Skellefte District - Boliden, Bjorkdal, Langsele, Langdal, Akulla, Kankberg, Renstrom, Petiknas, Asen, Kedtrask, Udden, Svansele, Norrliden, Holmtjarn, Maulirden, Mentrask, Rakkejaur, Nasliden, Storliden, Rudtjebacken, Kristineberg, Ravliden |
|
Vasterbotten, Sweden |
Main commodities:
Zn Cu Au Pb Ag
|
|
 |
|
 |
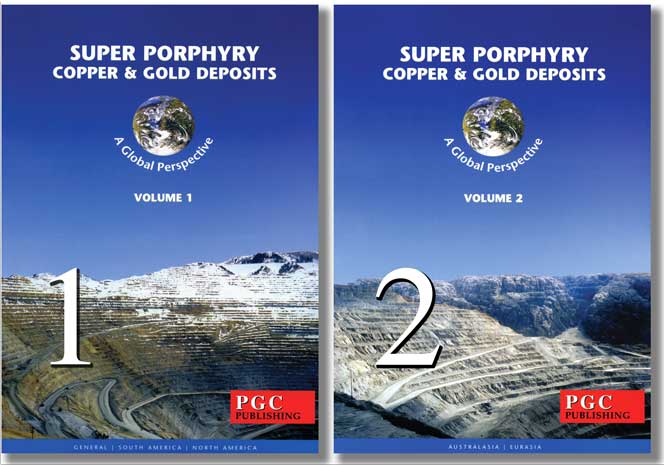 |
Super Porphyry Cu and Au

|
IOCG Deposits - 70 papers
|
All papers now Open Access.
Available as Full Text for direct download or on request. |
|
 |
The Skellefteå/Boliden/Kristineberg mining district contains >85 known pyritic volcanic hosted massive to semi-massive to vein sulphide zinc, lead, copper, silver, gold deposits and prospects located in Västerbotten Iän (County) of northern Sweden. These deposits describe an ~WNW trending, ~120 x 30 km arc that follows the Skellefte river from Boliden which is ~120 km SW of the city of Luleå. The main deposits include, from east to west: Björkdal, Boliden, Långsele, Långdal, Åkulla, Kankberg, Renström, Petiknäs South, Petiknäs North, Åsen, Kedträsk, Udden, Svansele, Norrliden, Holmtjärn, Maurliden, Mensträsk, Rakkejaur, Näsliden, Storliden, Adak Fältet (Rudtjebäcken, Lindskold, Brännmyran, Adak), Kristenberg, Rävliden North, Rävlidmyran, Horntrask, Kimheden and Åkerberg.
The deposits of the Skellefteå District are mostly hosted by the predominantly volcanic sequence of the Palaeoproterozoic Skellefte Group, and have generally been classed as volcanic hosted massive sulphide (VHMS) mineralisation. Almost all are hosted by volcanic and volcaniclastic rocks, although a few (e.g., Björkdal) are found within equivalent intrusive rocks. However, in more detail, the style of mineralisation varies considerably from stratabound at a volcanic-sedimentary boundary (e.g., Rävliden) to post-depositional, transgressive sub-seafloor mineralisation (e.g., Renström), including replacement of sedimentary rocks by massive sulphides (e.g., Näsliden), to large gash veins in shear zones (e.g., Svansele), to gold-copper stringer zones (e.g., the Fingal Zone at Renström), to vein systems in intrusives (e.g., Björkdal), to those that at least in part, have the characteristics of mineralisation found in epithermal environments (e.g., Boliden).
This record begins with an outline of the history of discovery of the key deposits in the field, then the regional setting and geology of the Skellefteå Field, an image of the geology and deposit locations, followed by descriptions of a representative selection of the deposits, as linked from the list above, including those from the different mineralisation styles. Production and reserve/resource statistics for all of the deposits listed above are included at the end of the record.
Discovery and History
The first significant mineralisation in the Skellefteå field was intersected in a drill hole at Fögelmyran, ~30 km NW of the town of Skellefteå, in December 1924. Further drilling outlined a deposit, and in the northern spring of 1926 the first ore was extracted from what would become the Boliden mine. The Rönnskär smelter was built 45 km to the SE on the coast of the Gulf of Bothnia (15 km SE of Skellefteå) to process the Boliden ore, and commenced smelting operations in 1930. Boliden and the smelter were connected in 1943 by an aerial ropeway to transport concentrates. This was later extended to be 96 km in total length to also service the Kristineberg deposits. By 1987 when it was closed this ropeway had transported 12 Mt of concentrate. Operations at the actual Boliden mine continued until 1967, although exploration outlined other deposits, with ore treated at Boliden and smelted at Rönnskär.
The total production from the Boliden deposit was 8.4 Mt @ 15.5 g/t Au, 50 g/t Ag, 1.43% Cu, 0.9% Zn, 0.27% Pb, 6.8% As, 25% S. Other trace metals included Sb, Co, Se, Te, W, Mo and Bi. During the life of the mine, ~128 t of Au, 0.118 Mt of Cu and 0.566 Mt of As were extracted (Grip and Witstam, 1970).
The first glacial boulders with dissemination sulphides were found in the Renström area in 1921, with electromagnetic ground surveys and tracing of glacial boulders back to their source during 1926. Anomalies were then tested by diamond drilling which located the Renström East orebody. Continued geophysical surveys (Turam EM) indicated the Renström A-B lenses ~500 m to the west in 1928, with subsequent diamond drilling then confirming the discovery. A shaft was sunk in 1944-48 before full production in 1953. The shaft was deepened in 1959 to the 910 m level. Simon, ~350 m to the SE of Renström, was discovered in 1998 and was in production in 2000. Two sulphide lenses were encountered in 2005 in the deep ore zone (Vilma and Julia) with ore production at Vilma commencing in 2006. Other satellite deposits were discovered including Aina, Fingal and Dagbrott. By 2019, 14.014 Mt of ore @ 2.7 g/t Au, 143 g/t Ag, 0.7 % Cu, 6.1 % Zn, 1.3 % Pb had been concentrated. Production in 2019 amounted to 0.447 Mt of ore in workings to a depth of 1500 m below surface.
Mineralised boulders found in the vicinity of Kristineberg prior to 1918 led to the commencement of exploration activities in that year and the detection of the mineralised body. A test shaft was sunk to 170 m in 1935, and 40 000 t of mineralised material extracted in 1940. The ropeway was extended to Kristineberg in 1943 to transport ore to the mill at Boliden. Mining has continued for at least 79 years to 2019, with a series of satellite lenses being outlined that extended the known resources. In that time 32.1 Mt of ore with average grades of 1.2 g/t Au, 37.7g/t Ag, 1% Cu, 3.7% Zn has been produced.
The Kankberg underground mine is located below a string of three historic open pits, the Åkulla Östra, Åkulla Västra and Kankberg gruvan (now called 'The Old Kankberg Mine'). The first two of thee open pits were mined between 1947 to 1956 and 1997 to 1998 respectively, while the third was exploited in two campaigns from 1966 to 1969 and 1988 to 1998, and now is the site of the portal to the (New) Kankberg Mine. Exploration had started in the Åkulla area in 1927, with geophysical surveys, followed up with drilling intersecting the Åkulla Östra Cu-Au-Zn mineralisation by 1933 and underground exploration carried out. Åkulla Östra consisted of several small sulphide lenses, whilst Åkulla Västra comprised Cu-Au mineralisation associated with quartz-filled fractures which was first discovered at a depth of 130 m. Sulphide-hosted copper, gold, silver and a little zinc were mined from these three open pits deposits which respectively produced 0.967, 0.197 and 1.17 Mt of ore. Exploration had continued for 'deep-seated sulphide ore' in the area throughout this period, and in 1995, Au mineralisation was encountered in a zone of strong Si-Al alteration at a depth of 350 m. This mineralised zone had mineable thicknesses with high Au grades, and is characteristic of the present Kankberg orebody. Further drilling and testing eventually led to the delineation of the deposit and commencement of production in early 2012. Production from 2012 to 2019 amounted to 2.9 Mt @ 3.5 g/t Au, 11
g/t Ag, 163 g/t Te (Voigt et al., Boliden, 2019).
The Maurliden open pit mine was closed as planned in 2019. The deposit was first indicated as a geophysical anomaly in the mid-1940s by the Swedish Geological Survey (SGU), but was not followed up by a diamond drill hole until the end of the 1950s. Further exploration by Boliden Mineral AB from 1998 resulted in a resource estimation being completed in 1999, with mining at the initial pit commencing in 2000. Production to the end of 2018 amounted to 3.8 Mt @ 1.4 g/t Au, 51 g/t Ag, 0.3 % Cu, 4.0 % Zn, 0.4 % Pb with a remaining proved reserve of 0.073 Mt @ 1.45 g/t Au, 56 g/t Ag, 0.56 % Cu, 4.40 % Zn, 0.37 % Pb (Albrecht, Boliden, 2018).
Regional Setting
The Skellefte District lies close to the northern margin of the Bothnia–Skellefteå Terrane, the largest of the 6 such terranes* that make up the 2.0 to 1.8 Ga Svecokarelian (or Svecofennian) Orogen in Sweden, that is, in turn, part of the larger Fennoscandia (or Baltic) Shield. It is separated from the Norrbotten Terrane, ~80 to 120 km to the NE of the Skellefteå line of deposits, by the Luleå-Jokkmokk Zone (Öhlander, 1993; Bergman and Weihed, 2020). This zone is interpreted to mark an unexposed, south dipping, north vergent thrust complex, which seismic data suggest dips at ~45°SW (Juhlin et al., 2002). Exposed ductile shears close to the zone dip SW with SW plunging mineral lineations, but have both normal and reverse displacements indicated. The Luleå-Jokkmokk Zone is interpreted to separate Svecokarelian rocks overlying Archaean basement to the NE from those deposited on juvenile Palaeoproterozoic rocks to the SW. This is based on 1.9 to 1.8 Ga Haparanda and Lina suite granites yielding εNd values of <-3 to the NE and >-3 to the SW. Apart from a change in character evident in aeromagnetic data (but not in gravity), this boundary is defined solely by geochemical data from younger rocks and does not mark a major change in the Svecokarelian succession, although strong deformation is noted in the Jokkmokk area, which took place after 1.87, but prior to 1.8 Ga (Mellqvist et al., 1999). At the opposite extremity of the terrane, the southwestern margin between the Bothnia–Skellefteå and Ljusdal terranes is defined by the major NW-SE trending Hassela Shear Zone some 350 km to the SW of Boliden. In contrast to the northern margin, this shear zone marks a major structural break and lithological change.
The Bothnia–Skellefteå Terrane is unconformably overlain to the west, mainly in Norway, by Late Neoproterozoic to Cambrian siliciclastic sedimentary rocks, and to the east, beneath the Gulf of Bothnia, by Mesoproterozoic to Early Palaeozoic sedimentary rocks. However, onshore in Sweden, the entire Bothnia–Skellefteå Terrane is composed of >1.96 to 1.76 Ga syn-orogenic sedimentary and magmatic rocks, although isotope geochemical data in Mesoproterozoic intrusive rocks in the southern part of the unit show contamination from an Archaean source, suggesting older basement rocks may be present at depth in that area (Andersson et al/, 2011). The exposed sequence of the terrane is dominantly composed of >1.96 to 1.76 Ga, medium to high grade metamorphosed marine siliciclastic sedimentary rocks, mainly turbiditic wackes to argillites, of the ~10 km thick Bothnian Supergroup. In the southern half of the terrane, this sequence is represented by the equivalent Härnö Group.
Pre-1.8 Ga exposures of this succession include intercalated volcanic rocks that range from mafic mass flows, pillow lavas and volcaniclastic rocks, overlain by dacite and rhyolite. These magmatic rocks geochemically progress from MORB, to island arc tholeiite and to calc-alkaline basalt with an island arc affinity (Kathol and Weihed, 2005). Dacites from this suite, which have been dated at ~1.96 Ga (U-Pb zircon; Eliasson et al., 2001), are intruded by 1.95 to 1.93 Ga granitoids (e.g., Hellström 2015; Lundqvist et al., 1993). Limited exposures of these volcanic and intrusive rocks are sporadically distributed over an interval of >500 km, from the Kiruna Greenstones in the Norrbotten Terrane, to small areas within the Luleå-Jokkmokk Zone in the northwestern Bothnia–Skellefteå Terrane; to the Knaften area, 240 km to the SSW (south of the Skellefte District). Further occurrences are found a further 200 to 220 km to the SSW and SSE. These volcanic and intrusive rocks are interpreted to represent the relicts of an early subduction related magmatic arc that has been obscured and disrupted by subsequent deposition, volcanism and intrusion (Skyttä et al., 2020).
Both the Bothnian Supergroup and Härnö Group are interleaved with subordinate amphibolite and metavolcanic rocks (in addition to those described in the previous paragraph), and are extensively intruded by a variety of plutonic suites, emplaced at 1.95 to 1.93, 1.90 to 1.88, 1.87 to 1.86, 1.87 to 1.85 Ga and 1.81 to 1.76 Ga.
During the interval from 1.88 to 1.85 Ga, polyphase ductile deformation and metamorphism at low pressures and variable temperatures is characteristic of the sedimentary rocks of the Bothnian Supergroup and Härnö Group. This included crustal melting, the formation of migmatites and extensive granite emplacement. Later ductile strain took place in a lower grade metamorphic regime and was restricted to localised deformation zones (Skyttä et al., 2020).
In the southern two thirds of the Bothnia–Skellefteå Terrane, volcanic rocks are a relatively minor part of the Bothnian Supergroup and equivalent Härnö Group. However, in the northern third of the terrane, the metamorphosed sedimentary rocks of the Bothnian Supergroup are structurally wrapped around significant developments of volcanic and plutonic rocks that define the Skellefte-Arvidsjaur Magmatic Province (principally comprising the Skellefte and Arvidsjaur groups and coeval intrusions). In contrast to the predominantly sedimentary sequence to the south,thes volcanic rocks have only been subjected to low-grade metamorphism and a low degree of regional ductile strain. This section of the terrane is also characterised by ~north-south trending deformation zones, which, like the much more prevalent volcanic suite in the same part of the terrane, continues northward into the Norrbotten Terrane (Skyttä et al., 2020; Bergen and Weihed, 2020). The north-south structures localise regional ductile strain, but also coincide with breaks in the stratigraphic sequence and metamorphic grade. The volcanic rocks of the >3 km thick 1.9 to 1.88 Ga Skellefte Group are succeeded to the north by the extensive, largely subaerial 1.88 to 1.86 Ga Arvidsjaur Group volcanism (Skyttä et al., 2020), which, in turn, passes north across the Luleå-Jokkmokk Zone to merge with the 1.88 to 1.87 Ga extrusives of the Porphyrite and Kiirunavaara groups of the Norrbotten Terrane. As such, these volcanic suites are correlated (Bergen and Weihed, 2020). The Kiirunavaara Group hosts the Kiruna and Malmberget iron oxide apatite deposits in the Norrbotten Terrane.
The Skellefte Group, in the southern 20 to 30 km of the Skellefte-Arvidsjaur Magmatic Province, hosts the VHMS deposits of the Skellefte District. In this mineralised belt, the structural trend changes from north-south in the north, which passes into, and dominates in the Norrbotten Terrane, to predominantly WNW-ESE, interpreted to be a separate, high strain deformation, in the southern part of the magmatic province. This latter trend then dominates, and persists southwards to the southwestern extremity of the terrane, defined by the Hassela Shear Zone, which has a similar WNW-ESE strike. The Skellefte VHMS deposits are localised in the transition between these two deformation trends, and in the transition from low grade meta-volcanic- to strongly metamorphosed sedimentary rock sequences. The transitional overlap of the two trends produced structural compartments that controlled deposition of supracrustal rocks and their subsequent deformation. These relationships are interpreted to indicate the Norrbotten and Bothnia–Skellefteå terranes were 'stitched together' during emplacement of the Skellefte-Arvidsjaur Magmatic Province. In addition, in this area, close to the southern margin of the Skellefte-Arvidsjaur Magmatic Province, turbidites and conglomerates of the Vargfors Group are intercalated with and overlie the volcanic rocks of the Skellefte Group. South and SE of the Skellefte District, coeval plutonic rocks of the magmatic province intrude rocks of the Bothnian Supergroup (Skyttä et al., 2020).
The Skellefte and Arvidsjaur groups both thin and pass laterally into volcanosedimentary rocks of the Bothnian Supergroup to the east and west, although finally, the lateral margins of the Skellefte-Arvidsjaur Magmatic Province are abruptly defined by two 'north-south' structures that are ~175 km apart, the NNE-SSW Karesuando-Arjeplog Deformation Zone (KADZ) to the west, and the curvilinear NNE-SSW to north-south Vidsel-Röjnoret Shear System (VRSS) to the east. Another structure between these two, the curved NNW-SSE to SSW-NNE trending Deppis-Näsliden Shear Zone (DNSZ), transposes supracrustal rocks and offsets the Kristineberg (western) cluster from the main Skellefteå string of VHMS deposits. Reverse east-side-up and west-side-up displacement on the Vidsel-Röjnoret Shear System (VRSS) and Karesuando-Arjeplog Deformation Zone respectively, has juxtaposed deeper levels of erosion straddling the low-metamorphic grade, low-strain volcanic rocks of the Skellefte-Arvidsjaur Magmatic Province. These uplifted deeper level rocks comprise high-grade amphibolite and locally granulite facies metasedimentary successions with partial melting, penetrative foliation and more intense deformation to the east (Thelander and Bastini, 2006). The meta-sedimentary rocks to the east and SE of the Vidsel-Röjnoret Shear System (in the east) are interpreted to belong to the Bothnian Supergroup, but differ from that group elsewhere in that they are characterised by intense magnetic banding reflecting intercalations of graphite and pyrrhotite-rich argillites with interlayered metabasalt. The meta-sedimentary rocks to the west of the Karesuando-Arjeplog Deformation Zone (the Snava-Sjöfallet Group) comprise a >6 km thick succession of weakly metamorphosed and gently folded quartz sandstone, arkose, siltstone, mudstone and conglomerate that are tentatively correlated with the Bothnian Supergroup (Skyttä et al., 2020). This pattern of reverse faulting has been attributed to late Svecokarelian east-west compression (Skyttä et al., 2020).
Whilst there are abrupt lateral changes in lithology, metamorphic grade and degree of deformation across these bounding reverse faults, there is also a gradual, but systematic change in the intensity of deformation and metamorphism in a lateral east-west and north-south direction from the central parts of the Skellefte-Arvidsjaur Magmatic Province towards its margins. The increase in metamorphism northward from the core of the magmatic province is gradual. To the south and SE, towards the strongly metamorphosed Bothnian Supergroup sedimentary succession, this change in metamorphic grade is coupled with a change in structural style. In the southern transition zone, a D1 recumbent isoclinal folding not obvious elsewhere, is overprinted by the F2 upright, north-vergent folding that characterises the Skellefte-Arvidsjaur Magmatic Province. This F2 folding was coeval to marginally later than peak metamorphism. The southward increase in metamorphism is also coincident with a series of ductile mylonites developed along a major WNW-ESE, north-vergent, south dipping and dextral deformation zone that is older than the F2 foliation. The mylonite zones are interpreted to have segmented the crust during NW-SE transpression, juxtaposing slices from different depths and degrees of metamorphism. These older structures are terminated to the east by the Vidsel-Röjnoret Shear System (VRSS). Sinistral displacement on the 'north-south' structures may be a conjugate relationship to the dextral offset on the WNW-ESE structures (Skyttä et al., 2020).
A number of tectonic models have been proposed for the development of the Bothnia–Skellefteå and Norrbotten terranes and the Karelian Craton to the north. The more recent, as detailed by Bergman and Weihed (2020) and Skyttä et al. (2020) may be summarised as follows:
• Intracratonic rifting of a coherent Archaean craton that had been assembled during a number of orogenic events between 2.9 and 2.68 Ga (e.g., Nironen, 2017). This continental rifting took place between 2.5 and 2.0 Ga, resulting in deposition of the Kovo Group and Kiruna 'Greenstones' (see below). It was characterised by episodic extension with associated dominantly mafic volcanic and intrusive magmatism and rift related, mainly siliciclastic, sedimentation over the craton and Norrbotten Terrane, probably extending to the south into the Bothnia–Skellefteå Terrane (Bergman and Weihed, 2020). This extension and concomitant crustal thinning resulted in the breakup of the cratonic terrane and development of a passive margin along the southwestern margin of the cratonic block. NW-SE aligned mafic dyke swarms within the craton are related and normal to this extension. However, a second NNE-SSW trending set of dykes is found in northwestern Sweden, possibly reflecting another rift basin aligned parallel to that trend (Bergman and Weihed, 2020). This latter trend may have been reactivated in northern Sweden during the Svecokarelian Orogeny to produce generally 'north-south' structural trends in the Norrbotten and northern Bothnia–Skellefteå terranes (Witschard, 1984).
• Inversion, compression and/or transpression. It has been suggested that at ~2.1 to 2.05 Ga, the closing pulse of the long lived extension and rifting detailed above led to the breakup of the Karelian Craton and separation of the Norbotten Terrane (e.g., Nironen, 2017). This was followed by inversion, SW-NE directed transpression, dextral strike slip and closure of the rift basin to form a suture between the Karelian Craton and the allochthonous Norrbotten Terrane prior to 1.89 Ga (Bergman and Weihed, 2020). This suture zone is occupied by the NW-SE to NNW-SSE trending Raahe-Ladoga Shear Complex in Finland which continues across the head of the Gulf of Bothnia as the generally north-south Pajala Shear Zone in northern Sweden before passing into northwestern Finland and neighbouring Norway (e.g., Henerson et al., 2015). This convergence and shortening is reflected in the unconformity between the Kiruna Greenstones and the Svecofennian sequence in the Norrbotten Terrane, and by SW vergent thrusting in the Lapland Granulite Belt within the Karelian Craton that was initiated between 1.93 and 1.91 Ga. Subsequent rotation of the bulk shortening direction at ~1.80 Ga resulted in sinistral shearing on the Pajala Shear Zone (Bergan and Weihed, 2020).
• Subduction with alternating pulses of compression and extension from 1.96 to 1.76 Ga. Prior to ~2.0 Ga the western margin of the Karelia Craton (and Norrbotten Terrane) had been a passive margin where a Palaeoproterozoic sedimentary succession and early facies of the Bothnian Supergroup had been deposited outboard of the cratonic blocks, apparently mostly on Palaeoproterozoic juvenile crust (e.g., Mints et al., 2020).
