Redstone Copper Belt - Coates Lake, Hayhook, Keele River |
|
NWT, Canada |
Main commodities:
Cu Ag
|
|
 |
|
 |
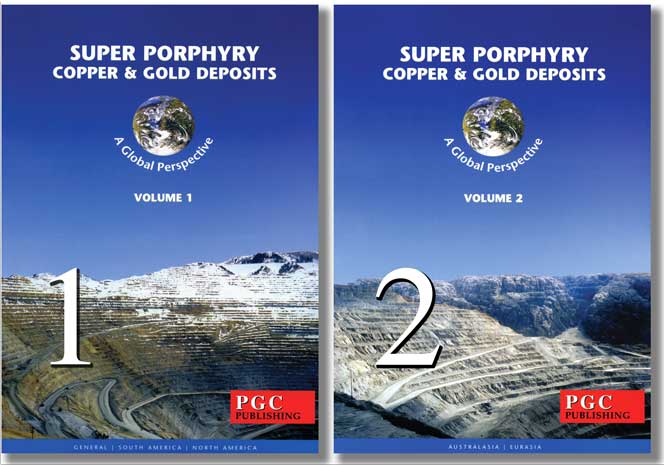 |
Super Porphyry Cu and Au

|
IOCG Deposits - 70 papers
|
All papers now Open Access.
Available as Full Text for direct download or on request. |
|
 |
The arcuate, ~200 km long Redstone Copper Belt, the Coates Lake sediment hosted Cu-Ag deposit and Hayhook and Keele River stratabound Cu occurrences are located in the Mackenzie Mountains of western Northwest Territories, Canada. The Coates Lake deposit, towards the south of the belt, is ~300 km south of Norman Wells, 115 km NE of Tungsten and 170 km WSW of Wrigley (#Location - Coates Lake: 62° 41' 48", 126° 37' 41"W).
This corridor of copper mineralisation is hosted within Neoproterozoic sedimentary rocks that are younger than 780 Ma and belong to the Mackenzie Mountains and younger Windermere supergroups. The sequence represents an episode of extension and two glacial events, followed by a transition to a passive-margin regime along the western edge of Laurentia (Young et al., 1979; Aitken, 1991; Narbonne and Aitken, 1995; Turner and Long, 2008). Stratabound copper mineralisation is concentrated in the Coates Lake Group, at the transition between these two packages of rocks, which also corresponds to a transition from oxidised to reduced conditions.
The ribbon of copper deposits and occurrences that define the Redstone Copper Belt closely parallels a thrust corridor on the eastern margin of the Cordilleran Orogen, within the Mackenzie Fold and Thrust Belt. The latter is a broad, thin-skinned, east vergent thrust and fold belt that has transposed the host Neoproterozoic sequences over Palaeozoic successions on the margin of the Canadian Shield. Similar younger lithologies overlie the host sequence to the west. Deformation is generally attributed to the contractional Late Cretaceous to Palaeocene Laramide Orogeny (Aitken and Long, 1978; Johnston, 2008). The host rocks have been metamorphosed to sub-greenschist grades but preserve sedimentary textures (Martel et al., 2011).
Stratabound Cu deposits and occurrences within the Redstone Copper Belt extend from ~60 km SSE of Coates Lake, to the Keele River area which is ~150 km NNW of Coates Lake. In addition to the stratabound deposits, there are numerous discordant vein-vug-breccia Cu and carbonate hosted Zn-Pb±Cu±Ag breccia and vein occurrences and deposits within rocks of the same Neoproterozoic succession, but also in younger Palaeozoic hosts. These occurrences lie within the same mineralised trend, in close vicinity to, between and beyond the key stratabound Cu deposits, as illustrated on the Mackenzie Mountains plan below. The occurrence of these younger mineralisation styles and their significance, as well as their possible inter-relationship is discussed separately at the end of this summary.
Copper occurrences in the Coates Lake area were discovered in 1961 during regional prospecting by the Nahanni Sixty Syndicate. In the following year, reconnaissance geological mapping and sampling were undertaken along the exposed strike length of the Coates Lake deposit. In 1961-62, Redstone Resources Inc. (then Redstone Mines Ltd) completed preliminary exploration on three lateral extensions of the Coates Lake mineralised zone, North Redstone and Hidden Valley, 25 to 50 km north, and South Redstone 53 km to the south, as well as sections of the Coates Lake deposit. In 1963, Redstone Resources acquired the Nahanni Sixty Syndicates interests and by the end of 1964 had drilled 45 'A' and 'B' sized holes into the Coates Lake deposit for 6902 m of core (Gourlay, 2005).
