from SEM-CL imaging of quartz veins and LA-ICPMS of fluid inclusions: in Mineralium Deposita v.57, pp. 279-300.
|
Lowell J D 1991 - Discovery of the La Escondida orebody: in Econ. Geol. Mono 8 pp 300-313
|
Mpodozis, C. and Cornejo, P., 2012 - Cenozoic Tectonics and Porphyry Copper Systems of the Chilean Andes: in Hedenquist J W, Harris M and Camus F, 2012 Geology and Genesis of Major Copper Deposits and Districts of the World - A tribute to Richard H Sillitoe, Society of Economic Geologists, Denver, Special Publication 16, pp. 329-360
|
Ojeda-F J M 1990 - Geology of the Escondida porphyry copper deposit, II Region, Chile: in Pacific Rim 90 Congress AusIMM, Melbourne pp 473-483
|
Ortiz F J 1995 - Discovery of the Escondida porphyry copper deposit in the Antofagasta Region, Northern Chile, March 1981: in Pierce F W, Bolm J G (Eds), 1995 Porphyry Copper Deposits of the American Cordillera Arizona Geol. Soc. Digest 20 pp 613-624
|
Padilla Garza, R.A., 2003 - Description and Evolution of the Escondida Porphyry Copper Deposit, Antofagasta Region, Northern Chile: in A dissertation submitted to the Faculty of the Department of Geosciences, In partial fulfillment of the requirements for the degree of Doctor of Philosophy in the Graduate College of the University of Arizona, PhD Thesis, 203p.
|
Padilla Garza, R.A., Titley, S.R. and Eastoe, C.J., 2004 - Hypogene evolution of the Escondida porphyry copper deposit, Chile: in Sillitoe R H, Perello J and Vidal C E 2004 Andean Metallogeny: New Discoveries, Concepts and Updates SEG Special Publication 11 pp. 141-165.
|
Padilla Garza, R.A., Titley, S.R. and Pimental, F., 2001 - Geology of the Escondida porphyry copper deposit, Antofagasta Region, Chile: in Econ. Geol. v.96, pp. 307-324.
|
Pizarro, A., Ipinza, M. and Veliz, W., 2015 - Geology and Discovery of Escondida Este Porphyry Cu-Mo Deposit, Escondida Cluster District, Northern Chile: in Geologia Economica y Recursos Naturales, Exploracion Andina, Neuvos Hallazgos y Actializaciones, XIV Congreso Geologica Chilena, Las Serena, October, 2015, Proceedings v.2, pp. 382-384.
|
Porter T M, 2005 - The Escondida Porphyry Copper Deposit, Northern Chile: Discovery, Setting, Geology, Hypogene Mineralisation and Supergene Ore - A Review: in Porter, T.M. (Ed), 2005 Super Porphyry Copper & Gold Deposits - A Global Perspective, PGC Publishing, Adelaide, v.1 pp. 133-149
|
Richards J P, Boyce A J, Pringle M S 2001 - Geologic evolution of the Escondida Area, Northern Chile: A model for spatial and temporal localization of Porphyry Cu Mineralization: in Econ. Geol. v96 pp 271-305
|
Richards J P, Noble S R, Pringle M S 1999 - A revised late Eocene age for Porphyry Cu magmatism in the Escondida area, northern Chile: in Econ. Geol. v94 pp 1231-1248
|
Riveros, K., Veloso, E., Campos, E., Menzies, A. and Veliz, W., 2014 - Magnetic properties related to hydrothermal alteration processes at the Escondida porphyry copper deposit, northern Chile: in Mineralium Deposita v.49, pp. 693-707. doi 10.1007/s00126-014-0514-7.
|
Romero, B., Kojima, S., Wong, C., Barra, F., Veliz, W. and Ruiz, J., 2010 - Molybdenite Mineralization and Re-Os Geochronology of the Escondida and Escondida Norte Porphyry Deposits, Northern Chile: in Resource Geology v.61, No. 1, pp. 91-100. doi: 10.1111/j.1751-3928.2010.00150.x.
|
Sillitoe R H, McKee E H 1996 - Age of supergene oxidation and enrichment in the Chilean Porphyry Copper Province: in Econ. Geol. v91 pp 164-179
|
Porter GeoConsultancy Pty Ltd (PorterGeo) provides access to this database at no charge. It is largely based on scientific papers and reports in the public domain, and was current when the sources consulted were published. While PorterGeo endeavour to ensure the information was accurate at the time of compilation and subsequent updating, PorterGeo, its employees and servants: i). do not warrant, or make any representation regarding the use, or results of the use of the information contained herein as to its correctness, accuracy, currency, or otherwise; and ii). expressly disclaim all liability or responsibility to any person using the information or conclusions contained herein.
|
Top | Search Again | PGC Home | Terms & Conditions
|
|