However, from 1.96 to 1.93 Ga, an interpreted, NNE-SSW striking, continental magmatic arc formed over both the Norbotten Terrane (underlain by Archaean basement) and the Bothnia–Skellefteå Terrane (floored by juvenile Palaeoproterozoic crust). This arc is composed of two different volcanic facies reflecting contamination from the respective basement rocks. It is interpreted to have been related to a generally north-south striking subduction zone, outboard of, and dipping east below the magmatic arc, the juvenile crust of the Bothnia–Skellefteå Terrane, Norrbotten Terrane and the Karelian Craton.
There is some uncertainty as to what followed, from 1.93 to 1.90 Ga; e.g., Rutland et al. (2001), Skiöld and Rutland (2006) and Guitreau et al. (2014) suggest that between 1.92 to 1.91 Ga crustal shortening and a short pulse of intervening subduction between the arc and the cratonic blocks to the east resulted with the 1.96 to 1.93 Ga magmatic arc being accreted, producing the F1 foliation seen in parts of the Skellefte District. Alternatively, extension may have commenced, with the main subduction zone to the west retreating, the development of intra-arc basin and deposition of the early stages of the Bothnian Supergroup (Skyttä et al., 2020).
Numerous conceptual models have been proposed for the development of the Svecokarelian Orogen, mostly involving complex interaction between accretionary processes and the collision of multiple continents, micro-continets and arcs (e.g. Nironen, 1997; Korsman et al., 1999; Korja and Heikkinen, 2005; Lahtinen et al., 2005, 2008; Kukkonen et al., 2008; Kukkonen and Lauri, 2009). The following is based on the model proposed by Stephens and Andersson (2015) which is simpler and more elagent, based on a study of the Svecokarelian terranes immediately to the south of the Bothnia–Skellefteå Terrane. These southern terranes have a similar geological composition and tectonic history those to the north, and were most likely formed as part of the same set of processes. All are characterised by low pressure-high temperature migmatites with associated ortho- and paragneisses developed over sedimentary, volcanic and intrusive protoliths. All of the Swedish Svecokarelian terranes are cut by the Trans-Scandinavian Igneous Belt (TIB), a north-south elongated, ~1500 x 100 to 350 km array of composite batholiths extending over the southern three quarters of the Scandinavian Peninsula, predominantly in Sweden. Intrusive ages cover the whole range from 1.85 to 1.65 Ga.
Crustal thicknesses beneath the low-pressure migmatites in the area studied were thick, varying from ∼46 km to >52 km (to 65 km in central and southern Finland). The same area is also characterised by a diffuse gravity maximum in the Bouguer gravity data, interpreted to suggest an increased excess of mass at depth. Even deeper Moho depressions up to 65 km are known further east in central and southern Finland. These anomalously high crustal thickness are in conflict with the low surface relief and isostatic balance that has characterised the whole of the area of the orogen since at least the end of the Neoproterozoic. These observations are consistent with a greater thickness of ancient, high-density, mafic igneous material in the lower crust. Density modelling work has also shown that the Moho depressions are not only associated with dense material in the lower crust but also additional dense material at higher crustal levels (Kozlovskaya et al., 2004). Analysis of these data showed a marked consistency between the spatial occurrence of migmatite, increased crustal thickness and mass excess at depth, as well as a temporal coincidence of migmatite development and the addition of hot, mantle-derived material during the principal magmatic (granitoid) events.
In the studied area, mafic underplating and high-grade metamorphism corresponded to a change in the tectonic setting from a brief interval (~10 Ma) of crustal shortening with transpressive ductile strain, followed by a longer period (20 to 50 Ma) of extension or transtension, the latter resulting in the development of back arc sedimentary basins. Anatexis under low-pressure conditions is interpreted to be related to mafic underplating and the addition of hot, mantle-derived material to the continental crust, accompanied and closely followed by major magmatic activity.
Compression versus extension is a product of whether the hinge of the subducting oceanic slab is advancing or retreating with respect to the overriding
plate front. When the slab is in roll-back, extension ensues, the over-riding continental plate is attenuated and the Moho rises to shallower depths. This, in turn, results in upwelling of the asthenosphere, which undergoes adiabatic melting due to a reduction in pressure, and/or flux melting as a result of contamination following dehydration of the underlying melting slab. The high temperature mafic material thus produced underplates, intrudes and metamorphoses the overriding plate to produces migmatites, ortho- and paragneisses, anatectic melting, mafic intrusion and volcanism, and felsic intrusions and volcanic rocks. The subduction front, in both retreating and advancing modes, progressively migrated to the west to SW with time as do the dates of various intrusive and extrusive suites (Stephens and Andersson, 2015).
The 1.96 to 1.93 Ga compressive event described above was followed by a period of retreat of the subducting plate, and extension of the overriding plate in the east. This resulted in subsidence and development of an intra-arc basin from 1.91 Ga over the 1.96 to 1.93 Ga relict arc. From 1.90 to 1.88 Ga, a new magmatic arc was generated over the thinned crust of the intra-arc basin (which was underlain by a mafic underplate), forming the first stage of the Skellefte-Arvidsjaur Magmatic Province and its VHMS deposits. This period corresponded to the deposition of the Skellefte Group and the GI Phase of the Jörn Granitoid (see below).
Geochronological and structural data suggests subsequent inversion of the tectonic regime characterised by crustal shortening at 1.88 Ga, marked by a depositional break at the top of the Skellefte Group, and by upright folding (F2) and reverse shearing along high strain zones (Skyttä et al., 2020). This resulted in exposure and erosion of the 1.90 to 1.88 Ga Jörn Granitoid GI phase, clasts of which are found in the overlying Vargfors Group.
Subsequent extension and magmatism, from 1.88 to 1.86 Ga, produced the Vargfors and Arvidsjaur groups and the Perthite-Monzonite Suite (including the GII to GIV Phases of the Jörn Granitoid), followed by further magmatism between 1.87 and 1.85 Ga (the Older Revsund Suite Granitoids). Deposition and volcanism during this extensional pulse is basically coextensive with that of the underlying Skellefte Group, although the younger granitoids are largely peripheral to the earlier 1.90 to 1.88 Ga suite.
The overlap and intersection of the dominant north-south structural grain to the north and the WNW-ESE trend to the south influenced the structural permeability of the area, and the consequent ingress of magmas producing intrusions and extrusions, as well as circulation of hydrothermal fluids. The north-south structural trend was due to reactivation of the earlier such trend within the Norrbotten Terrane, whilst the WNW-ESE structures reflected the oblique east-west approach (and retreat) of the subducting plate against the buttressing NW-SE 'cratonic' margin (as reflected by the Luleå-Jokkmokk Zone). Displacement on these two structural trends and their intersections varied from dextral to sinistral and transpressive to transtensional (and dilatant) depending upon whether the subducting plate was in advance or retreat. The same intersecting structural pattern produced localised depositional basins during extension that influenced deposition. During compression, the WNW-ESE and transform north-south trending high strain shears segmented the sequence, preserving poorly metamorphosed higher level volcanic and sedimentary rocks, structurally interleaved with deeper more strongly metamorphosed migmatitic, gneissic, sedimentary and volcanic rocks.
A short phase of renewed crustal shortening is indicated from ~1.84 to 1.83 Ga by the folding of an older ductile fabric to the south. This was followed by a longer period of igneous activity related to subduction processes along an active continental margin at 1.81 to 1.78 Ga, again in an extensional or transtensional regime (Stephens et al., 2009). This latter activity is concentrated to the south of the Skellefte-Arvidsjaur Magmatic Province, in the southern Bothnia-Skellefteå, Ljusdal and Bergslagen terranes as the subduction front retreated to the south and west.
* NOTE: The 'terranes' of the Fennoscandian Shield are referred to as 'lithotectonic units' in the Scandinavian literature, but are termed 'terranes' herein for consistency throughout the database.
Geology - Skellefte-Arvidsjaur Magmatic Province
The geology of the Skellefte-Arvidsjaur Magmatic Province can be summarised as follows, from the base:
Bothnian Supergroup - which is the oldest and dominant unit within the Bothnia-Skellefteå Terrane and Skellefte-Arvidsjaur Magmatic Province. Its immediate basement is not exposed, and is interpreted to be composed of juvenile Palaeoproterozoic crust south of the Luleå-Jokkmokk Zone. To the north, in the southern Norrbotten Terrane, it overlies or is structurally juxtaposed against 2.3 to 2.0 Ga supracrustal rocks of the Kiruna 'Greenstones' and equivalents. These comprise metamorphosed basalt, komatiite and associated sedimentary rocks which, further north, unconformably overlie Neoarchaean and older basement. The Kiruna Greenstones are unconformably underlain by the Kovo Group and equivalents which comprise basal siliciclastic rocks (conglomerates and quartzites) that are overlain by meta tholeiitc basalts. This sequence has been intruded by a 2184±5 Ma albitic mafic sill and has been correlated with a 2.5 to 2.3 Ga suite in Finland, but contains some ~2.7 Ga basalts. It rests on Neoarchaean basement in the Norrbotten Terrane. The juvenile basement south of the Luleå-Jokkmokk Zone may be equivalents of the Kiruna 'Greenstones' and/or Kovo Group or sections thereof (Skyttä et al., 2020).
The Bothnian Supergroup includes the volcanic suites of the Skellefte-Arvidsjaur Magmatic Province. Outside of the magmatic province, it is principally composed of up to 10 km of high grade metasediments, the protoliths of which are largely turbiditic wackes with argillites and subordinate debris flows that were deposited within the Bothnian Basin. These siliciclastic rocks are interlayered with graphite and pyrrhotitic schists, cherts, partly pillowed basalt and less common dacite and rhyolite. They have undergone high grade metamorphism to partial melting and migmatisation. As detailed previously, the volcanic rocks geochemically progress from MORB, to island arc tholeiite and to calc-alkaline basalt with an island arc affinity (Kathol and Weihed, 2005). Dacites are dated at ~1.96 Ga (U-Pb zircon), and were followed by the intrusion of granitoids between 1.95 and 1.93 Ga. Limited exposures of these latter granitoids are sporadically distributed over an interval of >500 km, from the Kiruna Greenstones in the Norrbotten Terrane to near the southern margin of the Bothnia–Skellefteå Terrane, as detailed above (Skyttä et al., 2020).
• Skellefte Group, deposited between 1.90 and 1.88 Ga in the southern part of the Skellefte-Arvidsjaur Magmatic Province. It is >3 km thick and is mainly composed of rhyolitic to dacitic subaqueous volcaniclastic rocks, lava domes and intrusive domes, porphyritic cryptodomes, with subordinate andesitic volcaniclastic rocks, lavas and intrusions, and minor basaltic lavas and intrusions. Particularly in the upper parts of the group, the dominantly volcanic sequence is intercalated with submarine grey to black mudstone, sandstone, breccia-conglomerate, volcaniclastic rocks with carbonate matrix, and rare limestone (Allen et al., 1996; Kathol and Weihed 2005; Montelius et al., 2007). Although predominantly submarine, local shallow water to subaerial characteristics are also evident, including erosional surfaces, oxidation facies and rounded clast conglomerates (Skyttä et al., 2020).
• GI Phase of the Jörn Granitoid, emplaced between 1.90 and 1.88 Ga, occurring as voluminous plutons of commonly equigranular granitoid with locally spatially associated ultrabasic, gabbro and diorite, within and to the south of the Skellefte-Arvidsjaur Magmatic Province. In higher grade, exhumed sequences to the south of the magmatic province, they are distinctly gneissic. Plutons of this suite have been heterogeneously affected by ductile Svecokarelian deformation. Within the Skellefte-Arvidsjaur Magmatic Province they intrude volcanic rocks of the Skellefte Group. Detrital zircons from the Bothnian Supergroup sedimentary sequence have returned both Archaean and 2.02 to 1.88 Ga ages (Claesson et al., 1993). The latter suggests granites intruded into the early Bothnian Supergroup were tectonically uplifted, eroded and exposed during a hiatus prior to the recommencement of deposition (Skyttä et al., 2020).
• The Vargfors and Arvidsjaur groups are regarded as temporal equivalents, as either may overlie the Skellefte Group, whilst the Vargfors Group commonly separates the predominantly volcanic Skellefte and Arvidsjaur groups. In other places, where the Skellefte Grpoup is absent, the Vargfors Group sits directly on Bothnian Supergroup meta-sediments and the two are visually indistinguishable. The Vargfors Group is predominantly composed of turbiditic wacke, argillite and polymictic conglomerates with clasts derived from the G1 Phase of the Jörn Granitoid. Shallow intrusions and felsic and mafic lavas are locally interlayered within the Vargfors Group, with associated subaqueous mass flow deposits of juvenile rhyolitic, dacitic and basaltic debris, and at least one subaerial pyroclastic flow deposit (Allen et al., 1996). Age dating (U-Pb, zircon) and geologic relationships indicate the basal sections of the Vargfors Group and the uppermost volcanic rocks of the Skellefte Group are of similar age, which may be as old as 1.892 Ga in the Boliden area in the eastern part of the district (Allen et al., 1996; Mercier-Langevin Allen et al., 2013). The Arvidsjaur Group occurs mostly to the north of the Skellefte Group in the Skellefte-Arvidsjaur Magmatic Province, where it comprises extensive areas of predominantly 1.88 to 1.86 Ga rhyolitic to dacitic lavas and ignimbrites, underlain by lesser andesite and basalt. These volcanic rocks locally directly overlie the Skellefte Group and extend northwards across the Luleå-Jokkmokk Zone into the Norrbotten Terrane. Conglomerates in the upper Vargfors Group contain clasts of both the Skellefte and Arvidsjaur groups. The volcanic rocks and intercalated sedimentary rocks within the Arvidsjaur Group indicate deposition in a subaerial to subordinate shallow water to alluvial setting.
• Perthite-Monzonite Suite, including the GII to GIV Phases of the Jörn Granitoid, emplaced between 1.88 and 1.86 Ga. They are predominantly granite, granodiorite and quartz-poor felsic rocks with subordinate gabbro and diorite, and have generally not been subjected to ductile deformation. This suite intrudes the Arvidsjaur Group, although most field relationships indicate a comagmatic relationship. They are predominantly ferroan and alkali-calcic in composition. With the GI Phase of the Jörn Granitoid, they are the dominant intrusive suite within the Skellefte-Arvidsjaur Magmatic Province, occupying much of a NW-SE elongated, 120 x 250 km lensoid shaped corridor, largely coincident with exposure of the Skellefte and Arvidsjaur groups. This lensoidal corridor is limited to the west and east by the Karesuando-Arjeplog Deformation Zone (KADZ) and Vidsel-Röjnoret Shear System (VRSS) respectively, and to the NE by the Luleå-Jokkmokk Zone.(Skyttä et al., 2020).
• Härnö and Older Revsund Suite Granitoids, emplaced at 1.87 to 1.85 Ga, and spatially associated with migmatites. These intrusions comprise fine-grained, garnet-bearing, leucogranites, and fine to medium-grained, equigranular to porphyritic granitoids to pegmatite. Apart from a limited exposure between Luleå and Skellefteå, they are restricted to the southern extremities of the Bothnia-Skellefteå Terrane, ~250 km to the south of the Skellefte-Arvidsjaur Magmatic Province (Skyttä et al., 2020).
• Dobblon Group supracrustal suite, deposited at ~1.8 Ga in the northwestern part of the Bothnia-Skellefteå Terrane in the west of the Skellefte-Arvidsjaur Magmatic Province. It is ~2.4 km in thickness and is composed of a variety of mono- and polymictic conglomerates and cross-bedded arkose intercalated with, and overlain by, rhyolite. The latter includes welded pumice clasts suggesting sub-aerial deposition. It rests unconformably on the Bothnian Supergroup and on 1.8 Ga granitoids, while also being intruded by some 1.8 Ga granites (Skyttä et al., 2020).
• Younger Revsund Suite and Sorsele, Skellefte and Equivalent Granitoids, emplaced at 1.82 to 1.76 Ga. These are the most widespread and voluminous granitoids of the Bothnia-Skellefteå Terrane. They predominantly occur to the SW of the Skellefte-Arvidsjaur Magmatic Province, but extend along and within the southern margins of the magmatic province, intruding volcanic rocks of the Skellefte and Arvidsjaur groups within the area where the Skellefteå Field cluster of VHMS deposits occur. They continue south to the southern limits of the Bothnia-Skellefteå Terrane, but also re-occur in the northern part of the Skellefte-Arvidsjaur Magmatic Province and extend north into the Norrbotten Terrane where they are known as the Edefors Suite. They are commonly medium to coarse grained and coarsely K feldspar phyric to equigranular with biotite and hornblende occurring with the dominant felsic minerals. They contain rounded to rectangular phenocrysts up to 100 mm across. Dark grey charnockites and subordinate mafic bodies mingle with the more felsic rocks. Deformation is either localised low grade ductile strain or is brittle.
In addition, a suite of pale grey equigranular and medium to fine grained granite, as well as heterogeneous granites with partially assimilated country rocks are found to the south of, and to the east of the Skellefte-Arvidsjaur Magmatic Province to the Gulf of Bothnia, continuing north into Norrbotten where they are known as the Lina Suite. These include the S-type 'Skellefte type' Granites which were emplaced at ~1.82 to ~1.80 Ga and are commonly intruded into migmatised sedimentary rocks and spatially associated with pegmatite and aplite which occur as dykes and irregular bodies in the intrusive and country rock (Skyttä et al., 2020).
The geodynamic and metallogenic context of the Skellefte District has been interpreted to comprise a complex pattern of horsts and grabens that developed during a period of extension, with a predominantly submarine volcanic arc emplaced on a moderately mature to young continental crust. The horsts and grabens are bounded by WNW-ESE trending extensional faults, and overlapping NNE-SSW structures extending south from the Norrbotten Terrane. Syn-volcanic extensional faults are interpreted to have controlled the passage of hydrothermal fluids to shallow levels in the sequence being deposited in these grabens. Subsequent inversion, compression and uplift are envisaged to have reactivated the early syn-volcanic extensional faults as reverse structures, including some high-strain corridors, resulting in the present steeply dipping, elongate geometry of the hosts to most of the sulphide deposits of the district. The same structural framework continued to act as a pathway for subsequent hydrothermal fluids (Allen et al., 1996; Bauer et al., 2011; Bauer et al., 2014).
Metamorphism in the Skellefte District is mainly to greenschist facies in the north, grading to amphibolite facies in the south (Kathol and Weihed 2005; Allen et al., 1996; Bergman-Weihed et al., 1996; Ödman 1941). This metamorphism and the associated compressional deformation event is interpreted to have post-dated deposition of both the Skellefte and Vargfors groups as both have similar trends and styles of deformation (Allen et al., 1996). Available isotopic age data and crosscutting relationships suggest syn-deformation metamorphism is younger than ~1.87 to 1.85 Ga, but older than 1.8 Ga (Mercier- Langevin et al., 2013). This deformation produced major NW-trending faults and gently plunging upright folds with NW-trending axial surfaces, attributed to NE-SW compression (Weihed et al., 2002) perpendicular to the early syn-volcanic faults. The latest major deformational event occurred at ~1.80 Ga (Bergman-Weihed 2001).
Much of the early literature divided the host sequence at a deposit and district scale into a Volcanic and an overlying Phyllite Group. The Volcanic Group generally refers to the volcanic sequence of the Skellefte Group, although possibly at times the Arvidsjaur Group, whilst the Phyllite Group may refer to sedimentary rocks within the upper Skellefte Group or to the Vargfors Group. These terms will be used below where information is taken from older sources.
Return to Top
SELECTED DEPOSITS within the Skellefteå - Kristineberg District
A study of the structural framework of the Skellefte District and the distribution of VHMS and related mineralisation (Bauer et al., 2014) concluded that the size, distribution and orientation of these deposits shows a strong correlation with the regional fault patterns resulting from the 1.90 to 1.88 Ga D1 upper crustal extension. The study also noted that the 3D orientation, shape and size of many of the orebodies reflects the deformation fabric of the hosting structures and, for that reason, inferred the present-day geometries mainly result from the 1.88 Ga D2 crustal shortening. A comparison of the size of VHMS deposits with their location shows that the smallest deposits are not related to known high strain zones whilst the largest (e.g., Boliden, Renström and Rakkejaur) are closely associated with regional-scale WNW-ESE and north-south to NW-SE high-strain district-scale faults. The latter, in particular, include the broad, transform, Deppis-Näsliden Shear Zone (DNSZ) and the Vidsel-Röjnoret Shear System (VRSS). Many deposits are located at, or close to the intersections of faults. While some occur within fault zones, many are hosted within volcaniclastic and sedimentary rocks in the vicinity of the faults but not exactly within the fault zone (Bauer et al., 2014).
The following are descriptions of the more significant of the deposits and resources, generally from east to west along the trend of the Skellefteå Field. All except those with an annotated location relative to another are plotted on the geological image above,
Boliden (#Location: 64° 52' 17"N, 20° 21' 45"E)
Geology - The sequence hosting the Boliden deposit belongs to the upper part of the Skellefte Group, near the contact with the sedimentary succession of the Vargfors Group. Observed younging directions are all towards the SE with no obvious folding. The oldest exposed volcanic rocks comprise coherent rhyolites and volcaniclastic rocks, capped by fine grained laminated sedimentary rocks. These are overlain by a heterogeneous assemblage of volcanic breccias, sandstones and feldspar porphyritic intrusions with a mainly dacitic composition which flank the Boliden ore. Some of the dacitic volcanic sandstones are pumiceous flows, whereas others are more reworked with well-rounded polymictic clasts. The pumiceous flow varieties are in part feldspar porphyritic ash-flows that have lithic rip-up clasts near the base, and an upward increasing amount of cm-size pumice clasts. Reworked breccias are also increasingly abundant upward, grading into conglomerate, and have a pumiceous flow matrix with polymict clasts that include coherent feldspar porphyry, angular mafic and biotite-rich sedimentary rocks, and pumice. Some conglomerate beds have a mudstone matrix. Several beds of conglomerate occur within this part of the sequence, separated by breccia and sandstone bands. The conglomerates are more polymictic, with rounded 5 to 50 cm clasts and a much higher clast:matrix ratio than the breccias. The volcanic sandstones and breccias are recrystallised, fine-grained, quartz-plagioclase-biotite rocks with scattered quartz phenocrysts. Variable sericite and chlorite are present, commonly accompanied by calcite-bearing fractures. Accessories include apatite and sulphides (Bergman-Weihed et al., 1996).