In 1970, Cerro Mining Company of Canada Limited optioned the Coates Lake deposit area, carried out reconnaissance geological mapping and geochemical sampling, and in 1971 drilled 1375 m in 3 diamond core holes. Two of these failed to reach the copper beds due to down-faulting, whilst the third intersected 1.13 m @ 3.9% Cu. The option was subsequently relinquished. Shell Canada Resources Ltd entered into an option agreement with Redstone Resources and after performing a seismic survey over the deposit in 1975, drilled 8 HQ → NQ (→ BQ) diamond holes to as much as ~1500 m depth in 1976-77 for 7225 m of core. The resources quoted later in this summary were estimated, on the basis of 7 of these holes, plus the Cerro Mining hole from 1971 (Hildebrand, 1978; Gourlay, 2005). See the table of intersections below, and drill hole collar locations on the Coates Lake geological map below. This figure was calculated prior to the inception of NI 43-101 and is considered by Gourlay (2005) to be consistent with NI 43-101 Inferred Resources. No further drilling has been undertaken to 2018 to support, refine or expand these resources. Shell returned the claims to Redstone Resources in 1982 (Gourlay, 2005).
In 1990, a mapping program attempted to delineate thicker sections of the deposit. In 1996 Redstone Resources became a wholly owned subsidiary of Franco-Nevada Mining Corporation which, in turn, in 2002 became a subsidiary of Newmont Mining Corporation. In 2002, Redstone Resources were acquired from Newmont by Lumina Copper Corporation. In 2006, Lumina was acquired by Western Copper Corporation, who in 2011 demerged section of its copper portfolio, including the Redstone Leases, to Copper North Mining Corporation, who retained title to the Coates Lake deposit in 2018 via its wholly owned subsidiary, Redbed Resources Corporation (Government of Northwest Territories, 2016).
Geology
The sequence in the Mackenzie Mountains is as follows:
BASEMENT
To the west, both the Mackenzie Mountains and Windermere supergroups unconformably overlie the 1.66 to 1.60 Ga metasedimentary Wernecke Supergroup. This supergroup is composed of three sequences, each of which is up to 4 km thick, namely the Fairchild Lake Group - siltstone, sandstone and minor carbonate; Quartet Group - pyritic black shale and slate and an overlying succession of siltstone and minor fine-grained sandstone; and - Gillespie Lake Group - dolomitised carbonate and subordinate clastic rocks (Furlanetto, 2015). The 1.71 Ga gabbro to tholeiitic diorite with lesser syenite and anorthosite which constitute the Bonnet Plume River Intrusions occur in the area, as well as the mafic Slab Volcanics, which Furlanetto et al., (2013) suggest may together represent obduction of an exotic oceanic terrane over the Wernecke Supergroup during the 1600 Ma Racklan Orogeny.
To the east, basement is largely concealed at depth below the thick succession of Neoproterozoic sedimentary rocks that continue eastward from the exposures in the Mackenzie Mountains, and by both Palaeozoic and Mesozoic cover sequences. It comprises the NNE-SSW trending, juvenile, 2.0 to 1.8 Ga Wopmay Orogen which includes the 2.1 to 1.88 Hottah Terrane magmatic arc and 1.88 to 1.84 Ga Great Bear Magmatic Zone on its eastern margin with the Archaean Slave Province. A further buried putative juvenile magmatic arc is believed to be concealed between these tectonic elements and the Mackenzie Mountains. This basement suite is extensively exposed as part of the Canadian Shield to the east of the Interior Platform, ~500 km NE of the Redstone Copper Belt, where it is separated from the overlying Neoproterozoic to Mesozoic cover by a Mesoproterozoic succession.
Click here to expand
MACKENZIE MOUNTAINS SUPERGROUP
Which is of Neoproterozoic age, 4 to 6 km thick and is composed of the following units:
Tabasco Formation, previously known as Unit H1, is the lowest strata exposed in the Mackenzie Fold Belt, and comprises a >480 m thick cherty dolostone unit with abundant stromatolites. Fluorite occurs widely within the unit and rare pyrobitumen is found within vugs. From the folding profile it is believed that bedded rocks thousands of metres thick intervene between the Tabasco Formation and any rigid basement. The formation is only exposed in a few outcrops in the Mackenzie Mountains, but elsewhere lie unconformably on deformed Mesoproterozoic rocks.
Tsezotene Formation, which is >1500 m thick and is composed of dark grey, green and medium brown shale, purple, maroon, grey and green argillite, light grey-green, grey and brown siltstone, fine to medium grained quartzite and interbeds towards the top of orange and buff weathering sandy dolostone to dolostone. The darker shales and quartzites contain fine grained pyrite. The basal contact with the shallow-water carbonate rocks of the Tabasco Formation represents a flooding surface (Turner and Long, 2008).