Immediately to the SE of this package of dacitic breccias and volcano-sedimentary rocks is a belt of coherent plagioclase-porphyritic dacitic rocks with a very fine grained, almost glassy matrix, which, on the basis of their massive texture and unbroken feldspar phenocrysts, are interpreted to be lavas or intrusives. The relationships between the two sequences is not known in detail, although their common texture and composition suggest they are coeval and possibly genetically linked. This relationship is supported by clasts of the coherent feldspar porphyritie dacite occurring in the polymict breccias and conglomerates which overlie the pumiceous sandstone. In addition, dacites apparently interfinger with fine sedimentary units at the contact; breccias with dacite clasts or blocks with a sandy to silty matrix occur in the underlying unit; and the dacite locally contains sedimentary fragments. These observations imply exposure and rapid erosion in the upper part of the combined sequence. The dacite has peperitic textures where it intrudes unlithified sediments of a mass-flow unit that is banded and appears baked along the contacts (Bergman-Weihed et al., 1996).
Two separate types of dacite are evident in the coherent plagioclase-porphyritic variety. The first is regionally distributed, older and fine grained, whilst the second is coarse grained and locally brecciates the first. Many of the latter dacites are porphyritic with altered and broken phenocrysts, although well preserved porphyritic textures are reasonably common. These have euhedral to subhedral phenocrysts of albite or sodic oligoclase comprising 10 to 15% of the rock, but are as high as 20% in the band partly hosting the main mineralisation. Phenocrysts are normally 1 to 2 mm across, but are up to 5 to 10 mm in the coarse grained variety, and are set in a matrix of quartz, plagioclase and biotite, variously altered to sericite. These dacites are interpreted to comprise repeated subvolcanic cryptodome intrusions into older domes and associated extrusive rocks (Bergman-Weihed et al., 1996).
The next unit to the SE is the overlying sedimentary sequence, the lowermost unit of which is a volcanic sandstone of quartz or feldspar porphyritic rhyolitic derivation, followed by brownish, biotite and plagioclase-dominated volcanic silt and sandstone of andesitic to basaltic composition. These are followed by a suite of graphite and sulphide-bearing mudstones. The remainder of the overlying sequence is divided into two main units, namely:
i). a lower unit of fine grained mudstone-siltstone with a few distinct thick sandstone beds deposited as major mass flows which can be up to 50 m thick with coarse bases, commonly including mud- and siltstone rip-up clasts, fining upward to a weakly laminated top, with an average grain size of 1 mm with bluish quartz crystals that are <3 mm in size,
ii). an upper unit of more well-defined turbidites (Bergman-Weihed et al., 1996).
This sedimentary sequence is apparently mostly derived from a volcanic and is mainly composed of plagioclase, quartz and biotite. It has been interpreted as a medial to distal turbiditc succession deposited well below the wave base (Slade, 1986; Smith, 1986).
A number of narrow massive, dark grey-green basalt-andesite bodies intrude the dacitic feldspar porphyries, volcanic sandstones and breccias near the ore at Boliden, and are in part host to ore, whilst a larger basalt-andesite unit occurs to the NE of the deposit, immediately north of the contact with the sedimentary rocks. Proximal to ore, the basalt-andesite is fine grained with <0.5 mm plagioclase phenocrysts in a matrix of feldspar, actinolite and biotite, with minor epidote and calcite. The basalt-andesite typically contains up to 1 cm quartz and/or calcite-filled amygdales (Bergman-Weihed et al., 1996).
The dacitic mass flows and intrusions are also intruded by two quartz porphyry stocks near the ore, whilst some <20 cm thick quartz porphyry dykes cut the dacite in the same area. A quartz porphyry stock immediately to the NW of the ore at surface plunges at 60°SE and approaches the ore in the deeper parts of the mine. It is invariably strongly altered, even external to the mineralisation related sericite and chlorite envelope that surrounds the ore, and is characterised by pervasive fracturing, sulphide impregnation and a sericite matrix. The quartz porphyry comprises <10% monocrystalline, bluish, and up to 1 cm quartz phenocrysts with embayments and inclusions of the finegrained matrix, which is composed mainly of quartz, plagioclase and sericite-chlorite. Any feldspar phenocrysts that may have originally been present were entirely obliterated by the pervasive alteration (Bergman-Weihed et al., 1996).
Other mafic intrusions, mostly sills, that are usually 1 to 2 m thick, occur in all rock types, including the massive ore. They have brownish, hybridised plagioclase and biotite-rich contacts with the wall rocks, and are composed of a metamorphic assemblage of actinolite, biotite and plagioclase, with accessory titanite and calcite. Rarely, a lower metamorphic grade, chlorite-dominated assemblage is present (Bergman-Weihed et al., 1996).
A north-south section through the centre of the ore reveals that all observed lithological contacts have a uniform 50°S dip, whereas the massive ore is subvertical. Shearing along the ore zone has obliterated lithological contacts through the orebody which cannot be traced from the northern to southern sides, although despite the intense alteration, it is apparently evident that more than one rock type is host to mineralisation, which seem to include feldspar porphyritic dacite and basalt-andesite which occur very close to the orebody and locally between ore lenses, whilst away from the immediate ore deposit, quartz porphyry is strongly impregnated with very fine grained arsenopyrite and pyrite (Bergman-Weihed et al., 1996).
Structure - Three ductile foliation-forming events have affected the rocks within and surrounding the Boliden deposit. These comprise a regional, steep, NE striking S1 foliation, formed during isoclinal folding, which was subsequently cut by a subvertical, east-west striking shear zone coincident with the Boliden ore zone. The latter formed a strong Ss cleavage (i.e., shear foliation) and extensive deformation of the ore itself (Bergman-Weihed et al., 1996).
The strong Ss cleavage along the shear zone in sericite- and chlorite-altered wall rocks to the ore is concordant to the orebodies (Ödman, 1941), and is defined by the orientation of these micas and the shape of very fine feldspar and quartz grains. It has obliterated almost all traces of the S1 foliation, although the S1 fabric is locally evident in less deformed shear pods within the shear zone. A stretching lineation, Ls, related to the shearing, plunges at ~55°E, and is parallel to the elongation of individual massive sulphide and quartz-tourmaline lenses as described below. Combining this plunge with the sinistral strike separation of the contact between volcanic and sedimentary units, an oblique movement with the northern side displaced west and up relative to the southern side has been inferred (Bergman-Weihed et al., 1996).
Both S1 and Ss are macro- and microscopically identical in appearance, and both are interpreted to have formed prior to peak metamorphism and S2. It is therefore probable that the shearing took place during or shortly after the regional deformation event responsible for S1 Both the S1 and Ss foliations were later crenulated by a locally developed, steep, north- to NE-striking crenulation cleavage, S2. The deformation responsible for S2 produced gentle to open folds which have axial surfaces striking north to NNE. On a regional scale, the S2 crenulation cleavage dips at 60 to 70°ESE, with a crenulation lineation, L2, that plunges at ~50°ESE which is well developed in the vicinity of the deposit (Bergman-Weihed et al., 1996).
Alteration - The composite ore zone at Boliden comprises a series of lenses of massive and vein sulphide that dip near vertical, plunge ~60°E and obliquely crosscut lithological contacts within the basalt-andesite and feldspar-phyric dacite units. It lies within a downward expanding, wedge shaped alteration pattern, which at the surface is <15 m wide perpendicular to strike on either side of the deposit. Within this alteration pattern, near surface, the mineralisation passes outwards on both sides into a sericite and then a chlorite zone before passing out into country rock. Down dip and down plunge, the ore deposit lenses out and passes into a downward widening central andalusite zone, which is flanked on both sides by the downward continuation of the sericite and chlorite zones that flanked the sulphides at shallower depths. By a depth of 500 m below surface, the andalusite zine has widened to as much as 100 m, whilst the combined alteration wedge is near 200 m wide perpendicular to strike (Bergman-Weihed et al., 1996).
The central andalusite zone immediately below the massive sulphide ore includes banded and massive andalusite-sericite-quartz rock and masses of almost monominerallic andalusite. The andalusite occurs both as minute grains disseminated along the main penetrative cleavage and as larger euhedral crystals cutting the main cleavage. Within the andalusite zone, corundum occurs locally, whilst pods of monominerallic sericite-, rutile-rich and apatite-rich rock are known (Ödman, 1941; Nilsson, 1968; Grip and Wirstam, 1970).
The sericite zone, which is composed of sericite and quartz, but also locally contains minor amounts of andalusite, is the dominant alteration assemblage and envelops the massive ore. As well as this 'normal' type of sericite alteration, Nilsson (1968) also distinguished an extremely silicic variety with up to 80% SiO2, which occurs in the deeper part of the sericite zone. Substantial arsenopyrite and chalcopyrite impregnations are are found adjacent to the ore within the sericite zone (Bergman-Weihed et al., 1996).
The outermost chlorite zone is almost entirely absent at the surface but thickens with depth. It is dominantly composed of quartz, sericite and chlorite, commonly with pyrite impregnations. The boundary with the sericite zone is commonly diffuse, comprising alternating bands of sericite and chlorite on a scale of tens of centimetres. In contrast, the transition from chlorite alteration to the relatively unaltered country rocks is always quite sharp.
In general, the pervasive character and intensity of alteration associated with the Boliden ore prohibits macroscopic identification of the precursor rocks (Bergman-Weihed et al., 1996).
Most volcanic rocks in the Boliden-Skellefte area have undergone regional alteration mainly affecting the large ion lithophile elements. K-enrichment predominates in volcaniclastic rocks, whereas Na-enrichment is most common in well preserved, feldspar porphyritic dacites proximal to the Boliden ore. Bergman-Weihed et al. (1996) suggest early hydrothermal fluids rich in CO2 altered intermediate to mafic volcanic rocks, which was followed by regional spotty metamorphic growth of actinolite. Actinolite, which is peak metamorphic mineral, is apparently absent within the alteration immediately surrounding the ore, suggesting the Boliden alteration zone was not geochemically susceptible to actinolite growth.
Mineralisation - Two ore types are differentiated at Boliden mine: i). massive sulphides which constituted the bulk of the ore and comprised ~75% massive pyrite with associated lenses and irregular bodies of massive arsenopyrite (25%) and minor pyrrhotite, and ii). vein ore which covers all mineralisation that occurs in structures crosscutting the massive ore and country rocks. Structures include a fine vein network containing quartz, sulphides and sulphosalts in brecciated arsenopyrite ore, larger veins with sulphides, sulphosalts and quartz in massive pyrite and host rocks, and quartz ±sulphide ±gold-bearing veins in tourmaline bodies, commonly found immediately below (laterally down plunge) of the massive sulphides. Sulphide and gold bearing quartz-tourmaline veining is frequently associated with lamprophyre veins and dykes which follows the existing schistosity and are selectively fractured due to it competence to form a locus for veining (Grip, 1978).
• Massive Sulphides - Pyrite mineralisation comprises >75% of the massive ore, and is divided into the separate western and eastern bodies, both with strike lengths of ~350 m. The western body is only ~15 m thick and pinches out at a depth of 130 m. The eastern body is ~50 m thick and persists to a depth of ~250 m below surface. Both pyrite bodies are elongated and plunge at ~60°E. The pyrite mineralisation has a considerable variation in grain size, from 0.5 to 5 mm, and it is commonly banded and normally strongly recrystallised (Ödman, 1941). Under the electron microprobe, Co-zoned pyrite can be seen to overgrow spongy-textured, fine-grained pyrite where the pyrite crystals are in contact with quartz (Bergman-Weihed et al., 1996).
Arsenopyrite fragments are common within the pyrite mineralisation, whilst arsenopyrite also occurs as intimate intergrowths with pyrite. Interstitial phases include chalcopyrite, sphalerite, pyrrhotite and locally, galena and sulphosalts. Rounded country rock inclusions, predominantly quartz and sericite schist, were also evident within the pyrite ore. All these fragments have apparently been incorporated into the pyrite lenses during deformation. Mylonitic banding of pyrite mineralisation commonly occurs along the margins of arsenopyrite bodies enclosed within the massive pyrite (Ödman, 1941). This banding, where observed in thin section, is defined by attenuated fragments of fine-grained arsenopyrite. Other banding was defined by layers containing sphalerite interstitial to pyrite grains (Bergman-Weihed et al., 1996).
Massive arsenopyrite usually occurs as irregular bodies, completely enclosed within massive pyrite, although it may locally also extend into the adjacent country rock. These bodies, which are usually elongate, are concentrated near the southern side of the massive sulphides, and plunge at ~60°E. Both pyrite and arsenopyrite mineralisation is banded within a metre of their mutual contact (Ödman, 1941), with rounded arsenopyrite ore fragments commonly occurring within the massive pyrite. This is interpreted to represent 'durchbewegung' texture (i.e., competent sulphide or silicate clasts in a matrix of less competent sulphides; e.g., Vokes, 1969), the result of physical structural remobilisation and recrystallisation attributed to a combination of compression and shear. These arsenopyrite clasts, as well as the more homogeneous interiors of arsenopyrite bodies, are almost universally brecciated into angular to subrounded fragments with quartz, minor sulphosalts and sulphide minerals such as chalcopyrite, pyrrhotite and sphalerite forming the matrix. The arsenopyrite mineralisation is usually very fine grained with an average grain size of 0.01 to 0.04 mm (Ödman, 1941) and is mainly composed of recrystallised euhedral to subhedral crystals. Quartz is commonly interstitial within these arsenopyrite fragments, accompanied by minor phases which include chalcopyrite, pyrrhotite, sphalerite and sulphosalts. Although mostly very fine, a bimodal grain size distribution is common within fragments, comprising extremely fine grained interiors, but coarser recrystallised margins where arsenopyrite grains commonly form well-developed rhomboid crystals protruding into the matrix. Electron microprobe studies show coarser, concentrically zoned arsenopyrite crystals enclosing finer grained spongy arsenopyrite aggregates with galena and quartz as minor interstitial phases. The arsenopyrite zonation reflects a variation in Co content. The spongy arsenopyrite is interpreted to be the oldest preserved texture within the deposit. Broken arsenopyrite crystals are rare along the fragment boundaries, taken to indicate the bulk of the deformation causing brecciation took place prior to recrystallisation, although some also occurred at a later stage, as seen by veins cutting recrystallised arsenopyrite and quartz. Apart from the type of arsenopyrite ore described above, Ödman (1941) also described extremely fine grained and compact, apatite-banded, and quartz-banded arsenopyrite ore (Bergman-Weihed et al., 1996).
The massive pyrite mineralisation is locally cut by veins of sulphosalts and quartz, the margins of which are usually strongly recrystallised with well-developed pyrite cubes occurring some distance into the veins. Embayments and inclusions within these pyrite cubes contain fine-grained fragments of arsenopyrite, chalcopyrite, sphalerite and pyrrhotite, indicating that the pyrite ore recrystallised after the incorporation of arsenopyrite fragments into the pyrite mineralisation and after the formation of the veins. Late-stage deformation in the pyrite mineralisation occurs as brittle fracturing of pyrite grains. Pyrrhotite mineralisation is commonly occurs along the contact between massive pyrite and country rock (Ödman, 1941).
Semi-massive, lead-zinc mineralisation occurs in the sedimentary sequence of mud and siltstones south of the Boliden deposit. This mineralisation is stratabound with chalcopyrite stringers overlying a chlorite-amphibole-garnet alteration in the mud and siltstones, and sericite-chlorite alteration in feldspar porphyritie dacite (Bergman-Weihed et al., 1996).
• Vein Mineralisation is mineralogically complex with, in addition to the common sulphides and calcite, a large number of sulphosalt minerals and precious metals, although quartz is by far the dominant constituent. Vein ore is most common within the brecciated arsenopyrite mineralisation, where it forms a matrix of fine network of veins between the arsenopyrite clasts. The most common constituants of these network of veins in the breccia are quartz, chalcopyrite, pyrrhotite and sphalerite, while sulphosalts are also frequently found, especially in the deeper parts of the deposit (Isaksson, 1973).
Strongly brecciated arsenopyrite ore closely correlates with the highest Cu and Au grades within the deposit, with textures indicating a component of extension in the formation of the breccia. Larger veins developed in massive pyrite and those extending into the country rock also contain galena, sulphosalts, and gold, in addition to the other common sulphides and quartz. Veins also commonly contain boulangerite, jamesonite, tetrahedrite, bournonite and gudmundite (Ödman, 1941; Isaksson, 1973).
A series of lens-shaped bodies of tourmaline alteration and gold mineralisation with tension gashes of quartz and minor sulphides occur mainly down plunge from the massive sulphide mineralisation within the sericitic alteration zone and are elongated parallel to the individual massive arsenopyrite and pyrite bodies (Ödman, 1941). Three main tourmaline orebodies were mined with grades >10 g/t Au. Multiple generations of tourmaline are recognised. A light greenish-brown, very fine grained tourmaline is cut by fibrous veins with darker greenish-brown tourmaline, with tourmaline needles normal to the vein walls. Both are cut by quartz-filled tension gashes. The tourmaline bodies are also fractured on a smaller scale, with small veinlets commonly containing quartz, sulphides, sulphosalts and gold. In the deeper parts of the deposit, randomly oriented tourmaline needles, predominantly dravite, also occur in the sericite-quartz schist (Ödman, 1941). The deepest and largest of the tourmaline ore lodes contained Sb-free sulphosalts and appreciable amounts of Ag, in contrast to the other tourmaline bodies (Isaksson, 1973).
Gold-bearing quartz veins are concentrated in an area known as the Gold Rise located down plunge beneath the massive ore in the central parts of the deposit These comprise a cluster of several narrow, parallel quartz-tourmaline veins with coarse gold and very high grades averaging ~50 g/t Au (Grip and Witstam, 1970).
Gold Occurrence - Gold is restricted to vein ore in deformational structures that crosscut massive sulphide mineralisation and locally persist into the enclosing country rocks. Within the veining, gold was encountered in four separate regimes:
i). In strongly brecciated arsenopyrite mineralisation, occurring as 4 to 200 µm rounded grains in the quartz-rich matrix with minor associated sulphides which include chalcopyrite, pyrrhotite and sphalerite. The gold grains are found along the interface between quartz and arsenopyrite fragments and as 'droplets', or locally crystals, inside the vein quartz.
ii). Within fragments of very fine grained arsenopyrite, also in strongly brecciated arsenopyrite mineralisation, occurring as <10 µm inclusions, together with Bi-Se minerals, distributed along grain boundaries of recrystallised, compositionally zoned arsenopyrite crystals and as inclusions in larger, recrystallised arsenopyrite grains.
iii). In veining containing sulphosalts and galena cutting massive pyrite. Gold grains are irregularly shaped, 20 to 100 µm across, and are closely associated with galena, sulphosalts, native Bi, quartz and stannite. Fine gold grains ~3 µm across have also been found enclosed in pyrite crystals, surrounded by sulphosalt and galena.
iv). In the tourmaline ore, where gold occurs in fractures cutting quartz and tourmaline and as a matrix to broken tourmaline crystals. In both cases, the gold was associated with the common sulphides and/or sulphosalts.
The first two of these were the most important contributors to the gold content of the deposit (cf. Mörtsell, 1931; Ödman, 1941; Grip and Wirstam, 1970).
All of the gold in the deposit occurs as an Au-Ag-Hg alloy with considerable variations in compositions, from Au0.17Ag0.68Hg0.16 to Au0.93Ag0.07 with an average of Au0.56Ag0.39Hg0.05 (atomic proportions). The lowest contents were for gold associated with sulphosalt veins cutting massive pyrite, whilst the highest occurs in a gold associated with aurostibite [AuSb2] (Bergman-Weihed et al., 1996).
In summary, Bergman-Weihed et al. (1996) note that the Boliden ore is not bound to one particular host rock but occurs in feldspar porphyritie dacite, quartz porphyry and basalt-andesite, which textural observations suggest represent intrusions or lavas. Geochemically, they are typical calc-alkaline volcanic rocks, enriched in large ion lithophile elements (LILE), depleted in heavy rare earth elements (HREE) and with troughs for Th, Nb, Hf and Ti. The ore zone, in its present setting, is sub-vertical and oblique to lithologieal contacts. Ore-related hydrothermal and regional metamorphic processes to lower amphibolite facies, have created a complex alteration system enveloping the ore, forming a symmetric pattern with an inner sericite-rich zone, locally containing abundant andalusite, and an outer chlorite-dominated zone. They conclude that the nature of the alteration is consistent with leaching of elements and a silica-alumina-rich residue, features which are often found in epithermal environments.
Return to Top
Björkdal
The Björkdal gold deposit is located at the contact between a Jörn G1 Phase intrusive granodiorite to tonalite and supracrustal volcanic rocks belonging to the Skellefte Group. Gold mineralisation consists of cm- to m-thick, subvertical, auriferous quartz veins within the granodiorite (Weihed et al., 2003; Gold Ore Resources 2011). The veins mainly trend NE and NNE. The gold occurs as both free milling and associated with pyrite (Hallberg et al., 2016). See the separate Björkdal record for more detail.
Return to Top
Långsele
The Långsele deposit is located ~5 km SW of Boliden. It was discovered via tracing mineralised boulders and followup with electrical geophysics in 1926, before being delineated by underground development between 1947 and 1955. It came into production in 1956, with ore transported via a 5 km tunnel to Boliden at the 470 m level. Mineralisation occurs as at least 5 lenses over a NE trending strike length of 360 m and persists to a depth of >400 m (Grip, 1978). The ore lenses dip at 55°SE and plunge50°S. Mineralisation is located at the transition between a volcanic and a sedimentary/phyllite package, known locally as the Volcanic and Phyllite groups, and is related to a syncline with a fold axis plunging at 50°S. Mineralisation is interpreted to occur at the intersection between this lithological contact and a NNE striking zone of schistosity, with mineralised lenses being controlled by both bedding planes and schistosity. The volcanic rocks, which are predominantly in the footwall of the mineralisation, are quartz porphyries or felsites, which are overlain by a thin layer of black phyllite, followed by several tens of metres of dacite or volcano-sedimentary rocks of dacitic composition. There is a probable discordance between the Volcanic and Phyllite groups. The volcanic sequence is cut by abundant pre-mineral amphibole-rich greenstone dykes with associated weak developments of sulphosalts and galena-bearing quartz veins (Grip, 1978). The schist zone mentioned above is the result of sericite alteration which occurs in the footwall of the mineralisation. It follows the contact between the mineralisation and the overlying Phyllite Group for ~1 km to the NNE and contains significant pyrite which is locally concentrated into schlieren up to several metres thick. The main complex sulphide ore is predominantly composed of pyrite with associated sphalerite and pyrrhotite, and subordinate chalcopyrite, galena, arsenopyrite and occasional sulphosalts. It has the highest mercury content in the Skellefte District, averaging ~250 ppm Hg, which is almost exclusively within the sphalerite lattice. Several stages of mineralisation have been recognised. The earliest was galena bearing quartz veins associated with the greenstone dykes, which is equivalent to a lamprophyre stage at Boliden. This was followed by the dominant complex sulphide ore, and finally by a third stage associated with the pyritic schlieren of the schist zone (Grip, 1978).
Return to Top
Långdal
The Långdal deposit is located ~8 km SW of Boliden. It comprises three en echelon lenses which together define an ~400 m strike length with a north-south trend, and a dip of ~70°E. The individual lenses plunge at ~40°SSE. The country rock in the immediate footwall of the deposit is predominantly quartz porphyries that are frequently felsitic. Alteration is moderate, largely composed of sericite with disseminated pyrite, grading to a quartz-schist as ore is approached. Disseminated pyrite and sphalerite persist well into the footwall. Stratified dacitic tuffs predominate in the hanging wall, while skarn alteration is developed adjacent to ore, represented by chloritic schist with variable amphibole, cordierite and andalusite. Distal to ore, overlying layers of black and grey phyllite with interbedded limestone are evident. Higher in the sequence, dacitic beds and lavas appear, followed by quartz porphyry and then ~300 m to the east, the main Phyllite Group (see the Långsele description above). The central or 'A' orebody has the highest grades and most sulphides, mainly pyrite, sphalerite and galena with lesser chalcopyrite and sulphosalts. The pyrite decreases downwards where silver increases and the ore is similar to that found in the flanking 'B' and 'C' orebodies, where the sulphide grades are about half of those in the 'A' orebody. All three orebodies narrow down to thin stalks where the highest silver grades are concentrated (Grip, 1978).
Return to Top
Åkulla Västra and Östra
The Åkulla Västra (West) deposit is located 7 km NW of Boliden and was mined between 1847 and 1957. The orebody was 155 m long, up to 20 m thick and persisted to a depth of 140 m down a 45° plunge with an azimuth of 330°. The western side of the main orebody was folded around a north-south trending, horizontal fold axis. The country rock comprises quartz porphyry, 'keratophyre' and dacite with interbedded pyroclastic rocks. Mafic dykes, similar to the lamprophyres at Boliden, cut the volcanic rocks, but predate the mineralisation. The sulphide mineralisation is massive and fine grained, with vague banding and a stong pyrite content, accompanied by chalcopyrite and rarely sphalerite and other sulphides (Grip, 1978).
Åkulla Östra (East) lies 700 m to the NE. It has a length of ~400 m, a maximum width of 180 m and is developed over a vertical interval of at least 180 m, occurring as two lenses. It shows a conspicuous schistosity that strikes at 150° and dips 85°E, with a steeply plunging lineation to the SE. This structural framework controls the geometry of the ore lenses which dip more shallowly than those at Åkulla Västra (West). The host country rocks are the same as at Åkulla Västra (West), but with less intense sericite alteration, but much coarser grained sulphides. The latter comprise pyrite, chalcopyrite and minor pyrrhotite, sphalerite and arsenopyrite. In addition, disseminated and joint filling chalcopyrite is also found. At the 180 m level, a number of east-west striking, steeply south dipping quartz veins occur within sericite schist that has been strongly chloritised with accompanying disseminated pyrite. The veins contain chalcopyrite and significant gold (Grip, 1978).
Return to Top
Kankberg
The Kankberg deposit is located ~8 km NW of Boliden. It has a strike length of ~70 m, maximum width of 15 m and persists to a depth of at least 300 m, plunging at 55°S. The country rock comprises felsic porphyries and keratophyres (i.e., sodic trachyte) with interbeds of tuff, particularly in the footwall. The hosts have undergone sericite alteration adjacent to mineralisation, which is composed of fine grained sulphides, predominantly pyrite, with associated sphalerite and pyrrhotite, accompanied by minor chalcopyrite, galena and arsenopyrite. This complex sulphide mineralisation is surrounded by a halo of fine grained pyrrhotite. The pyrrhotite halo mineralisation has a gradational boundary with the inner pyritic sulphide core, but a sharp contact with the countryrocks, which, in turn, contain disseminated sulphides (Grip, 1978).
Return to Top
Renström (#Location: 64° 55' 25"N, 20° 5' 35"E)
The Renström deposits are located ~15 km WNW of Boliden, in the eastern part of the Skellefteå district, and are part of a cluster of five zinc- and gold-rich volcanic-hosted massive sulphide deposits, comprising at least 12 ore lenses, concentrated within an area of 4 km2, namely: Renström, Kyrkvägen, Renström East, Petiknäs North and Petiknäs South.
Geology - The Renström deposits lie within a complex volcanic stratigraphy and multiply deformed rock sequence. Rock types include a wide range of basaltic-andesite to rhyolite volcanic facies. Juvenile basaltic-andesite, dacite and rhyolite volcaniclastic facies, which are particularly abundant, have been intruded by numerous basaltic, andesitic, dacitic and rhyolitic sills and domes, although lava flows are uncommon. Allen and Svenson (2000) recognised 44 facies on the basis of a combination of phenocryst mineralogy, rock texture and lithogeochemistry. Petrochemistry suggest the rocks are subalkaline and are composed of at least four magmatic suites (Allen and Svenson, 1999).
From bottom to top, the stratigraphic succession comprises (after Allen and Svenson, 2000):
• Diffusely stratified andesitic breccias - which comprises more than 1 km of diffusely stratified basaltic andesite, andesite and locally dacite breccias, lesser sandstones and siltstones of composition similar to the breccias, and abundant basaltic andesite and andesite sills. It is interpreted to represent the proximal to medial facies association of a large andesite shield volcano.
• High Ti dacitic pumice breccia - which conformably overlie the andesitic breccias above a sharp contact and comprise 10 to 120 m of massive to stratified, dacitic pumiceous rocks, characterised by 10 to 15%, 1 to 1.5 mm feldspar, but no quartz phenocrysts, and has a distinctive high Ti-Fe-P chemical composition. The thick pumiceous beds are interpreted as syneruptive pumiceous mass flow deposits derived from large pumiceous pyroclastic eruptions. Heterogeneous, more thinly stratified sequences, and crystal- and/or lithic-rich intervals, are interpreted as variably reworked pyroclastic debris. Moderately feldspar porphyritic dacite intrusions and lava, as found in the footwall to Kyrkvägen and Petiknäs South have a texture, phenocryst mineralogy, and chemistry similar to the high Ti dacitic pumice breccias.
• Rhyolitic, vitric sandstone-siltstone and feldspar-phyric pumice breccia - This suite conformably or disconformably overlies the high Ti dacitic dacitic pumice breccia east of Kyrkvägen. It appears to be absent at Kyrkvägen, Ren-ström and south of Renström, but thickens progressively eastwards to become 100 m thick 1.5 km along strike from Kyrkvägen. It is dominated by vitric sandstone-siltstone, has a distinct brown colour due to pervasive biotite in the rock matrix, and has a distinct rhyolitic chemistry. The pumice breccia interbeds mainly comprise intervals of the vitric sandstone-siltstone with abundant matrix-supported, ragged, brown, variably compacted, feldspar phyric pumice clasts.
• Renström ore host unit: rhyolitic pumice breccia, vitric siltstone-sandstone and porphyritic intrusions - east of Kyrkvägen, this unit conformably overlies rhyolitic vitric sandstone-siltstone of the previous unit, but further west and south at Renström, south of Renström and in the Petiknäs South block, it conformably or disconformably overlies the deeper high Ti dacitic pumice-rich. Despite transgressing the underlying sequence, it is laterally continuous over much of the Renström area, although it varies from 20 to 200 m in thickness. The thickest sections are south of the main Renström deposit where the unit mostly comprises a weakly porphyritic rhyolite dome or sill in the east and a strongly quartz-feldspar porphyritic sill in the west. The clastic, sedimented component (i.e., total thickness minus intrusions and/or domes) is not known to exceed 70 m true thickness and is thickest at the Renström deposit. The base generally comprises 1 to 10 m of dark grey to black tuffaceous siltstone with or without a few thin beds of limey siltstone. This is overlain by a 20 to 70 m of alternating pumiceous deposits, vitric siltstone-sandstone and grey to black siltstone. The pumiceous deposits have been divided into seven different facies which may be summarised into three groups: i.e., Facies A - diffusely to sharply bounded beds of monomict, rhyolitic pumice blocks up to 3 m in size (average 20 cm), that are matrix- to clast-supported in a matrix of rhyolitic siltstone-sandstone or grey-black siltstone. These are interpreted to have been deposited by the settling from subaqueous suspension of large pumice blocks and comagmatic ash or ambient hemipelagic siltstone; Facies B - massive to normal graded, monomict pumice breccia-sandstone without siltstone matrix, attributed to subaqueous mass flow sedimentation sourced syneruptively from moderate size pyroclastic eruptions at nearby subaqueous or subaerial vents; and Facies C - massive to normal graded, matrix- to clast-supported, polymict pumice breccia with two or three different pumice clast types, interpreted as slump-generated mass flow deposits, sourced locally from collapse of parts of this unit and the upper footwall succession.
The number of pumice facies within the Renström ore host unit and the number and thickness of beds of each varies from one part of the area to another. Close to mineralisation, the pumiceous deposits are generally strongly altered to chlorite or sericite, with the only relict primary textures being diffuse dark phyllosilicate patches (after pumice clasts) with a porphyritic distribution of relict quartz phenocrysts. Quartz-feldspar porphyritic rhyolite sills, cryptodomes and partly emergent cryptodomes are common within and close to the ore host unit, where they have coherent cores and either coherent or hyalo-clastic margins. Several of the hyaloclastic margins are also peperitic indicating emplacement while the ore host unit was water-saturated and unlithified. The rhyolites are strongly altered close to mineralisation, and at least locally there is a close spatial association between the strongly porphyritic rhyolites and mineralisation. All known occurrences of the ore host unit have bed forms that suggest deposition in a subaqueous environment below wave base.
• Grey to black mudstone and sandstone, which is up to 50 m thick and conformably overlies the ore host unit. At Renström and Kyrkvägen, the base of the unit comprises up to 20 m of thick- to thin-bedded, feldspar-quartz crystal-rich volcanic sandstone with interbeds of tuffaceous siltstone and minor black mudstone. The lower sandstone beds are commonly ungraded or irregularly graded, whilst the upper beds are distinctly normal graded. The sandstones are interpreted to be turbidites derived from local slumping or reworking of the top of the underlying ore host unit. The sandstone suite grades upward into grey and black mudstones and thins laterally away from the Renström and Kyrkvägen deposits. The unit is cut by a series of fine-grained, locally microdoleritic, andesitic sills that are up to 50 m thick.
• Rhyolitic to dacitic quartz-poor pumice-lithic breccias, which conformably overlie the grey to black mudstone and sandstone and comprise a thick succession of felsic, pumice-rich mass flow deposits with thin interbeds of grey to black mudstone. The lower 50 to 300 m is characterised by mass flow deposits with <5%, and commonly ~2% or less of 0.5 mm diameter or less quartz crystals. These have compositions that range from low silica rhyolite to dacite and locally felsic andesite and are mostly monomictic, pumice-rich with a juvenile composition, except for minor lithic clasts. The beds are graded and are attributed to subaqueous clastic mass flows of freshly erupted pumice-rich pyroclastic debris in a subaqueous environments below wave base.
The succession of juvenile mass flow rocks has been subdivided into i). a rhyolitic member characterised by 1 to 5%, 0.5 mm quartz crystals and a low silica rhyolite composition which occurs at the base of the unit to the west, north and NE of the Renström A-B lenses; ii). a dacitic member characterised by <2%, 0.5 mm quartz crystals with a mafic rhyolite to felsic dacite composition, occurring at or near the base of the unit on most of the eastern side of the Renström deposit, although it is interpreted to stratigraphically overlie the rhyolitic member; and iii). a minor mafic dacite member with 25 to 30% crystals including 1 to 4% quartz. whereas the dacitic members occur at or near the base of the unit on most of the eastern side of the Renström deposit.
• Rhyolitic, quartz crystal-rich volcaniclastic rocks and intrusive domes, which conformably overlies the rhyolitic, quartz crystal-poor mass flow rocks of the previous unit, is distinguished by its more quartz crystal-rich and more felsic composition. It is at least 100 m thick and occurs NE of Kyrkvägen and in the northern part of the Petiknäs South block. West of the Renström A-B lenses, this unit was not deposited and the overlying andesitic breccias, lava and black mudstone were deposited direct onto the preceding unit.
• Andesitic breccias, lava, intrusions and black mudstone - the felsic mass flow succession units described above are overlain NE of Kyrkvägen and west of Renström by 50 to 200 m of coherent and clastic basaltic andesite with minor interbeds of black mudstone, the top of which has not been encountered. To the west of Renström, the basaltic andesites of this unit are weakly feldspar-porphyritic, whilst to the NE of Kyrkvägen they are pyroxene-porphyritic. Although these two mafic lithologies appear to occupy similar stratigraphic levels, they are petrographically and chemically different, and may belong to different magmatic suites. Both exposures of this unit include thick breccia beds containing globular fluidal shaped bombs, and are interpreted as pyroclastic fallout from submarine fire fountain eruptions. Such eruptions are commonly fed from elongate fissure vents along active faults. The coincidence of subaqueous fire fountain deposits, hyaloclastites and black shales suggest deposition in a subaqueous environment, below wave base, and may be the product of a second marine andesite shield volcano in the Renström district.
Intrusions cutting this sequence that correspond to the various volcanic units (after Allen and Svenson, 2000) include:
• Pre-mineral high Ti dacite sills and lava,
• Pre- to syn-mineral porphyritic rhyolites (five types),
• Pre- to syn-mineral fine-grained rhyolite sills,
• Post-mineral fine-grained rhyolite sills,
• Post-mineral, pre-F2, andesite, and hybrid andesite-dacite and andesite-rhyolite sills,
• Postmineral, pre-F3, post-F2 strongly porphyritic dacite intrusion,
• Postmineral, pre-F3, basaltic andesite intrusions,
• Postmineral, syn- to post-F3, high Mg (komatiitic) basaltic andesite dykes.
Stratigraphic correlation suggests that the Renström, Kyrkvägen, and Petiknäs South ore deposits occur at approximately the same stratigraphic level, i.e., the Renström ore host unit as described above, whereas the Renström East deposit occurs in the footwall rocks and the relative stratigraphic position of the Petiknäs North deposit is uncertain (Allen and Svenson, 2000).
Lithogeochemical data suggest that the main Renström ore stratigraphic position coincides with a change from a high Ti (transitional to tholeiitic) magmatic suite to a low Ti (transitional to calc-alkaline) suite (Allen and Svenson, 2000).
Structure - The Renström district is punctuated by two major faults and fault-controlled intrusion which juxtapose four structural blocks containing the sequence from different levels with differing facies, thicknesses, deformational history and character. These are the WSW-ENE trending Petiknäs Main Fault in the west, which separates the Petiknäs North and South blocks to the NW and SE respectively. Further to the east, the NNE-SSW Renström Fault juxtaposes the Petiknäs South Block against the Renström Block. Less than 1 km to the east of the latter fault, a generally north-south intrusion directly east of the Renström mine, the Main Dacite Intrusion, defines the western margin of the Renström East Block. Each of these blocks hosts massive sulphide deposits with the same name (Allen and Svenson, 2000).
The Renström Fault Zone is a broad, up to 400 m wide zone of steeply west-dipping shears and faults, intruded by a swarm of high Mg basaltic andesite dykes. Kinematic indicators suggest a major component of dip-slip displacement and dextral displacement, although offset of the stratigraphy also indicates sinistral movement implying multiple events (Allen and Svenson, 2000).
The Petiknäs Main Fault Zone is a moderately south-dipping, post-F2 shear zone and thrust fault which has presumably accommodated considerable displacement as no stratigraphic correlation across the fault zone has been recognised to date. The Petiknäs North deposit lies beneath the fault zone, in the Petiknäs North structural block, and its top has probably been truncated by the fault zone (Allen and Svenson, 2000).
The Petiknäs North and South blocks (in the west of the district) have been subjected to one main set of broad, tight regional folds with WNW-trending axes, although some outcrops preserve evidence of both an earlier and a later fold generation. In contrast, the Renström and Renström East blocks (in the east of the district) exhibit two main generations of folding forming a complex interference pattern. The older folds (F2) are mainly east-west to WNW trending, tight to isoclinal, upright folds. The younger folds (F3) trend north-south, are open to very tight, upright to slightly overturned, and mainly plunge either steeply north or steeply south. The different generations of cleavage related to these fold generations vary in intensity from place to place, with the strongest cleavage in one area is not necessarily the same fabric as the strongest cleavage a few hundred metres away. At Renström, F3 are a local series of structures related to deformation along the Renström fault zone. They are apparently part of a 900 m wide, NNE trending corridor of strong deformation that envelopes both the Renström and Kyrkvägen deposits. This corridor is parallel to the Renström Fault Zone, which forms its western margin, whilst to the east, it gradually fades eastward from the two deposits (Allen and Svenson, 2000).
There is a strong L3 lineation in the Renström and Renström East blocks which is commonly the dominant fabric. It appears to be a combination of stretching lineation and S2-S3 intersection lineation and plunges from 80°S to SE, to 80°N (Allen and Svenson, 2000).
Structural fabric correlation suggests that the first known generation of regional folds in the Petiknäs South, Renström and Renström East structural blocks are the same set of WNW to east-west trending F2 folds, whilst that the second generation of north-south trending F3 folds at Renström are local, related to deformation along the Renström Fault Zone (Allen and Svenson, 2000).
The Main Dacite Intrusion which separates the Renström and Renström East blocks, encloses the Kyrkvägen deposit and separates it from the Renström deposit to its SW. The intrusion dips steeply east, at an angle that is ~5 to15° shallower than bedding. This results in the dacite retreating eastward from the Renström A-B lenses with depth. The western contact of the intrusion is weakly sheared, whilst to the east it is bounded by both primary intrusive and fault contacts. Stratigraphic correlation implies that the sequences to its west and east are structural repetitions of the same succession. The intrusion has a moderate tectonic lineation that postdates the strong hydrothermal alteration at Kyrkvägen and Renström East. It also appears to truncate the F2 folds at Renström but is cut by the Renström Fault Zone and the suite of high Mg dykes. This implies that the Main Dacite Intrusion has intruded along a subtle but important thrust fault that postdates the ore deposits and early deformation, but predates the Renström Fault Zone (Allen and Svenson, 2000).
Within the immediate Renström deposit area, overprinting of shallowly to moderately plunging, upright, east-west F2 folds and younger steeply plunging, upright, north-south F3 folds has produced a tight dome and basin style interference pattern, which due to the effect of intrusions and shearing has some irregularities. The Renström A-B massive sulphide lenses occur near the hinge of a refolded, tight to isoclinal F2 anticline and syncline. At the northern end of this structure the A lense is refolded by an F3 fold with an axis plunging at 75°SSE, and continues eastwards with that moderate to steep plunge. These folds are disrupted by cleavage-parallel shears and later, crosscutting brittle-ductile faults. The general strike of the A-B lenses at higher levels is ~north-south with an ~80°E dip, whilst the strike of the deeper section of the combined lens is near east-west. The Simon lens lies between the 950 and 1200 m levels and is separated from the deep A-B lenses by a refolded syncline containing hanging-wall rocks.
The distribution and shape of the other lenses (as listed in the introductory section of this record above) are similarly influenced by these dome and basin structures.
The Kyrkvägen deposit lies within a tight F3 anticline and the influence of F2 folding is uncertain. The structure of the Renström East deposit is uncertain, largely because no distinct stratigraphic marker occurs close to the deposit. It is interpreted to occur in the sheared hinge region of an F2 anticline. All of the rocks in the Renström area have been metamorphosed to greenschist facies (Allen and Svenson, 2000).
Mineralisation - The Renström cluster of ore lenses and the nearby Kyrkvägen and Renström East deposits, are dominantly characterised by massive to semi-massive pyrite-sphalerite mineralisation, with subordinate massive to semi-massive pyrite-chalcopyrite and local stringer-type pyrite-chalcopyrite ±pyrrhotite. The principal ore minerals are pyrite, sphalerite, galena, chalcopyrite, pyrrhotite and arsenopyrite, with minor tetrahedrite-tennantite, other sulphosalts, electrum and amalgam (Helfrich, 1971; Kläre, 2001). Mineralisation in the Renström area has higher zinc, gold, silver and lead contents and lower sulphur and arsenic than most other VHMS deposits in the Skellefte district (Allen and Svenson, 2000).
In detail each of the Renström orebodies consists of several smaller lenses, which is partly a primary feature of the original ore deposition and partly due to later deformation. Above the 600 m level, the Renström deposit is composed of two main lenses, A and B. The latter merges with the A-lens downwards, and then continues, but significantly tapers to the 1750 m level. The two lenses separately and together are developed iver a vertical interval of more than 1700 m, with maximum horizontal width of ~400 m and maximum thickness of ~20 m.
Shearing of the sulphide mineralisation has produced a range of ore textures including recrystallisation, lenticular mylonitic structures, fine-grained cataclastic sulphides and durchbewegung texture (Duckworth and Rickard, 1993). The fine-grained cataclastic and durchbewegung sulphides are typically sphalerite-galena rich and occur in distinct veins or shoots such as the south end of A-lens and the thin ore shoots that extend northwards out of B-lens. They are interpreted to be mechanically remobilised mineralisation that was 'squeezed' out of the more ductile, high grade, massive sphalerite- and galena-rich parts of the deposit during folding and shearing. The less ductile pyrite-rich ores were deformed in situ without being remobilised.
The orebodies are typically surrounded by a complex envelope of partially stratabound, strong chlorite, sericite and silica alteration, commonly with associated disseminated pyrite ± other sulphides, whilst lenses and pods of patchy to semimassive dolomite are common within 50 m of ore. Sodium depletion has proved very effective in defining areas of strong alteration likely to be related to ore formation which falls within the 1% Na2O contour. The dolomite alteration contains relict quartz porphyritic textures, indicating it is an alteration product of the quartz porphyritic pumiceous host facies and was not deposited as a seafloor chemical sediment. The footwall and ore host unit are generally much more strongly altered than the hanging wall rocks. However, the strong chlorite-dolomite alteration is interpreted to extend through the ore host unit stratigraphically upward into the hanging-wall rocks at the southern end of B-lens, suggesting the mineralised system postdated, or persisted during deposition of the 'rhyolitic to dacitic quartz-poor pumice-lithic breccias' unit. Calcite impregnation is common in the stratigraphic hanging wall and footwall rocks below and lateral to the zones of chlorite, sericite and silica alteration and is interpreted to be a weak to moderate, distal alteration.
A porphyritic rhyolite occurs in the upper levels of the A-B lenses and is directly associated with mineralisation over its entire strike length. It occupies most of the ore host unit to the B-lens. This is one of many such porphyritic rhyolite sills, cryptodomes and partly emergent cryptodomes that are close to or within the ore host unit at Renström (Allen, 1992). Allen and Svenson (2000) suggest the spatial association between ore and rhyolite is so strong, particularly on the 200 m level, that it may be conclude that the rhyolite exerted an influence on location of ore, either via a genetic connection or that the rhyolite formed a permeability barrier that focussed the mineralising hydrothermal solutions. The rhyolite is intensely altered and mineralised and consequently was clearly emplaced before or during ore formation.
The small (0.328 Mt Mineral Resource, 2019) Fingal Au-Cu zone has a greater concentration of chalcopyrite stringers (Nylander, 2004; Collin, 2019) compared to the other ore lenses of the deposit. It lies at a level immediately above the Simon lenses and has a resource that grades 1.62% Cu 0.38% Zn, 0.05% Pb, 1.07 g/t Au, 16 g/t Ag, in contrast to the more Cu poor and Zn rich Renström A-B that averages 0.58% Cu 6.41% Zn, 1.47% Pb, 1.98 g/t Au, 123 g/t Ag (Collin - Boliden, 2019).
Renström is interpreted to have originated as sub-seafloor mineralisation because the ore lenses occur within rapidly deposited, pumiceous beds rather than in slowly sedimented siltstone facies (Allen et al., 1996). Also, there is no consistent pattern of metal zonation within and/or between different ore lenses (e.g., Kläre, 2001). The rhyolitic rocks associated with the Renström ore are interpreted as part of a rhyolite cryptodome-tuff volcano and the ores occur in the proximal (near vent) part of this volcano (Allen et al., 1996).
Return to Top
Petiknäs South
The Petiknäs South pyritic massive sulphide deposit occurs ~500 m south of Petiknäs North and ~2 km WNW of the Renström mine in the Petiknäs South structural block, as detailed in the Renström description above. Petiknäs is connected to Renström underground by a 2.5 km long drive at the 800 m level, and via a decline to the surface. The deposit, which is vertically dipping with a steep to moderate plunge to the SE lies on the southern limb of a steeply plunging, tight, upright, F2-anticline. Folding is less complex than in the Renström Block to the east, with only one major fold generation being recognised, as reflected by the rocks, which have been metamorphosed to greenschist facies and generally only having one penetrative tectonic foliation (Jonsson and Svenson, 1991; Schlatter 2007). However, the deposit directly overlies the NE-trending, moderately south-dipping Petiknä̈s Main Fault Zone, a major thrust fault, which deforms and truncates the down-dip portion of the deposit at the 700 m level (Allen and Svenson, 2000; Schlatter 2007).
Geology - The mine stratigraphy strikes NW-SE to WNW-ESE, dips subvertically and youngs consistently southwards. Allen and Svenson (2000) described the seqeunce at Petiknäs South as commencing with Lower Footwall andesitic breccias, followed by Upper Footwall high-Ti dacitic pumice breccias and sills that are overlain and intruded by up to 800 m of coarsely feldspar-porphyritic and quartz-feldspar-porphyritic rhyolite sills and cryptodomes. Jonsson et al. (1995) recognised five different rhyolites based on the size and relative abundance of feldspar and quartz phenocrysts. These rhyolites are found at the top of the footwall sequence, directly stratigraphically below the main B and C massive sulphide lenses, and are chemically similar to the quartz-feldspar-porphyritic intrusions close to the Renström ore lenses. The B and C lense host unit at Petiknäs South is composed of 20 to 40 m of rhyolitic tuffaceous sandstone and grey siltstone which is overlain by 30 m of mixed felsic and mafic pumiceous mass flow breccias, and in turn by up to 80 m of tuffaceous siltstone-sandstone with abundant scattered coarsely quartz-feldspar-porphyritic rhyolite pumice clasts. This rhyolitic pumiceous siltstone unit hosts the smaller D and A sulphide lenses and is texturally and chemically similar to one of the coarsely porphyritic pumice host units to the Renström ores and may be the same stratigraphic unit. The uppermost unit at Petiknäs South comprises >100 m of normal-graded andesitic breccia mass flow beds with lesser rhyolitic beds that are intruded by rhyolite, dacite and andesite sills.
In more detail, Schlatter et al. (2007) recognised six mine-scale units, from north to south i.e., from footwall to hanging wall, as follows:
Lower Footwall
• Unit 1a - diffusely stratified andesite breccias containing abundant andesitic scoria lapilli and andesitic lithic clasts. It comprises a dark-green to beige-green, chloritic, weakly feldspar-phyric breccia. Chemically it is mainly of basaltic-andesite and andesitic composition. This unit is interpreted to have been deposited in a subaerial or shallow subaqueous environment.
• Unit 1b - A ≥100 m thick dacitic pumice breccia, that occurs as a dark-green to blue-grey, weakly feldspar-phyric pumice breccia with a characteristic high-Ti dacitic composition (Allen and Svenson 2004). The original composition of the pumice clasts is uncertain as they have been almost completely replaced by chlorite and sericite. It is intercalated with a monomictic basaltic-andesite breccia containing globular bombs and pinkish andesitic lithic clasts with chloritic rims, within a matrix of pale-grey weakly flattened scoria clasts. The uppermost section of Unit 1b locally comprises ~150 m of coherent, both strongly and moderately feldspar-porphyritic rhyolite with brecciated margins characterised by angular blocky curviplanar jigsaw-fit clasts. These rhyolites locally display pumiceous texture at their margins. Some 1 to 10 m thick dacitic volcaniclastic beds are intercalated with the rhyolite.
• Unit 1c - a 100 to 150 m thick dacite sill hosted entirely within Unit 1a, occurring as a green, fine grained, massive rock that is feldspar phenocryst-poor and contains chlorite and carbonate patches, with some sericite bands and minor patchy opaques.
Upper Footwall
• Unit 1d - mainly a strongly feldspar-porphyritic rhyolite, containing 5 to 20%, 1 to 3 mm albite plagioclase phenocrysts, with weak sericite alteration. Numerous primary volcanic microtextures are evident, e.g., pumiceous, circular spherulitic and perlitic texture. They are up to 150 m thick
at the proximal footwall, but thin towards the east and are absent in the distal footwall. In places coherent rhyolites grade into monomictic breccia which may be either clast or matrix supported. Thin sills of strongly feldspar-quartz porphyritic rhyolites occur at the base and top of the unit. This unit is interpreted to represent multiple generations of subaqueous cryptodomes (shallow intrusive domes) and sills. Thin Unit 1b relict volcaniclastic beds are evidence that the cryptodome-sill complex has mainly intruded into the dacitic footwall units proximal to the Petiknäs South deposit. Units 1b and 1d commonly exhibit hyaloclastitic and peperitic contacts.
Host Unit
• Unit 2 - mainly composed of relatively featureless interbedded rhyolitic tuffaceous sandstone and siltstone with good sorting and thin planar bedding. Although only 20 to 40 m thick, it comprises more than twelve different volcanic facies. The massive sulphide mineralisation, which is hosted within this unit, is bifurcated into two lenses (B and C) by a combination of steep, layer-parallel faulting and a set of anastomosing, postore andesite sills. The sandstone facies are medium to coarse grained, layered, rich in broken feldspar and quartz crystals, and locally contain some flattened pumice clasts. Some of these sandstones occur immediately below the main B-C ore lenses where they represent the sub-stratum of the massive sulphide mineralisation. Those rocks in the immediate footwall or in the hanging-wall of the B-C ore lenses are strongly chlorite-sericite-quartz altered with disseminated pyrite. Whilst most of the Unit 2 sandstones are rhyolitic or dacitic, some thin, dark grey sandstone beds at the base of the unit have a basaltic-andesite or andesitic composition, and contain both small flattened pumice and some felsic lithic clasts.
In the centre of the eastern part of the deposit, Unit 2 comprises a grey siltstone to sandstone succession of predominantly rhyolitic composition. The lower and middle part of this succession contains tuffaceous siltstones with thin, dark grey to black andesitic mudstone interbeds whereas the top of the succession comprises normal-graded, medium to coarse grained sandstones. Large, 5 to 10 cm pumice clasts occur locally in either sandstone or siltstone. Pumice clasts in Unit 2 are distinguished from those in Unit 1b by their larger size and the abundance of blue quartz crystals. The blue quartz grains have average sizes between 1 to 3 mm and a maximum size of 6 mm.
Up to 20 m thick feldspar-quartz porphyritic rhyolite sills with peperitic contacts are found in the middle of Unit 2 in the centre of the deposit. These sills are locally flow banded, and are similar to those at the top of Unit 1d in the proximal cross-section.
Bedding and textures suggest Unit 2 was deposited in a subaqueous environment below wave base from low density turbidity currents and is interpreted as reworked or re-sedimented felsic pyroclastic detritus because of its crystal rich character (Schlatter, 2007).
Unit 3
• Unit 3 - predominantly an andesite mass-flow breccia succession that was rapidly emplaced in subaqueous conditions following magmatic or
phreatomagmatic pyroclastic eruptions from an adjacent andesite-dacite volcano. It reaches more than 100 m in thickness, but varies laterally. The mass-flow breccia succession has a basal ~30 m thick section that is light-grey to beige-grey in colour and mainly of rhyolitic and lesser dacitic composition. This section is only found in the centre of the mineralised area and is absent from the the proximal and distal parts of the ore deposit. This breccia is composed of distinct graded beds, each of up to 15 m in thickness, containing abundant pink, angular, feldspar-phyric andesitic lithic clasts, 5% strongly chlorite altered pumice clasts and locally minor blue quartz crystals. The felsic breccia is overlain by a middle to upper section that is green-grey, comprising a basaltic-andesite and andesite breccia succession with individual beds that are up to 50 m thick. Pink, angular, feldspar-phyric andesitic lithic, felsic lithic and pumice clasts of two varieties, feldspar-phyric scoria and locally round, blue quartz crystals, occur in this facies. The lower part of this facies is poorly sorted and diffusely stratified, whilst the upper part is normal graded with some grey to black mudstone bed tops. The contact between unit 2 and 3 is sharp, not faulted and sometimes erosional.
• Unit 3R is a fault bounded and isolated, structurally repeated section of Unit 3, straddled by Unit 4. It comprises a basal felsic breccia succession, very similar to that of Unit 3, which only occurs as a small wedge proximal to the deposit, thinning laterally to the east and absent in distal sections. The felsic breccia is overlain by a 10 to 20 m thick andesitic breccia that is massive, poorly sorted, and weakly polymictic to monomictic with massive 5 to 10 m thick beds.
Unit 4
• Unit 4 and 4R mainly comprise rhyolitic tuffaceous sandstones, siltstone and mudstone which host the zinc rich A and D massive sulphide mineralisation lenses. Both Unit 4 and 4R are characterised by 1 to 10 cm rhyolitic pumice clasts containing abundant large, round, blue quartz phenocrysts that are either embayed or shattered. Most pumice clasts are coarsely (up to 4 mm) quartz-porphyritic, with less abundant pumice containing smaller quartz but abundant albite feldspar phenocrysts. The large pumice clasts occur as two distinct tuffaceous plane bedded to massive siltstone beds, which, proximal to mineralisation, are separated by a fine grained coherent and peperitic rhyolite that is absent further from ore. The A and D mineralised lenses occur in the stratigraphic intervals containing the large pumice clasts. Adjacent to the ore lenses, strong hydrothermal chlorite-garnet alteration has reduced the large pumice clasts to chlorite patches with large round quartz grains. Whilst volcaniclastic rocks of this unit are dominantly felsic, several thin basaltic-andesite and andesitic sandstone intercalations are present. The unit is intruded by andesite sills, the thickest of which are located in the immediate hanging-wall of the A ore lens, as well as four texturally distinct rhyolite sills, two of which have peperitic contacts. This succession comprises a lower suite of mudstone and normal-graded tuffaceous sandstone to siltstone in the mineralised lens area, which is interpreted to have been deposited by low density turbidity currents in a subaqueous environment below wave base. They are overlain by massive weakly polymict breccia which is coarser, and was probably emplaced by high density turbidity currents in deep subaqueous conditions. Unit 4R is structurally separated and isolated from the main Unit 4.
Hanging-wall Complex
• Unit 5 - comprises thick, coherent, rhyolite bodies and subordinate volcaniclastic rocks. The coherent rocks are characterised by local rounded, up to 5 mm diameter blue quartz grains. The contact with Unit 4 and 4R is sharp and not faulted. The hanging-wall complex (Unit 5) comprises two main varieties of rhyolite, one of which is thick and contains albite and quartz phenocrysts, whilst the second is relatively thin and contains plagioclase, quartz and K feldspar phenocrysts. The dominant coherent rhyolite bodies are interpreted to be sills, although occasionally some thin beds of volcaniclastic rocks are recognised. The contacts of the thick sills are frequently peperitic. Sericite and chlorite in some of the sills suggest that at least some parts were exposed to weak hydrothermal alteration. Therefore the sills of Unit 5 are interpreted to have been emplaced during or after the waning stages of hydrothermal alteration.
Post-ore and Post-alteration Intrusions
• Unit 6 - predominantly sills, including felsic, andesitic and late high Mg-Cr-Ni basalt. Two late rhyolitic sill populations have been
recognised, a dark-grey to beige-grey, massive aphantic, non-peperitic variety and a grey-beige to grey-yellow, strongly quartz-feldspar porphyritic composition that are up to 20 m thick. The andesite sills are dark-green, massive, have a granular texture and are rich in chlorite and carbonate. They are up to 50 m thick, have chilled margins and have intruded various levels of the sequence and the ore lenses. The basaltic dykes cut the andesitic sills, are only a few metres thick, and are soft with a greenish-yellowish colour and a 'soapy' appearance, but have not been affected by hydrothermal alteration related to the main mineralisation.
Lithogeochemistry shows the thick andesite and dacite breccias, and onerlying rhyolites of the Lower Footwall sequence lie on a high-TiO2 fractionation trend. In contrast, rocks of the Upper Footwall, the Host Unit to the B-C massive sulphide lenses and most of the felsic rocks higher in the stratigraphy belong to a low-TiO2 trend. This change corresponds closely to a change from an early andesite-dacite-rhyolite shield volcano to a later rhyolitic cryptodome-sill-tuff volcano. The B-C ore horizon lies between calc-alkaline to transitional, felsic volcanic rocks of the Host Unit and tholeiitic, basaltic-andesitic and andesitic juvenile mass-flow deposits of Unit 3 that were derived from a different and relatively distal volcanic centre (Schlatter et al., 2007).
Alteration - Most rocks within and surrounding the Petikn&numl;s South deposit are only weakly to moderately altered, but still retain relatively well preserved primary volcanic textures. The most altered rocks belong to the dacitic pumice breccia of Unit 1b on the northern limb of an F2 anticline to the NE of the main B-C lenses. Rhyolites of the upper footwall directly below the main B-C ore lenses are only weakly altered. These rocks have undergone weak sericite and carbonate alteration including the feldspar phenocrysts. It is suggested that these footwall rhyolites have been intruded as sills or domes below the main ore lenses after the ore had formed and at a time when hydrothermal alteration had virtually ceased (Schlatter et al., 2006). Alternatively, if they are coherent, they may not be as permeable as pumiceous and other tuffaceous members of the succession, thus inhibiting the ingress of hydrothermal fluids.
Rocks in the immediate footwall to the A and D ore lenses, and some sections of the footwall to the B-C ore lens are strongly altered (Schlatter et al., 2006) to chlorite-sericite schist with variable amounts of garnet, quartz, carbonate and porphyroblastic feldspar, obliterating the primary volcanic textures. Six alteration types have been recognised: i). chlorite-sericite-garnetfeldspar-quartz; ii). chlorite-sericite-feldspar-quartz; iii). chlorite-sericite-garnet-quartz; iv). chlorite-sericite-quartz; v). chlorite; and vi). sericitequartz. The feldspar porphyroblasts within these alteration assemblages frequently have a complex mineralogy composed of domains of plagioclase, K feldspar and quartz with a sericitic rim. The most intensely altered rocks comprised strongly sulphidic chlorite-sericite-quartz schists with garnet and plagioclase porphyroblasts that occur directly below and lateral to the A ore lens, and chlorite-sericite-quartz schists with plagioclase porphyroblasts immediately below the eastern edge of the B-C ore lens (Schlatter et al., 2007).
The D-ore lens is surrounded, in both the footwall and hanging wall, by an envelope of garnet porphyroblasts with associated magnetite and sulphide stringers within a chloritic matrix. As such, this ore lens is interpreted to have formed by replacement (Schlatter et al., 2007), although, alternatively, Jonsson et al. (1995 ) suggest it might represent a faulted segment of the A ore lens. In the central footwall below the B-C ore lens, a chlorite-sericite-garnet-quartz alteration zone contains massive sulphide-stringers up to 110 cm thick (Schlatter et al., 2007).
Thin bands of carbonate alteration occurs locally throughout the mine sequence at Petiknä̈s South, being most intense in the volcaniclastic rocks of Units 3 and 3R and Units 4 and 4R but only weak in other units. Epidote is the result of saussuritization of feldspars and generally is most intense immediately below the ore lenses (Schlatter et al., 2007).
Mineralisation - the 4 main ore lenses of the Petiknä̈s South deposit are generally concordant with bedding, dip vertically, strike NW and plunge at ~60°E. The lenses are located above the SSE dipping Petiknä̈s Main Fault Zonewhich truncates mineralisation at depth. The largest and stratigraphically lowest B-C lenses are hosted in Unit 2, whilst the smaller A and D lenses are within Unit 4, higher in the sequence. A lens is ~100 m south of B lens, whilst the minor D lens is ~30 m north of A lens.
To the west the B-C ore is a single body, but is split, fork-like, into separate lenses to the east by an andesite sill which has sharp contacts and fine grained, chilled margins. The ore lenses are elongated parallel to the steep tectonic lineation. The main B-C ore lens persisted for ~400 m down-dip, 250 m along strike and was up to 30 m thick. The D and A-ore lenses have dimensions of ~100 m down-dip, 50 to 70 m along strike and are between 10 and 20 m thick.
The B-C ore lens was a single massive mineralised body prior to emplacement of the andesite sills, and graded laterally into several thinner ore lenses (C, C’, and B'), intercalated with weakly sulphide impregnated clastic rocks. The contact of the B-C ore lens with underlying and overlying clastic rocks is sharp, although those of the footwall are moderately sulphide impregnated, whilst the overlying clastic rocks only have weak sulphide disseminations. The massive sulphides of A lens are flanked by a 2 to 4 m thick gradational zone of strong sulphide impregnation. The semi-massive mineralisation of D lens is similarly flanked by strong sulphide impregnation with a gradational transition (Schlatter et al., 2007).
The principal sulphides at Petiknä̈s South are pyrite, sphalerite, pyrrhotite, chalcopyrite, galena and arsenopyrite with minor tetrahedrite, bournonite, gudmundite, amalgam and native bismuth (Jonsson et al. 1995), in gangue of quartz, carbonate, chlorite, sericite and minor epidote (Schlatter et al., 2007).
The B-C ore lenses are composed of massive yellow-brown to dark brown, fine grained, pyrite-rich mineralisation. The ore comprises abundant euhedral metamorphic pyrite crystals with variable interstitial brown sphalerite, lesser chalcopyrite and galena, with some faint banding resulting from grain size variation between euhedral pyrite and contrasting amounts of sphalerite. Brown ores are rich in sphalerite whilst yellow ores are rich in pyrite and chalcopyrite. Euhderal pyrite crystals, which may be up to 50% of these ores, are from 0.1 to 1 mm and often broken. Arsenopyrite is present as needle shaped euhedral crystals, whilst pyrrhotite, which is generally <5%, occurs as ~0.5 mm subhedral grains, but rarely pyrrhotite may comprises 65% of the ore.
The D and A lens mineralisation is very fine grained, red-brown and less massive than the B-C ores. Pyrite and arsenopyrite are found as 0.2 to 1 mm euhedral crystals whilst pyrrhotite occurs as ~0.2 mm subhedral grains. The D and A ores are also rich in interstitial sphalerite and galena. Arsenopyrite and sulphosalts are subordinate and chalcopyrite is absent (Allen and Svenson, 2000).
Return to Top
Åsen
The Åsen deposit is found on the southern side of an ~4 km long isoclinal syncline that is overturned to the NE and has a core of 'phyllites'. The main fold axes are shallow, striking at ~310°. The deposit comprises a number of well-developed sheets that lie parallel to layering and are restricted to the same stratigraphic level, but are concentrated in small secondary folds on the limb of the main syncline. These drag folds, which control the development of ore, are the product of intense sinistral displacement parallel to the fold axes, which also resulted in a strong lineation. The ore is more copper-rich than that at Udden and Kedträsk, whilst the zinc and sulphur contents are lower. It is also characterised by a high barite content, not seen elsewhere in the Skellefte District (Grip, 1978).
Return to Top
Kedträsk
The Kedträsk deposit is located ~2 km to the ESE of Udden (see below), with very similar mineralisation that is associated with the same phyllite zone. Ore occurs as a number of lenses hosted within a sericite schist, occurring as schlieren (streaks) of massive sulphides. The deposit has a total cumulative strike length of 800 m, a width of 15 m, and persists to a depth of at least 400 m. The ore is composed of massive pyrite and sphalerite with some pyrrhotite, chalcopyrite, galena and arsenopyrite (Grip, 1978).
Return to Top
Udden
The Udden deposit is located at the southeastern end of a phyllite zone that is parallel to and 1.5 km south of the Svansele mineralised zone (see below). The deposit is ~370 m in length, varies from 10 to 35 m in width and persisted to a depth of 350 m. It comprises several parallel lenses of massive sulphide interlayered with disseminated sulphides in a skarn gangue. The ore minerals are pyrite and sphalerite with lesser pyrrhotite, chalcopyrite, galena arsenopyrite and sulphosalts. The skarn-rick disseminations are composed of galena and sulphosalts, and has elevated levels of precious minerals. The presence of skarn minerals indicates the protolith was calcareous. The mineralisation was deposited in a weakly sheared and fractured zone between porphyries and tuffs of contrasting competencies. The deposit strikes at 310° and plunges 67°W (Grip, 1978).
Return to Top
Svansele
The Svansele ores are an easterly continuation of the anticlinorium that hosts the Maurliden deposits (see below) and has a similar geological setting. The deposit comprises massive sulphide mineralisation composed of pyrite and pyrrhotite with minor chalcopyrite and sphalerite, hosted within a 20 to 30 m thick band of sericite schist. The orebody occurs a thin sheet that strikes at 310° with a dip and plunge of 40°SW. It is as a gash vein cutting across the zone of sericite schist, having opened during anticlockwise (sinistral) translation within the shear zone represented by the schist. The small Svansele Väg deposit is 200 m to the east and 100 m lower in the host succession, composed of two parallel sheets of massive pyrite carrying sphalerite and lesser chalcopyrite which plunge at ~30°SW. Both lenses are disposed diagonally across the direction of schistosity as at Svansele.
Return to Top
Norrliden
The Norrliden mineralisation was drilled, and the northern of two zones of mineralisation, Norrliden Norra (North), was discovered by the Swedish Geological Survey in 1968 following drilling of a geophysical electromagnetic anomaly detected in 1958-59. Delineation drilling continued until 1974 with 66 holes having been completed. In 1999, North Atlantic Natural Resources, which in 2004 became a majority owned subsidiary of Lundin Mining, initiated a second drilling program to establish the nature and full extent of the mineralisation (Wheeler, 2006).
The two zones of mineralisation Norrliden Norra and Norrliden Sodra (South) lie within the WNW-ESE trending Malånäset Anticlinorium, a fault offset continuation of the Menträsk Anticlinorium to the west. To the north, this structure is bounded by a broad syncline containing sedimentary and volcanic rocks of the Vargfors Group, while to the south it is overlain by phyllites, also presumably part of the same group. The limbs of these structures host deposits such as, from west to east, Menträsk, Maurliden Västra and Östra (5 km to the NW), Svansele (10 km to the east), Udden, Kedträsk and Åsen (20 km to the SE of Norrliden). Other nearby historic mines and significant sulphide prospects include Bjurfors Gruvfält (a mined out flat lying 185 x 30 x 30 m lens of chalcopyrite-pyrrhotite, 1.5 km to the ESE), Bjurliden and Bjurträskgruvan (5 km along strike to the SE), and Högkulla (2 lenses of zinc rich pyrite and pyrrhotite that yielded 0.114 Mt of massive sulphide) and Skäggträskberge (5 km to the west of Norrliden; Grip, 1978; MRG Metals Ltd ASX Releases, June and August 2017). These primary structures have undulose, near horizontal fold axes. To the north, the interbedded volcanic and sedimentary rocks have been refolded about steep secondary fold axes on the flanks of the primary structure, while other tectonic units have been thrust over them, including a tongue of Revsund Granite. Norrliden lies on the northern limb of the Malånäset Anticlinorium and is controlled by the secondary folds (Grip, 1978).
The Norrliden Nora deposit is hosted by interbedded sedimentary and pyroclastic rocks described as felsites, rhyolites, tuffites and foliated 'greenstones'. The host sequence strikes at 110°, dips at 60 to 80°S, and is intruded by narrow dykes and sills of rhyolite and andesite (Wheeler 2006; Grip 1978). Greenstone predominates in the immediate hanging wall of the highest massive sulphide lens and quartz porphyry in the footwall. The foliated 'greenstone' is characterised by delicate laminations which may be primary bedding features. The andesite dykes, which cross-cut the central part of the deposit, are distinguished by their abundant tabular feldspar phenocrysts (Wheeler, 2006).
All the lithologies have been regionally metamorphosed to lower amphibolite facies and intruded by the ~1.8 Ga Adak (Revsund) granite which has imparted some contact metamorphic effects (Wheeler, 2006).
Only the uppermost point of one of the two closely spaced lens shaped orebodies is exposed at surface, with mineralisation extending to a depth of 300 m before thinning. The deposit comprises a 400 m, east-west trending zone divided into the two orebodies, each of which is composed of numerous lenses of massive to semi-massive sulphide. These are separated by mineralised zones with 30 to 40% sulphide which include stringer, disseminated and semi-massive mineralisation, often characterised by silicification and sericitisation (Wheeler, 2006). The sulphide lenses lie sub-parallel the volcanic stratigraphy, close to, or at the contact between felsic and mafic volcanic rocks in a strongly deformed quartz-sericite rich band (Wheeler, 2006) and dip at 80 to 85°S (Grip, 1978). The massive to semi-massive sulphide lenses comprise banded pyrite with some pyrrhotite, accompanied by fine grained sphalerite ± minor chalcopyrite, with dispersed galena and minor arsenopyrite (Wheeler, 2006).
Norrliden Södra is a prospect located 500 m SW of Norrliden Norra, immediately south of a major WNW-ESE shear zone. It was first identified during the 1930s and appears to be a separate mineral occurrence (MRG Metals Limited, 2017).
Return to Top
Holmtjärn
The significance of the small, but very high grade, Holmtjärn deposit, which is located ~55 km NW of Boliden, is that it was the first to be mined in the Skellefteå Field. It was discovered in 1924 and 366 tonnes of ore were mined in that and the following year. It was exposed as a strongly rust coloured weathered outcrop that was highly enriched in gold and silver, with an oxidised sample assaying 1165 g/t Au and 5542 g/t Ag. The main mineralisation comprised a compact gold-copper-arsenic ore in the hanging wall and a pyrite ore in the footwall. The ore body was 10 m long, up to 3 m wide and continued to a depth of 28 m. A second body of the same size, but much lower grades, occurred 35 m east of the main ore. The upper parts of the unweathered copper-arsenic ore in the main orebody carried ~100 g/ton Au, but increased towards the bottom where a 23 m interval assayed 524 g/t Au and 858 g/t Ag. The gold-copper-arsenic ore was predominantly arsenopyrite brecciated by chalcopyrite. Fine grained native gold occurred in minor amounts. The pyrite ore was dominated by pyrite and chalcopyrite, but also contained native gold and several other sulphides. The wall rock comprises a strongly sericitised quartz porphyry (Johansson Bildström, 2003, Georange Excursion sites in Northern Våsterbotten).
Return to Top
Maurliden
The Maurliden mining area is located in the central part of the Skellefte minining district, in Norsjö municipality, ~45 km WNW of the concentrator in Boliden. The mining area, which covers an area ~8 x 6 km, hosts four deposits two of which have been mined by open pit: Maurliden (or Maurliden West) and Mauliden Östra. The area is predominantly underlain by volcanic rocks with a primarily rhyolitic composition (Allen et al.,1996, Montelius 2005) that belong to the Skellefte Group. All four sulphide deposits and prospects in the Maurliden area are hosted by a quartz-feldspar porphyritic volcanic rock that was deposited as a series of pumiceous mass-flows (Montelius 2005).
The main Maurliden deposit is surrounded by strongly quartz-sericite altered and intense hyalocastic breccias that often host pyrite veins, particularly in the footwall on the northern margin of the deposit. A weakly porphyritic ryholite intrusion is found on the southern side of the orebody. A pyritic mudstone interfingers with the ore and the quartz-feldspar rhyolite on the northern side of the deposit (Albrecht - Boliden, 2018).
Maurliden West is a massive, pyritic sulphide deposit in which zinc is the primary metal. The deposit is tabular, dips vertically and is sub-parallel to lithological banding. The centre of the deposit is composed of massive pyrite with minor bands and streaks of sphalerite, whilst in the upper part of the pit, the margins of the deposit tend to have higher sphalerite grades. Two distinct high grade zinc zones can be distinguished lower in the pit, at the southern and northern margins of the deposit. Chalcopyrite occurs in the upper part of the deposit on its northwestern tip and northeastern end, increasing downwards to where is concentrated between the two high-grade sphalerite zones along the whole length of the deposit. Chalcopyrite is found both as disseminations in massive pyrite and in stringers of pyrite. Silver is closely associated with sphalerite and occurs within tetrahedrite, whilst the bulk of the antimony is in bornite (Bohlin 2009). Gold occurs in electrum (Au-Ag-Hg-alloy), and as micro inclusions and small fracture fillings in pyrite (80%). Electrum also occurs as inclusions in primary arsenopyrite and is intergrown with antimony minerals (Brising, 2016). Gold recoveries are low (Johansson 2003).
Return to Top
Mensträsk or Inre Sjö Mine
Mensträsk lies within the central anticlinal structure that extends to the ESE to Kedtrask. On the northern flank of this structure, volcanic rocks of the Skellefte Group dip shallowly below sedimentary rocks of the Vargfors Group in a gentle syncline. In the south, the adjacent syncline is more intensely deformed, in places isoclinally folded. Cross folding has produced a series of domes. In the Mensträsk area, isoclinal folding has produced steep dips in the ore-bearing northeastern sections, whereas to the south the stratification is flat lying. Late secondary folding has produced drag dolds and a steeply plunging lineation. The ore at Mensträsk occurs as six lenses of massive sulphide distributed over an area of 300 x 70 m, with grade of ~2.2% Zn, 0.5% Cu, 31% S that strike at 130°, dip at 70°NE and plunge at 45°NNW. The are hosted uin coarse grained sedimentary rocks and weathering breccias that lie between the Skellefte Group felsites and Vargfors Group phyllites. Weak sulphide mineralisation is also found within the felsites where they have been brecciated. The main ore is in conglomerates sandwiched by two lithologies of different competencies, namely the older, brittle quartz porphyries and the younger, more ductile phyllites. The conglomerate has been brecciated due to differential deformation. The wall rocks have been altered to an assemblage of sericite, chlorite and calcite, altjough alteration may have preceded the main sulphide mineralisation as clasts of sericite and chlorite schist occur within the massive sulphides. The principal ore minerals are arsenopyrite, followed by pyrite, followed paragenetically by sphalerite, pyrrhotite and chalcopyrite, in places overprinted by a later disseminated pyrite. The mineralisation has a schlieren structure, parallel to the foliation of the enclosing country rock. Bands of more or less pure pyrite alternate with sphalerite, pyrrhotite, chalcopyrite and some sulphosalts (Grip, 1978).
Return to Top
Rakkejaur
The Rakkejaur deposit lies on the north eastern flank of the Näsliden dome which has a core of felsite, keratophyre, quartz-keratophyre and dacite with equivalent pyroclastic rocks. These are overlain in the south by sedimentary rocks that are mainly calcareous and form a transition from the volcanic sequence to the overlying 'Phyllite Group'. These transition sedimentary rocks thin to the north, or are unevenly developed, but re-occur and thicken around Rakkejaur to the NE. The dome is folded about horizontal fold axes to form an anticlinorium, which passes to the NE and SW into synclinoria that embrace Phyllite Group sedimentary rocks. Subsequent deformation associated with intrusion of mafic and granitic rocks has resulted in folding about near vertical axes, shearing, and well developed lineations (Grip, 1978).
Rakkejaur is one of the largest mineralised systems in the Skellefteå field, with plan dimensions of ~550 x 65 m, extending to a depth of at least 320 m, with a stock-like form. It occurs within the transition sequence described above, with footwall rocks to the west comprising quartz porphyry altered to sericite schist, with local strongly developed schistosity. These are followed by a calcareous weathering breccia and a polymict conglomerate with occasional boulder sized granite clasts, then by banded phyllite, which in places lies directly on the uneven surface of the altered quartz porphyry. Where mineralised, the conglomerate and carbonate breccia are also sericitised, with occasional accompanying chlorite. The orebody follows the phyllite contact and has a vertical dip. It is bounded to the north by a drag fold with an axis plunging at 80°NNW that formed during sinistral rotation (Grip, 1978).
Mineralisation occurs as low grade pyrite, which is rarely massive, containing variable zinc, copper and arsenic, including a few lenses of arsenopyrite and of copper ore. The arsenopyrite lenses, which formed during the initial stages of mineralisation, are always fined grained and dense, and are best developed in the uppermost parts of the deposit, thinning downwards, with only a few thin schlieren remaining at depth. The main stage produced pyrite ore which generally has a sharp contact with the country rock, except to the south where it either thins or passes into disseminated mineralisation. It contains variable sphalerite, some arsenopyrite and insignificant galena as schlieren, as well as local fine grained disseminations of chalcopyrite and minor pyrrhotite. The pyrite ore is brecciated and replaces the country rock, particularly those that are coarse grained, including the conglomerates and calcareous breccia, but also the schists and phyllites. Replacement is accompanied by silicification. The third stage produced copper ore which occurs in irregular NW-SE elongated bodies within brecciated pyrite ore and country rocks. The breccia is recemented by chalcopyrite and pyrite with occasional associated pyrrhotite and minor sphalerite, arsenopyrite and tetrahedrite. This stage of mineralisation accompanied continued sinistral rotation which produced gash veining. A forth stage gave rise to the development of abundant carbonate dykes containing chalcopyrite and sulphosalts such as boulangerite and tetrahedrite (Grip, 1978).
Return to Top
Näsliden
The Näsliden deposit is located on the southwestern flank of the Näsliden dome (described above) where the volcanic felsites of the footwall 'Volcanic Group' are overlain by several hundred metres of a transition sequence to the overlying 'Phyllite Group'. This transition comprises phyllites, sandstones and conglomerates, all of which are calcareous as is the 'Phyllite Group'. The mineralised hosts have been altered to sericite-chlorite facies schists. Mineralisation is hosted by several horizons within the transition sequence, mostly replacing graphitic phyllites, as well as calcareous sections in the lower part of the overlying Phyllite Group. Remnants of graphitic phyllite are evident within the massive sulphides, taken to support the interpreted replacement by sulphides. The southwestern flank of the regional anticlinorium in the Näsliden district is overprinted by a series of drag folds with axial plunges of 70° at an azimuth of 300°. These folds controlled the development of shear zones oriented at 340 and 353°, both of which dipped at ~70°W within the transition sequence. During the sinistral rotation on these structures, sulphides were deposited within the 340° shear zone, while repeated movement occurred on the 353° planes. The calcareous lithologies were the favoured sites of deposition (Grip, 1978).
The Näsliden Södra section of the deposit comprises three en echelon 'ruler shaped' bodies that are each up to 160 m long and 20 m wide, persisting to depths of at least 350 m. They are composed of massive sulphide that comprises pyrite, sphalerite, pyrrhotite, arsenopyrite, galena, sulphosalts and tetrahedrite, which pass outwards into a halo of disseminated or with the same composition (Grip, 1978).
Näsliden Nora comprises five lenses within a range of horizons directly above the felsites, the largest and smallest of which are 300 and 100 m in length. The lenses are also structurally controlled and the mineral assemblage similar, but with considerable quantities of magnetite (Grip, 1978).
Return to Top
Storliden
Storliden is ~80 km WNW of Boliden. It contrasts to other massive sulphide ores in the Skellefte District, in that the ore is very coarse-grained, composed of sphalerite, chalcopyrite, pyrrhotite and calcite, and contains almost no pyrite. It occurs as a single, flat-lying, stratabound lens within the uppermost part of a felsic, pumiceous, crystal-rich unit and has a distinct, asymmetric alteration system, which consists of six metamorphosed alteration facies. The innermost zone comprises intensely silicified rocks and is surrounded by a distinct and persistent layer of spinel-orthoamphibole-humite. This is succeeded outward by spotted cordierite-anthophyllite, cordierite-phlogopite and biotite-muscovite assemblages. Skarn alteration is common and is mainly formed where the inner alteration zones overprint earlier diagenetic pods of calcitic alteration. Relicts of silicified volcaniclastic rocks within the ore lens suggest that the ore formed by replacement of the volcaniclastic sedimentary rocks (Imana, Allen and Barrett, 2005).
Geochemical studies have shown that mineralisation related alteration led to strong Na depletion and significant addition of Si, Mg and Fe towards the orebody. These alteration halos extend asymmetrically for at least 100 m both along strike and above the orebody, and for 10 to 15 metres below it. The quantity of Mg added varies from ~40 wt.% near the thickest part of the ore to <5 wt.% distal to ore. The added Mg in the core of the system after subsequent metamorphism as assemblages that form distinct zones of orthoamphibole, spinel and cordierite. These metamorphic assemblages envelope the southwestern part of the ore lens. Similarly, intense silicification is evident in rocks within and adjacent to massive sulphides, with typical Si gains of >40 wt.%. Silicification is seen in most synvolcanic sills and some pumiceous crystal rich deposits, but in contrast to the ore-related silica addition, this alteration type has discrete Si and Na gains and very low metal values (Imana, Allen and Barrett, 2005).
Return to Top
Adak Fältet
The Adak Fältet (or Adak Field) deposits occur towards the northwestern extremity of the Skellefteå field, ~20 km NW of Storliden. It comprises five deposits, Lindskold, Brännmyran, Rudtjebäcken, Adak and Karlsson distributed around the margin of the small, ~2 to 3 km diameter Adak structural dome. These deposits represent two ore styles. The first three comprise massive stratabound mineralisation, whilst the remaining two contain breccia-dissemination ores. The core of the dome is occupied by a micaceous schist, which passes progressively upward and outward into the ore-bearing unit, known as the Lower Banded Series, composed of tuffaceous volcanic and sedimentary rocks that are exposed over a strike length of ~4 km around the dome; then the Andesite Formation with limestone interbeds; overlain by the Upper Banded Series, a tuffite unit similar to the Lower Banded Series; and the uppermost Grey biotite-hornblende tuffite formation, including graphitic phyllite beds. The dome is intruded on three sides by younger red microcline granites. The mica schist and Lower Banded Series dip at 15 to 40° outwards.
The Rudtjebäcken deposit forms a more or less concordant sheet that is up to 1000 m long and 360 m wide. Locally it is >10 m, but normally ~3 to 4 m thick, striking at 315 to 300° with a dip of 15 to 25°NE. It occurs within a separate crossfold in the eastern part of the dome. In its upper part the deposit is planar, but at depth it becomes folded. In its upper and western sections the plunge is near horizontal, but to the SE and at depth it steepens to 20°SE, forming an anticlinal saddle. The ore is weakest in the deposit's upper sections, fading out into low grade disseminations. The principal sulphide minerals is pyrite, accompanied by pyrrhotite, sphalerite and chalcopyrite. Pyrite is conspicuously coarse grained with crystals that are >1 cm in diameter (Grip, 1978).
At the similar Lindsköld deposit 3 km to the west, the stratabound mineralisation grades upward from massive to progressively more disseminated sulphides. Arsenopyrite is abundant in the massive sulphides, forming dense masses with chalcopyrite and pyrrhotite. Arsenopyrite also sometimes occurs as fine grained massive bodies, brecciated by chalcopyrite. Gangue minerals in the more massive sulphides include quartz and various skarn minerals, diopside, hornlende and anorthitic plagioclase. Outside of the main orebodies, but still within the same horizon, schlieren of sphalerite, pyrrhotite and some chalcopyrite and galena occur with abundant native bismuth. A late phase of mineralisation produced calcite veins containing yellow sphalerite with varying amounts of native arsenic, native sulphur, dyscrasite, proustite, tetrahedrite, cobalt and nickel arsenides, calcite, quartz and zeolites. The Lindsköld mineralisation, which can be traced around the dome to Rudtjebäcken, is more copper rich than the other deposits of the dome. The footwall rocks to the sulphide mineralisation at Lindsköld, which are strongly silicified to quartzites, have associated developments of cordierite, cummingtonite, andalusite, biotite and chlorite. In contrast, the hanging wall Lower Banded Series with calc-silicate bands, have not apparently been affected by the ore fluids to the same degree (Grip, 1978).
The Adak deposit is located <1 km to the south of Lindsköld. It is composed of a large number of small orebodies that are elongated and plunge gently towards an azimuth of 230°, related to a series of fracture and to a lesser extent the primary banding of the host mica schist. The most significant fracture directions strike at 45° and 75°, dipping at 50°NW and 10°SE respectively. Mineralisation is richest within and adjacent to the fractures, decreasing in intensity outwards, but following the foliation in schists and breccia zones in quartzite. The dominant sulphide minerals are chalcopyrite and pyrrhotite, with minor sphalerite. Cobalt bearing arsenopyrite is widespread. Ore grade at Adak was ~1.9% Cu (Grip, 1978).
The Brännmyran deposit is located ~2 km SE of Adak and 2 km SW of Rudtjebäcken. It comprises two overlapping sheets of mineralisation at slightly different horizons, and a third body a little to the east. All are distributed along the crest and flank of a slight anticline. Mineralisation is composed of pyrtite, chalcopyrite, arsenopyrite and pyrrhotite, with some galena in crosscutting calcite veins. Although most of the ore is fine-gained pyrite and arsenopyrite form crystals that are up 1 cm across. The ore contained ~1.7% Cu (Grip, 1978).
Return to Top
Kristineberg (#Location: 65° 3' 53"N, 20° 33' 48"E)
The Kristineberg deposit is located towards the western extremity of the Skellefteå mining field and is the largest of a cluster of deposits in the surrounding district. It occurs within a 2 to 3 km thick sequence of felsic to intermediate and minor mafic metavolcanic rocks which belong to the Skellefte Group. These rocks are intruded by syn-volcanic granitoids (granodiorite to tonalite) of the 1907±13 Ma (Bergström et al., 1999) Viterliden Intrusive Complex of the regional Jörn G1 Phase. Both the volcanic sequence and the intrusives are overlain by meta-sediments of the Vargfors Group. Younger Granites of the Revsund Suite intrude all of the above rocks.
In the Kristineberg deposit area, a variety felsic rocks and schists are mapped within the Skellefte Group. Whilst massive, coherent feldspar-phyric rhyolites can be recognised, many of the rocks in the vicinity of the deposit are strongly altered and metamorphosed, and can only be described as schists containing various proportions of muscovite, quartz, chlorite, phlogopite, cordierite, andalusite, pyrite and magnetite (Årebäck et al., 2005). Geochemically, most of the primary volcanic rocks comprise a more or less continuous range of compositions from andesite through dacite to rhyolite and their subvolcanic equivalents (Barrett et al., 2005). The overlying fine-grained turbiditic metasediments of the Vargfors Group comprise a lower several hundred metre thick unit characterised by graphite and pyrrhotite-bearing black shales. This is followed by a much thicker succession of fine grained turbiditic metasediments (Vivallo and Willdén 1988; Weihed et al., 1992; Allen et al., 1996).
Mineralisation within the district is found in two stratigraphic positions, both near the contact with the overlying Vargfors Group, and near the base of the felsic volcanic pile, close to the Viterliden Intrusive Complex (Du Rietz 1953; Edelman 1967; Grip 1978; Vivallo and Willdeń 1988). The upper of these, the 'Rävliden Horizon', hosts the Rävliden, Rävliden North, Rävlidmyran and Hornträsk deposits, a stratigraphic position typical of most of the Skellefte district VHMS deposits (Allen et al., 1996; Årebäck et al., 2005), whilst the lower, the 'Kristineberg Horizon' includes the Kristineberg and Kimheden deposits that are lower in the Skellefte Group than most other such mineralisation (Skyttä et al., 2012).
The supracrustal rocks of the Kristineberg area define the regional-scale 'Kristineberg Antiform' which encloses two individual second-order west-plunging antiforms, the Southern and Northern antiforms. These structures are separated by either a synform (Årebäck et al., 2005) or a large-scale shear zone (Malehmir et al., 2007; Dehghannejad et al., 2010) which cuts both the Skellefte and Vargfors groups. These two larger
structures host a series of parasitic kilometre-scale antiforms and synforms, each also having a westerly plunge. Strain was heterogeneously distributed within the Kristineberg district, strongly partitioned into curviplanar east-west to NE-SW trending high-strain zones separated by low-strain tectonic lenses (Skyttä et al., 2010).
The Kristineberg deposit comprises multiple ore zones, occurring as two separate, generally east-west trending, parellel strings or arms, with one larger zone (Zone-A) at the eastern extremity of northern arm. These zones are distributed over an ~4.5 km east-west interval and include: i). A-Zone massive sulphide lens which has historically been the largest and principal orebody containing Cu, Zn and Au mineralisation. It has a strike length of ~1400 m, and extends from surface to a depth of ~1200 m and has a moderate dip to the south; ii). B-Zone massive sulphide Cu-Au lens, located 100 to 150 m structurally above the A-Zone, extending from surface to ~1000 m depth; iii). Einarsson or E-Zone, which occurs in the vicinity of the B-Zone at ~1000 m depth, and comprises mainly Au-Cu rich veins and heavily disseminated sulphides, together with massive sulphide lenses in a quartzite-rich host in contrast to the chlorite schist in which almost all of the other zones are found; iv). J-Zone Zn-Cu-Au; v). K-Zone Zn; vi). L-Zone, which is an ~2 km long, gently west-plunging irregular 'rod-shaped' body of Zn-Cu-Au mineralisation; vii). M-Zone Zn-Cu; viii). Raimo and Tommy Zn-Cu mineralisation; and ix). Ag-Zone Ag-Pb, which like the E-Zone, is hosted by 'quartzites'. The southern arm consists of the B-, E-, J-, K-, M- and Ag-Zones as well as the Raimo and Tommy mineralisation, whilst the northern only includes the A- and L-Zones (Boliden, 2019). The southern arm forms a composite 'rod-shaped' mass parallel to to the northern arm over a strike length of ~1.5 km, with the M-Zone a parallel 'sub-arm' immediately to its north.
On a district scale the Kristineberg deposit is flanked by two major felsic rock units, the massive coherent feldspar-phyric rhyolite to the south and the Viterliden Intrusive Complex porphyry to the north. The ore zones all lie within a package of hydrothermally altered, dominantly felsic volcanic rocks that have been deformed and metamorphosed to schists which contain varying proportions of quartz, muscovite, chlorite, phlogopite, pyrite, cordierite and andalusite. The most intense alteration occurs within 5 to 10 m of the ore lenses. The stratigraphic younging within the deposit area is uncertain as primary bedding and volcanic textures have been obliterated by the strong alteration, folding and shearing. In the vicinity of the ore lenses, hydrothermal alteration has formed both 'chlorite schists' and 'quartzites'. The 'chlorite schists' contain Mg-rich assemblages of Mg-chlorite, cordierite, phlogopite and locally talc, with variable muscovite, quartz, biotite, andalusite and magnetite. The 'quartzites' are composed of quartz-muscovite-andalusite assemblages. Both suites commonly contain disseminated pyrite. The 'chlorite schist' hosted mineralisation generally contains semi-massive to massive sulphides comprising variable chalcopyrite, sphalerite and galena, with minor silver and gold. The 'chlorite schists' occur as lenses within the 'quartzites' which are interpreted to be highly altered rhyolitic to dacitic rocks. The 'remobilised' Ag-Pb mineralisation within within silicified cordierite and chloritic quartzites is characterised of the dominant silver bearing mineral freibergite [(Ag,Cu,Fe)12(Sb,As)4S13], and minor hessite (Ag2Te) often present. High silver grades are often present in narrow zones associated with galena veins or fracture fillings (Boliden, 2019; Barrett and MacLean, 2000). The volcanic sequence within an area of some 50 km2 surrounding the Kristineberg-Rävliden cluster of deposits has been influenced by extensive sodic depletion (albite destructive alteration) resulting in the formation of chlorite and muscovite and consequent strong co-enrichment in magnesium and potassium. Kristineberg is enveloped by a 2 km diameter halo of quartz-chlorite alteration and a distal but coherent pyrite-quartz-muscovite zone extending as far out as 4 km. Chlorite and talc in the immediate Kristineberg deposit area is notably magnesium-rich and contains anomalous F, Ba, Zn and Mn. High fluorine is also present in coexisting muscovite and phlogopite (Hannington et al., 2003).
Return to Top
Rävliden North
The Rävliden North deposit is ~5 km to the west of Kristenberg and ~1 km WNW of the historic Rävliden mine, hosted within the upper sections of the Skellefte Group, close to the contact with the overlying Vargfors Group (see the Kristineberg description above). It is interpreted to comprise a sub-vertical to steeply south dipping, ~5 to 25 m wide, 900 m long down plunge and ~150 m wide mineralised lens, or cluster of lenses. Mineralisation occurs as:
• Massive to semi-massive sphalerite-dominated mineralisation, with lesser galena and pyrrhotite, and local porphyroblasts of pyrite which are aligned, giving the mineralisation a crudely banded appearance and suggesting ductile deformation. Local zones of massive pyrrhotite are also evident within the massive sulphides. This mineralisation is found near the transition from rhyolitic rocks to stratigraphically overlying graphitic shale, marking the transition from the Skellefte to the Vargfors group. Mineralisation occurs as lenses separated by weakly mineralised to barren rocks, and is most commonly associated with tremolite skarn, talc schist, chlorite schist and dolomitic marble at this stratigraphic transition. Both the tremolite skarn and dolomitic marble also contains pyrrhotite and pyrite, whilst chalcopyrite and arsenopyrite are locally present in the marble. The massive to semi-massive sulphides locally contain tension gashes infilled by chalcopyrite and pyrrhotite; mineralisation formed in pressure-shadows near host rock fragments; and ball-ore (or 'durchbewegung') textures reflecting ductile deformation of pre-existing sulphide mineralisation (e.g., Vokes 1973; Johansson, 2017; Boliden 2019).
• Stratigraphically and structurally below the sphalerite mineralisation, sulphide-bearing stringers, veins and breccia Cu>Zn mineralisation. Large parts of this zone, particularly in dacitic intrusions, are dominated by pyrrhotite and pyrite, with only carry traces of sphalerite and chalcopyrite. However, an ~10 to 30 m thick segment of this zone that is proximal to, and stratigraphically directly below the main massive sulphide cluster of lenses, carries substantially elevated chalcopyrite and minor idiomorphic arsenopyrite crystals and sphalerite, with grades ranging from 2 to 3 % Cu over several metres, and commonly network-style breccias. These zones are within intensely silicified footwall rhyolite in which sulphides are hosted by hydraulic breccias and strongly to intensely chlorite-altered footwall rhyolite. In schistose sections of the latter, the sulphides are subtly but strongly disseminated. In contrast, the more common quartz-sericite altered footwall rhyolite appears to be less endowed in metals, even though it commonly carries minute crystals of pyrite. Galena is rare but dies occur in some hydrothermally-altered rhyolite, altered volcaniclastic rhyolite and tremolite skarn. With the exception of the poorly-mineralised dacitic intrusion, there is a gradational decrease of mineralisation stratigraphically below the massive sulphides (Johansson, 2017; Boliden 2019).
• Local, vein- and/or fault-hosted silver-rich mineralisation in the stratigraphic hanging wall, stratigraphically above the massive sulphide mineralisation, hosted within the Vargfors Group. It locally comprises faulted and/or sheared massive sulphides compositionally similar to the stratigraphically underlying main massive to semi-massive sulphide mineralisation, but with significantly more freibergite. Parts of the hanging wall mineralisation are entirely dominated by sulphides and sulphosalts of silver, including pyrargyrite (Ag3SbS3), pyrostilpnite (also 3), argentopyrite (AgFe2S4), sternbergite (AgFe2S3) and stephanite (Ag5SbS4). These silver sulphides and sulphosalts occur in structurally late settings, which together with their temperature stabilities suggest a late origin. As the silver-bearing minerals in the main massive to semi-massive sulphide mineralisation and the two hanging wall styles contain the same metals, those in the hanging wall are interpreted to have formed by late-stage remobilisation of the main massive sulphides, which facilitated upgrading of the Ag-content and development of this paragenetically late mineralisation. Alternatively, they may represent a late stage of silver-rich hydrothermal activity, overprinting both the massive sulphides and hanging wall (Johansson, 2017; Boliden 2019).
Return to Top
Rävlidmyran
The Rävlidmyran deposit is ~5 km WNW of Kristenberg and 1.5 km north of Rävliden North. It is hosted within the transition sequence between the Volcanic and Phyllite groups of the earlier literature, or between the volcanic rocks of Skellefte Group, and the contact with the overlying Vargfors Group sedimentary rocks. The country rock in the deposit area comprises black or grey phyllite, sericite schist, chlorite-talc phyllite and impure dolostone or limestone. The carbonates are sometimes altered to a skarn mineralogy. The principal hosts to ore are talc-chlorite phyllite or impure dolostone.
Two parallel ore zones that are some 200 m apart have been delineated at Rävlidmyran. The southern zone embraces four orebodies, each of which is 40 to 160 m long and up to 4 m wide. The northern zone comprises the single Sture orebody that is 350 m long and has an average thickness of 20 m. All of the ore lenses are east-west striking, vertically dipping and plunge at 25 to 30°W. The Sture orebody is composed of zones of massive polymetallic sulphides and of disseminated copper ore. The former comprises pyrite, chalcopyrite and galena in varying proportions in the stratigraphic hanging wall, while the disseminated copper chalcopyrite mineralisation is mostly in the footwall. Pyrrhotite is unevenly distributed across the orebody. Zinc-rich sections exhibit well developed 2 to 10 cm thick alternating bands of pyrite and sphalerite. Mineralisation is interpreted to have been affected by drag folding, local brecciation and intense shearing developed during deformation of the host dolostones (Grip, 1978). Grip (1978) describes the carbonate rocks as having been replaced to varying degrees, as have various other country rocks with the hydrothermal system being capped by the overlying phyllites.
Return to Top
Hornträsk
The Horntrask deposit was mined between 1987 and 1991 and is located ~3 km NW of Kristineberg. It is the smallest of a cluster of deposits, including Rävliden and Rävlidmyran. For details of the district geology and structure see the Kristineberg description above. The Kristineberg district is characterised by two west- to SW-plunging antiforms separated by a shear zone. The shear zone divides the district into a domain of dominantly coaxial deformation in the north, and a transpressional domain to the south (Skyttä et al., 2013). The deposits of Hornträsk and Rävlidmyran are located on the northern limb of the southern antiform, and are sub-horizontal (Bauer et al., 2014). The structure of the Hornträsk deposit is described as having been subjected to isoclinal folding (Andersson, 1996) with an axial strike of 60° and a steep dip that plunges at 25°SW, sitting on the northwestern limb of the composite Kristineberg Anticline. Hornträsk, like Rävliden and Rävlidmyran, is located within a zone of
transition between the volcanic and sedimentary rocks of the Skellefte and Vargfors groups respectively (Friedrichs 2017).
The hanging wall at Hornträsk has been divided into two main units, i). a rhyolitic to dacitic siltstone, containing siliceous and carbonate clasts and blue quartz phenocrysts; and ii). andesitic turbidite and sandstones, as well as a third thin basaltic siltstone. The footwall is also composed of volcaniclastic rocks, but in contrast to the hanging wall, rocks are only of rhyolitic origin. Grain sizes vary from ~2 mm, up to 50 mm clasts, and the rock has a weakly quartz-phyric texture, which with a local relict <1 mm crystalline groundmass, suggest the footwall most likely constituted coherent rhyolitic lavas and/or sub-volcanic intrusions. Mineralisation is located in the transition between the hanging wall and footwall packages, in a carbonate rich unit that typically has a thickness of around 2 m (Friedrichs 2017).
The footwall is dominated by weak to strong sericitic alteration, with chloritic alteration appearing in the top towards the mineralised horizon. Alteration in the hanging wall rhyolitic to dacitic siltstone is also dominated by sericite and chlorite, but is mostly of low intensity except in proximity to the mineralised horizon at the base of the hanging wall succession. Within the andesitic hanging wall unit, alteration ranges from weak chlorite to skarn (dominantly tremolite) in close contact with mineralisation. More intense alteration commences a few metres above the mineralisation with increasing chlorite that grades into the skarn alteration accompanied by the development of anorthite. Skarn is the dominant alteration type within the main zones of mineralisation, with tremolite forms crystals that are up to 2 cm long. Carbonate units within the sequence adjacent to ore can be recrystallised to marble layers with thicknesses of up to 5 cm (Friedrichs 2017).
Return to Top
Kimheden
The Kimheden deposit comprised of three, thin, elongate, narrow lenses arranged in an en echelon pattern with a strike of 65°, dipping 85°S. The lenses are hosted by chlorite rich schists that are alteration products of volcanic rocks. Ore occurs as both massive pyrite with a low copper content and as disseminated copper with insignificant pyrite. A number of minor pyrite-pyrrhotite occurrences are found along strike to the east. The average grade of the combined mineralisation was 1.2% Cu, 0.1% Zn, 6 g/t Ag, 0.3 g/t Au, 21% Zn. The deposit was mined until 2004 (Grip, 1978).
Return to Top
Åkerberg
The Åkerberg deposit is located ~35 km NW of Skellefteå, just to the north of the main Skellefteå Ore District, and like Björkdal to its south, is a gold-only deposit. Both deposits are to the east of the Vidsel-Röjnoret Shear System (VRSS). The area surrounding the Åkerberg deposit is dominated by metasedimentary rocks of the Bothnian Supergroup, with metavolcanic rocks of the Skellefte Group conspicuous by their absence. The Bothnian Supergroup sedimentary succession at Åkerberg is interpreted to overlie the volcanic rocks of the Skellefte Group. A granodiorite intrusion of the Younger Revsund 1.8 Ga Skellefte Suite and pegmatite bodies occur in the proximity of the deposit, emplaced into the gabbro which hosts the gold ore. More distal Older Revsund granitoids occur to the east. All supracrustal rocks, and partly the granitoids, have been subjected to a regional metamorphic event.
The host gabbro occurs as an ~10 km long, north-south elongated layered intrusion. The magmatic layering, often dipping at ~50°, but locally flatter, is relatively diffuse, defined by variations in mineralogical composition and by the parallel orientation of plagioclase. Geophysical data suggest that the gabbro is shallow sheet, and has been mapped as an early orogenic intrusion, i.e., emplaced between 1.89 and 1.87 Ga. Grain size varies, ranging from a coarse-grained, feldspar-rich rock of dioritic composition to a medium-grained, dark-green, quite homogeneous gabbroic variety containing clusters of biotite. In the ore zone, the gabbro tends to be richer in quartz. Some layers are finer-grained with a dominantly mafic composition, whilst sulphide-bearing layers are also evident. Local xenoliths of sedimentary rocks are present. Feldspars are locally altered to albite, particularly where associated with quartz veins. Chlorite alteration is obvious at the contact with sedimentary rocks, whilst the orientation of biotite flakes gives rise to a pronounced foliation. In sheared sections of the gabbro, all primary textures may be obliterated. Trace pyrite, and to a lesser extent pyrrhotite and arsenopyrite, occur within the gabbro, although localised clusters of sulphides and even thin sulphide veins are common. Feldspar and actinolite are locally common, occurring as 1 to 2 mm wide monomineralic veins. Quartz veins and pegmatite dykes are also locally abundant, whilst aplite veins are relatively rare. Scheelite occurs in quartz-feldspar veins, but is also found randomly within the gabbroic rock. Mafic dykes, that are between 0.15 and 3 m thick, contain calcite veins and traces of scheelite, cross-cutting both the gabbro and granodiorite and are usually homogeneous and fine to medium grained, with sharp contacts to their host rocks.
The granodiorite is light grey, medium-grained and granoblastic, with a homogeneous and basically isotropic structure. It intrudes the gabbro with shallow dipping contacts that are either sharp or gradational. It forms an elongate intrusion in the interior of the gabbro body, as well as small, individual lenses in that intrusive. Dominant minerals are quartz, feldspar and biotite, with occasional 1 to 2 mm feldspars forming a dark grey, but locally reddish, spotty, weakly porphyritic rock. Opaque minerals include impregnations of arsenopyrite and traces of pyrite. The geochemistry of the granodiorite has features in common with S-type granites.
The Bothnian Supergroup sedimentary rocks to the east of the Åkerberg deposit are dominantly greywackes with occasionally well-developed sedimentary structures, such as cross-bedding, graded bedding, load casts and convolute folds. Locally, pyrite and pyrrhotite occur as prominent layers in sedimentary rocks that are intruded by the gabbro, but are also locally found within the gabbro (Billström et al., 2012).
Two different pegmatites types have been distinguished. The first occurs as whitish to reddish, steeply dipping dykes that are coarse- to very coarse-grained with a simple mineralogy dominated by quartz, feldspar, biotite and tourmaline, occurring as numerous small bodies both within the gabbro and the granodiorite. The second comprises sub-horizontal bodies with a more complex chemistry containing muscovite and garnet in addition to the assemblage of the first type. Near the ore zone, it occurs as a single, up to 30 m thick, sheet which adjoins the gold ore and has a dip of 5 to 10°N. It has surface dimensions of ~1000 x (100 to 200 m) and partly caps the gabbro. This two-mica rock is partially faulted to the south and may have a subhorizontal layering within coarser parts, whereas other parts are more granitic in appearance. When approaching the ore zone, it grades into a complex Li-Cs-Ta type pegmatite, with an assemblage of Li-Cs minerals (lepidolite, spodumene and pollucite), several tourmaline varieties, allemontite, topaz, amblygonite, cassiterite, columbite and microlite that occur in vein-like zones or masses. The two pegmatite types are interpreted to define two different generations which pre- and post-date the regional metamorphism, respectively. The first type, which has a simple mineralogy, is generally cross-cut by quartz veins, whilst the opposite is always true for the flat-lying second type of pegmatite (Billström et al., 2012).
Gold mineralisation at Åkerberg is atypical of the Skellefteå District. Gold is concentrated in the vicinity of narrow, sub-parallel, gabbro-hosted quartz veins or veinlets that are typically 1 to 2 mm thick. These zones of veining often expand at depth where they occasionally may carry grades of up to 50 g/t Au. A conspicuous characteristic is an ~300 m wide halo, with only very minor quartz veining, that contains erratically distributed sub-zones with 0.1 to 0.5 g/t Au. The mined ore extends into this halo which is defined by an ~10 m, occasionally reaching 30 m wide, and ~350 m long zone containing essentially vertical east-west trending quartz veins, and is basically delimited by two mylonite zones. The ore persisted to a depth of 150 m in the west, where it is displaced by the complex pegmatite, whereas to the east, it is truncated by the intruding granodiorite. The quartz veins strike and dip similar to, but yet distinct from, that of the mylonite zones. In the mined area, gold-associated veins are typically densely spaced, sometimes >50 veins per metre, forming a sheeted vein complex suggesting formation during tensional fracturing. These veins are continuous and can occasionally be followed for hundreds of metres along strike. Generally, such veins dip steeply to the north and occur in an en echelon arrangement. The quartz veins or veinlets constitute a sheeted vein complex, which suggests the veins did not form within shear structures but are due to tensional fracturing (Billström et al., 2012).
Only very minor alteration is observed, mainly involving albitisation of feldspar within the gabbro. Within the quartz veins, amphiboles form thin schlieren that are often parallel with the vein margins. Where there is a high frequency of cross-cutting microfractures, amphiboles on the margins of the quartz veins are slightly altered to form very thin greenish-brown Fe-rich chlorite rims along the quartz-amphibole contact with minor associated pyrrhotite and rare chalcopyrite. Spatial association between gold and veinlets of pyrrhotite and actinolite is rare. Gold-associated quartz veinlets always have low sulphide contents (typically <1%) comprising pyrrhotite with some pyrite, and occasionally ilmenite. However, there is a definite correlation between quartz vein density and gold grade. Scheelite is the only phase that apparently strictly follows gold, whilst tourmaline is also possibly a gold-associated phase (Billström et al., 2012).
Gold is very fine-grained, typically ~10 to 15 µm, whilst macroscopic gold can normally only be seen on sawn/smeared surfaces. Gold is associated with feldspar, scheelite and sphalerite, but is quite rare within quartz. It is found both along grain boundaries and intra-grain at sites within and near quartz veinlets. Quartz vein systems have different directions in different parts of the gabbro, and large zones within the gabbro body have anomalous, but sub-ppm contents of gold. Gold also locally occurs in both a homogeneous, dark grey, and a finer-grained and more heterogeneous part of the gabbro. In these settings, it is not accompanied by quartz veinlets. Enhanced gold levels are also found in the granodiorite and sometimes, but not always, quartz veins are present at such sites. Wherever granodiorite is more altered, some arsenopyrite, pyrite and quartz veinlets are locally evident (Billström et al., 2012).
Return to Top
Production, Reserves and Resources
To the end of 1996, the field has produced ~97 Mt of ore from 26 volcanic hosted massive sulphide deposits in a district of ~120 x 30 km, that contains >85 known deposits. At the end of 1995, 52 of these were estimated to collectively contain ~161 Mt before mining with an average grade of 0.7% Cu, 3.0% Zn, 47 g/t Ag, 1.9 g/t Au, 0.4% Pb and 0.8% As, often with significant accompanying Sb and Hg (Allen et al., 1996).
• Adak Fältet including Rudtjebäcken, Adak, Lindskold and Brannmyran;
Production from 1932 to 1977 - 6.34 Mt @ 3.0 % Zn, 0.1 % Pb, 0.8 % Cu (Hallberg et al., 2016);
Rudtjebäcken Production - 1947 to 1975 - 4.49 Mt +
Reserve 2012 - 1.01 Mt @ 3.0% Zn, 0.1% Pb, 0.9% Cu, 10 g/t Ag, 0.3 g/t Au, 32%S (Hallberg et al., 2012).
• Åkerberg
Production - 1989 to 2000 - 1.48 Mt @ 0.06% Pb, 0.04% Cu, 3.2 g/t Ag, 3.14 g/t Au (Hallberg et al., 2016).
• Åkulla
Åkulla Västra Production - 1938 to 1957 - 0.98 Mt @ 1.0% Cu, 12 g/t Ag, 0.7 g/t Au (Hallberg et al., 2016);
Åkulla Östra Resource - 0.2 Mt @ 1.7% Cu, 2.1 g/t Au (Bauer et al., 2014).
• Åsen
Past production - tonnage unknown @ 1.7% Cu, 1.9% Zn, 0.5% Pb, 64 g/t Ag, 1.0 g.t Au, 5% BaO, 23% S (Grip, 1978);
Remaining resource 2014 - >0.7 Mt @ 1.74% Cu, 1.84% Zn, 0.46% Pb, 64 g/t Ag, 1.0 g.t Au (Bauer et al., 2014).
• Bjurfors Gruvfält
Total production - 0.2 Mt @ 2.6% Cu, 27% S (Grip, 1978);
Remaining resource 2014 - 0.6 Mt @ 3.5% Zn, 0.2% Pb, 18 g/t Ag, 0.25 g/t Au (Bauer et al., 2014).
• Bjurtrask
Resource 2014 - 0.377 Mt @ 0.3% Cu, 6.6% Zn, 7 g/t Ag, 0.25 g/t Au (Bauer et al., 2014).
• Boliden
Total production from 1826 to closure in 1967 - 8.35 Mt @ 0.9% Zn, 0.3% Pb, 1.4% Cu, 50 g/t Ag, 15.5 g/t Au, 6.8% As, 600 ppm Sb (Grip and Wirstam 1970).
• Björkdal
Total production from 1988 to 2015 - 20.14 Mt @ 1.8 g/t Au (Hallberg et al., 2016)
Proved + Probable Reserves 31 December 2019 - 11.09 Mt @ 1.54 g/t Au (Mandalay Resources Reserve and Resources statement 2019);
Measured + Indicated Resources 31 December 2019 - 15.558 Mt @ 2.15 g/t Au (Mandalay Resources Reserve and Resources statement 2019);
Inferred Resources 31 December 2019 - 5.483 Mt @ 1.71 g/t Au (Mandalay Resources Reserve and Resources statement 2019).
• Holmtjärn
Deposit size estimate 1997 - 0.5 Mt @ 4.0% Zn, 0.4 % Pb, 0.4% Cu, 92 g/t Ag, 7.4 g/t Au, 0.9% As, 22% S (Allen et al., 1997).
• Hornträsk
Production - 1987 to 1991 - 0.88 Mt @ 4.6% Zn, 0.5% Pb, 1.08% Cu, 65 g/t Ag, 0.6 g/t Au (Hannington et al., 2003);
Remaining resource 2014 - 0.6 Mt @ 2.3% Zn, 1% Cu, 65 g/t Ag, 0.6 g/t Au (Bauer et al., 2014)
• Kankberg
Production from 1966 to 1970 - 1.16 Mt @ 1.8% Zn, 0.3% Pb, 1.4% Cu, 2.6 g/t Au, 52 g/t Ag (Hallberg et al., 2016);
Production from 2012 to 2019 - 2.9 Mt @ 3.5 g/t Au, 11 g/t Ag, 163 g/t Te (Voigt et al., Boliden, 2019);
Proved + Probable Reserves 31 December 2018 - 5.05 Mt @ 3.4 g/t Au, 9 g/t Ag, 162 g/t Te (Voigt et al., Boliden, 2019);
Measured + Indicated Resources 31 December 2018 - 0.87 Mt @ 3.9 g/t Au, 8 g/t Ag, 152 g/t Te (Voigt et al., Boliden, 2019);
Inferred Resources 31 December 2018 - 1.46 Mt @ 3.9 g/t Au, 7 g/t Ag, 161 g/t Te (Voigt et al., Boliden, 2019).
• Kedträsk
Production - 1969 to 2000 - 0.69 Mt @ 2.9% Zn, 0.2% Pb, 0.4% Cu, 24 g/t Ag, 0.5 g/t Au (Hallberg et al., 2016);
Past production - 3.9 Mt @ 2.9% Zn, 0.2% Pb, 0.4% Cu, 24 g/t Ag, 0.5 g/t Au (Bauer et al., 2014).
• Kimheden
Production - 1967 to 1969 and 1974 to 1975 - 0.13 Mt (Jansson et al., 2013);
Past production - 1 Mt @ 0.7% Zn, 1.17% Cu, 6 g/t Ag, 0.5 g/t Au (Bauer et al., 2014).
• Kristineberg
Production from 1940 to 2019 - 32.1 Mt @ 3.7% Zn, 1% Cu, 37.7g/t Ag, 1.2 g/t Au (Boliden, 2019).
• Kristineberg and Rävliden North
Proved Reserve 31 December 2019 - 3.66 Mt @ 5.4 % Zn, 0.3 % Pb, 0.6 % Cu, 35 g/t Ag, 0.5 g/t Au, g/t Au (Boliden, 2019);
Measured + Indicated Resources 31 December 2019 - 5.24 Mt @ 5.0 % Zn, 0.5 % Pb, 0.9 % Cu, 65 g/t Ag, 0.4 g/t Au (Boliden, 2019);
Inferred Resources 31 December 2019 - 6.12 Mt @ 2.9 % Zn, 0.4 % Pb, 0.9 % Cu, 57 g/t Ag, 0.4 g/t Au (Boliden, 2019).
• Lådal
Deposit size estimate 1997 - 4.4 Mt @ 5.8 % Zn, 1.7 % Pb, 0.1 % Cu, 149 g/t Ag, 1.9 g/t Au, 0.1% As, 0.01% Sb, 12% S (Allen et al., 1997).
• Långdal
Production - 1950 to 1999 - 4.74 Mt @ 5.7% Zn, 1.7% Pb, 0.1% Cu, 160 g/t Ag, 1.9 g/t Au, 32%S (Hallberg et al., 2012).
• Långsele
Production from 1951 to 1991 - 11.2 Mt @ 3.9 % Zn, 0.3 % Pb, 0.6 % Cu, 25 g/t Ag, 0.9 g/t Au (Hallberg et al., 2016).
• Maurliden
Production from 2000 to 2018 - 3.8 Mt @ 4.0 % Zn, 0.4 % Pb, 0.3 % Cu, 51 g/t Ag, 1.4 g/t Au (Collin, Boliden, 2019);
Proved Reserve 31 December 2019 - 0.073 Mt @ 4.40 % Zn, 0.37 % Pb, 0.56 % Cu, 56 g/t Ag, 1.45 g/t Au, g/t Au (Collin, Boliden, 2019);
Maurliden is composed of:
Maurliden Västra, Prod. 2000 to 2015 - 2.58 Mt + Reserve 2016 - 2.80 Mt @ 3.4% Zn, 0.4% Pb, 0.2% Cu, 49 g/t Ag, 0.9 g/t Au (Hallberg et al., 2016);
Maurliden Östra, Prod. 2010 to 2015 - 1.73 Mt + Reserve 2016 - 0.56 Mt @ 0.77% Zn, 1.61% Cu, 23.7 g/t Ag, 1.08 g/t Au (Hallberg et al., 2016).
• Näsliden
Production - 1963 to 1989 - 4.03 Mt @ 2.9% Zn, 0.3% Pb, 1.2% Cu, 37 g/t Ag, 1.4 g/t Au, 1.2% As, 0.12% Sb, 29%S (Hallberg et al., 2012).
• Norrliden Norra
Reserves 2012 - 2.34 Mt @ 2.9 % Zn, 0.3 % Pb, 0.7 % Cu, 43 g/t Ag, 0.54 g/t Au (Hallberg et al., 2012);
Indicated + Inferred Resource 2004 - 1.5 Mt @ 4.4 % Zn, 0.4 % Pb, 0.8 % Cu, 59.9 g/t Ag (Wheeler 2006).
• Petiknäs North
Deposit size estimate 1997 - 1.3 Mt @ 5.6 % Zn, 0.9 % Pb, 1.3 % Cu, 103 g/t Ag, 5.6 g/t Au, 2.7% As, 0.234% Sb, 14% S (Allen et al., 1997).
• Petiknäs Södra (South).
Production from 1989 to 2007 - 5.4 Mt @ 4.9 % Zn, 0.9 % Pb, 0.9 % Cu, 102 g/t Ag, 2.4 g/t Au (Hallberg et al., 2016), or,
Deposit size estimate 1997 - 6.5 Mt @ 4.8 % Zn, 0.9 % Pb, 1.1 % Cu, 108 g/t Ag, 2.3 g/t Au, 0.4% As, 0.02% Sb, 37% S (Allen et al., 1997).
• Rakkejaur
Production - 1934 to 1988 - 0.72 Mt @ 2.3% Zn, 0.2% Pb, 0.3% Cu, 45 g/t Ag, 1.0 g/t Au (Hallberg et al., 2016);
Production + Resources - 20 Mt @ 2.3% Zn, 0.2% Pb, 0.3% Cu, 50 g/t Ag, 1.0 g/t Au (Bauer et al., 2014).
• Rävliden
Production from 1936 to 1991 - 7.54 Mt @ 4.2 % Zn, 0.8 % Pb, 1.0 % Cu, 90 g/t Ag, 0.5 g/t Au (Hallberg et al., 2016).
• Rävlidmyran
Production - 1942 to 1976 - 1.9 Mt @ 3.9% Zn, 0.6% Pb, 1.0% Cu, 51 g/t Ag, 0.8 g/t Au (Hallberg et al., 2016);
Deposit size estimate 1997 - 7.5 Mt @ 3.9% Zn, 0.6% Pb, 1.0% Cu, 51 g/t Ag, 0.8 g/t Au, 0.1% As, 0.01% Sb, 18% S (Allen et al., 1997).
• Renström
Production from 2012 to 2019 - 14.014 Mt @ 6.1 % Zn, 1.3 % Pb, 0.7 % Cu, 143 g/t Ag, 2.7 g/t Au (Collin, Boliden, 2019);
Proved + Probable Reserves 31 December 2019 - 4.0 Mt @ 5.7 % Zn, 1.1 % Pb, 0.4 % Cu, 111 g/t Ag, 2.11 g/t Au (Collin, Boliden, 2019);
Measured + Indicated Resources 31 December 2019 - 1.2 Mt @ 4.8 % Zn, 1.0 % Pb, 0.4 % Cu, 111 g/t Ag, 2.11 g/t Au (Collin, Boliden, 2019);
Inferred Resources 31 December 2019 - 1.5 Mt @ 9.3 % Zn, 1.6 % Pb, 0.96 % Cu, 133 g/t Ag, 2.2 g/t Au (Collin, Boliden, 2019).
• Sjömalmen ~7.5 km west of Maurliden
Past production - 2.3 Mt @ 2.2% Zn, 0.5% Pb, 0.5% Cu, 47 g/t Ag, 0.8 g/t Au (Bauer et al., 2014).
Remaining resources 2014 - 0.1 Mt @ 3.65% Zn, 0.39% Pb, 2.1% Cu, 50 g/t Ag, 2.9 g/t Au (Bauer et al., 2014).
• Storliden
Production - 2002 to 2008 - 1.7 Mt @ 10% Zn, 1.0% Cu, 12 g/t Ag, 0.7 g/t Au (Hallberg et al., 2016).
• Svansele
Deposit size estimate 1997 - 2.9 Mt @ 0.3 % Zn, 0.1 % Pb, 0.3 % Cu, 4 g/t Ag, 0.2 g/t Au, 0.1% As, 0.003% Sb, 36% S (Allen et al., 1997).
• Udden
Production from 1971 to 1990 - 5.95 Mt @ 4.8 % Zn, 0.3 % Pb, 0.5 % Cu, 39 g/t Ag, 0.8 g/t Au (Hallberg et al., 2016), or,
Deposit size estimate 1997 - 6.7 Mt @ 4.3 % Zn, 0.3 % Pb, 0.4 % Cu, 36 g/t Ag, 0.7 g/t Au, 0.1% As, 0.015% Sb, 24% S (Allen et al., 1997).
The most recent source geological information used to prepare this decription was dated: 2020.
Record last updated: 31/7/2020
This description is a summary from published sources, the chief of which are listed below. © Copyright Porter GeoConsultancy Pty Ltd. Unauthorised copying, reproduction, storage or dissemination prohibited.
Boliden Renstrom Kristineberg
|
|
|
Adomako-Ansah, K., Ishiyama, D. and Allen, R., 2018 - Origin of Zn-Pb-Sb-Au mineralization adjacent to the Paleoproterozoic Boliden Au-rich VMS deposit, Sweden: evidence from petrographic and oxygen isotope characteristics: in Mineralium Deposita v.53, pp. 683-700.
|
Allen, R. and Svenson, S.-A., 2004 - 1.9 Ga Volcanic Stratigraphy, Structure, and Zn-Pb-Cu-Au-Ag Massive Sulfide Deposits of the Renstrom Area, Skellefte District, Sweden: in Allen, R.L., Martinsson, O. and Weihed, P., (Eds.) 2004 Svecofennian Ore-Forming Environments of Northern Sweden - Volcanic-Associated Zn-Cu-Au-Ag, Intrusion-Associated Cu-Au, Sediment-Hosted Pb-Zn, and Magnetite-Apatite Deposits in Northern Sweden Society of Economic Geologists, Guidebook Series, v.33, pp. 65-88.
|
Allen, R.L., Weihed, P. and Svenson, S.-A., 1996 - Setting of Zn-Cu-Au-Ag massive Sulfide deposits in the evolution and facies architecture of a 1.9 Ga marine volcanic arc, Skellefte district, Sweden: in Econ. Geol. v.91, pp. 1022-1053.
|
Areback, H., Barrett, T.J., Abrahamsson, S. and Fagerstrom, P., 2005 - The Palaeoproterozoic Kristineberg VMS deposit, Skellefte district, northern Sweden, Part I: Geology: in Mineralium Deposita v.40, pp. 351-367.
|
Barrett, T.J., MacLean, W.H. and Areback, H., 2005 - The Palaeoproterozoic Kristineberg VMS deposit, Skellefte district, northern Sweden. Part II: chemostratigraphy and alteration: in Mineralium Deposita v.40, pp. 368-395.
|
Bauer, T.E., Skytta, P., Hermansson, T., Allen, R.L. and Weihed, P., 2014 - Correlation between distribution and shape of VMS deposits and regional deformation patterns, Skellefte district, northern Sweden: in Mineralium Deposita v.49, pp. 555-573.
|
Bergman Weihed, J., Bergstrom, U., Billstrom, K. and Weihed, P., 1996 - Geology, Tectonic Setting, and Origin of the Paleoproterozoic Boliden Au-Cu-As Deposit, Skellefte District, Northern Sweden: in Econ. Geol. v.91, pp. 1073-1097.
|
Billstrom K, Weihed P 1996 - Age and provenance of host rocks and ores in the Paleoproterozoic Skellefte district, northern Sweden: in Econ. Geol. v91 pp 1054-1072
|
Billstrom, K., Mattson, B., Soderlund, U., Areback, H. and Broman, C., 2012 - Geology and Age Constraints on the Origin of the Intrusion-Related, Sheeted Vein-Type Akerberg Gold Deposit, Skellefte District, Sweden: in Minerals (MDPI) v. 2, pp. 385-416.
|
Chmielowski, R.M., Jansson, N., Persson, M.F. and Fagerstrom, P., 2016 - 3D modelling of hydrothermal alteration associated with VHMS deposits in the Kristineberg area, Skellefte district, northern Sweden: in Mineralium Deposita v.51, pp. 113-130.
|
Grip, E., 1978 - Sweden - Extract on the Skellefte District of Northern Sweden: in Bowie, S.H.U., Kvalheim, A. and Haslam, H.W., 1978 Mineral Deposits of Europe The IMM, London v.,1 pp. 144-162.
|
Hallberg, A., Bergman, T., Gonzalez, J., Larsson, D., Morris, G. A., Perdahl, J. A., Ripa, M., Niiranen, T. and Eilu, P., 2012 - Metallogenic areas in Sweden: in Eilu, P., 2012 Mineral deposits and metallogeny of Fennoscandia, Geological Survey of Finland, Special Paper 53, pp. 139-206.
|
Hannington, M.D., Kjarsgaard, I.M., Galley, A.G. and Taylor, B., 2003 - Mineral-chemical studies of metamorphosed hydrothermal alteration in the Kristineberg volcanogenic massive sulfide district, Sweden: in Mineralium Deposita v.38, pp. 423-442.
|
Imana, M., Allen, R. and Barrett, T., 2005 - Volcanic stratigraphy, chemical stratigraphy and alteration system of the Storliden massive sulphide deposit, Skellefte district, northern Sweden: in Mao, J. and Bierlein, F.P., (Eds) 2005 Mineral Deposit Research: Meeting the Global Challenge, The 8th Biennial SGA Meeting is held in Beijing, August 18-21, 2005, Proceedings, V.1, pp. 627-630.
|
Jansson, N.F., Hermansson, T., Persson, M.F., Berglund, A. and Kruuna, A., 2013 - Recent advances in structural geology, lithogeochemistry and exploration for VHMS deposits, Kristineberg area, Skellefte District, Sweden: in 12th SGA Biennial Meeting, 12-15 August 2013, Uppsala, Sweden. v.2, pp. 545-548.
|
Johansson, S., 2017 - Ore mineralogy and silver distribution at the Ravliden N volcanogenic massive sulphide deposit, Skellefte district, Sweden: in Lulea University of Technology Department of Civil, Environmental and Natural Resources Engineering, Masters Thesis, 117p.
|
Malehmir, A., Thunehed, H. and Tryggvason, A., 2009 - The Paleoproterozoic Kristineberg mining area, northern Sweden: Results from integrated 3D geophysical and geologic modeling, and implications for targeting ore deposits: in Geophysics v.74, pp. B9-B22.
|
Malehmir, A., Tryggvason, A., Juhlin, C., Rodriguez-Tablante, J. and Weihed, P., 2006 - Seismic imaging and potential field modelling to delineate structures hosting VHMS deposits in the Skellefte Ore District, northern Sweden: in Tectonophysics v.426, pp. 319-334.
|
Malehmir, A., Tryggvason, A., Lickorish, H. and Weihed, P., 2007 - Regional structural profiles in the western part of the Palaeoproterozoic Skellefte Ore District, northern Sweden: in Precambrian Research v.159, pp. 1-18.
|
Rincon, J., Jansson, N., Thomas, H., Kaiser, M.C., Persson, M.F., Nordfeldt, E. and Wanhainen, C., 2024 - Ore Remobilization History of the Metamorphosed Ravliden North Volcanogenic Massive Sulfide Deposit, Skellefte District, Sweden: in Econ. Geol. v.119, pp. 907-934. doi: 10.5382/econgeo.5083.
|
Schlatter, D.M., Barrett, T.J. and Abrahamsson, S., 2003 - Chemostratigraphy of metamorphosed and altered Paleoproterozoic volcanic rocks associated with massive sulfide deposits at Ravliden and Kristineberg West, Skellefte district, Sweden: in Eliopoulos, 2003 Mineral Exploration and Sustainable Development, Seventh Biennial SGA Meeting, Athens, Greece, 24-28 August 2003, Millpress, Rotterdam, Proceedings, pp. 1103-1106.
|
Schwarz, G., Kathol, B., Ripa, M., Thunholm, B., Lynch, E.P. and Jonberger, J., 2019 - Report on pilots: the Kristineberg and Nautanen mining areas in Northern Sweden: in CHPM2030, Deliverable D 6.2 Appendix 6.2.4, 72p.
|
Sillitoe R H, Hannington M D, Thompson J F H 1996 - High Sulfidation deposits in the volcanogenic massice Sulfide environment: in Econ. Geol. v 91 pp 204-212
|
Skytta, P. Bauer, T., Hermansson, T., Dehghannejad, M., Juhlin, C., Garcia, M., Hubert, J. and Weihed, P., 2012 - Structural evolution of the VMS-hosting Kristineberg area, Sweden - constraints from structural analysis and 3D modelling: in Solid Earth Discussions, v.4, pp. 1281-1315.
|
Talbot C J 1988 - A desk analysis of the tectonic history of the Langdal mine, Skellefte District, Sweden: in Econ. Geol. v83 pp 647-656
|
Tryggvason, A., Malehmir, A., Rodriguez-Tablante, J., Juhlin, C. and Weihed, P., 2006 - Reflection Seismic Investigations in the Western Part of the Palaeoproterozoic VHMS-Bearing Skellefte District, Northern Sweden: in Econ. Geol. v.101, pp. 1039-1054.
|
Vivallo W 1987 - Early Proterozoic bimodal volcanism, hydrothermal activity and massive Sulfide deposition in the Boliden-Langdal area, Skellefte district, Sweden: in Econ. Geol. v82 pp 440-456
|
Wagner T, Boyceb A J, Jonssonc E and Fallick A E 2004 - Laser microprobe sulphur isotope analysis of arsenopyrite: experimental calibration and application to the Boliden Au-Cu-As massive sulphide deposit: in Ore Geology Reviews v25 pp 311-325
|
Weihed P, Arndt N, Billstrom K, Duchesne J C, Eilu P, Martinsson O, Papunen H and Lahtinen R 2005 - Precambrian geodynamics and ore formation: The Fennoscandian Shield : in Ore Geology Reviews v27 pp 273-322
|
Porter GeoConsultancy Pty Ltd (PorterGeo) provides access to this database at no charge. It is largely based on scientific papers and reports in the public domain, and was current when the sources consulted were published. While PorterGeo endeavour to ensure the information was accurate at the time of compilation and subsequent updating, PorterGeo, its employees and servants: i). do not warrant, or make any representation regarding the use, or results of the use of the information contained herein as to its correctness, accuracy, currency, or otherwise; and ii). expressly disclaim all liability or responsibility to any person using the information or conclusions contained herein.
|
Top | Search Again | PGC Home | Terms & Conditions
|
|