Katherine Group, 300 to 1375 m thick - well bedded pink, white and purple resistant quartzites and sandstones with subordinate shale, slate and dolostone. Crossbedding, ripple marks, scour and fill structures are abundant within the coarser clastics and mudcracks are evident within the interbedded shales. The sandstones are very siliceous and evenly grained. The basal unit of the sequence has a sharp contact with the underlying Tsezotene Formation, with local evidence of erosion, while the contact with the overlying Little Dal Group is typically sharp, and represents a third-order sequence boundary (Turner and
Long, 2008). U-Pb dated detrital zircon from this group are dated at 1083 and 1010 Ma, providing a minimum age for this unit (Rainbird et al., 1996;
Leslie 2009).
Little Dal Group - a 0 to 2500 m thick sequence of carbonate and evaporite strata, divided into (after Turner and Long, 2012):
Dodo Creek Formation, a basal sandstone overlain by shales;
Silverberry and Stone Knife formations, which are lateral equivalents to the NW and SE respectively and are principally limestones composed of ooid and interclast grainstones with numerous algal stromatolites, and intraclastic and molar-tooth carbonate rocks.
Gayna Formation, is dominantly a platy dolostone, comprising oolitic and intraclastic dolograinstones, dolomudstone and local stromatolite, molar-tooth and cherty rocks containing abundant mudcracks, ripple marks, with salt casts near the top;
Ten Stone Formation, (formerly known as the Gypsum Formation), a bedded gyspum/anhydrite blanket that is locally >500 m thick. It is very widespread, being found as far afield as the Minto Inlier on Victoria Island, >1000 km to the NNE. This blanket provides a surface that the major thrust faults commonly follow;
Snail Spring Formation, (previously the Rusty Shale Formation) - a rusty shale, sandstone and stromatolitic carbonate;
Ram Head formation, a 0 to 600 m thick, cyclical unit of stromatolitic, pure and impure dolostones and subordinate intercalated shales which includes stromatolitic dolostone and limestone;
'Little Dal Basalts', an unconfirmably overlying, regionally discontinuous 0 to 92 m thick suite of continental-tholeiitic, plagioclase-pyroxene phyric basalt and dolerite (Jefferson, 1983; Dudás and Lustwerk, 1997). Zircons from a dolerite within the Little Dal Basalts, 20 km north of Coates Lake have been dated at 774.9±0.5 Ma (weighted mean 206Pb/238U age; CA-ID-TIMS), giving a youngest age for the Little Dal Group and a maximum age for the overlying Coates Lake Group.
The Little Dal Basalts are interpreted to be part of the ~780 Ma regional Gunbarrel mafic magmatic event along the western margin of Laurentia, heralding the 780 to 720 Ma early stage of the Rodinia supercontinent breakup. The Gunbarrel mafic magmatic event involved the intrusion of a dyke swarm that spans a ~2400 km interval across western Laurentia from Wyoming (U.S.A), to the Mackenzie Mountains, NWT (Canada) (Park et al., 1995; Harlan et al., 2003). This correlation is based on geochemical, palaeomagnetic and isotopic similarities between the basalts and the Tsezotene Sills which are are dated at ~780 Ma, cut the Mackenzie Supergroup and are part of the Gunbarrel dyke/sill swarm (Harlan et al., 2003)
Coates Lake Group, which is the principal host to sediment hosted copper mineralisation in the Redstone Copper Belt. It rests unconformably to disconformably on the Little Dal Basalts or Ram Head Formation of the Mackenzie Mountains Supergroup, and is, in turn, unconformably overlain by the Windermere Supergroup, but is not assigned to either supergroup (Jefferson and Parrish, 1989). It comprises a series of lithofacies deposited within three formations during rifting across five distinct basins or 'embayments' (Jefferson, 1983). These formations are:
Thundercloud Formation, which varies in thickness up to 300 m and is subdivided into four lithofacies members TH1 to TH4. The siliciclastic and carbonate rocks of these four lithofacies members range greatly in character and distribution between each depositional basin. They comprise (Milton et al., 2017),
• TH1 - basal conglomerate and coarse volcanic clastic rocks derived from Little Dal Basalt, and hematitic chert breccia;
• TH2 - interbedded maroon mudstone and buff dolostone;
• TH3 - white, buff to grey/brown sandstones, cyclically interbedded with black, grey, red or green pyritic shales;
• TH4 - various carbonate lithologies with interbeds of dark laminated shale, which may contain limestone, dolostone, evaporites, stromatolites or collapse breccias.
Redstone River Formation - composed of a lower 0 to over 1220 m of evaporites and red, fine to coarse, siliciclastic rocks (Jefferson and Ruelle, 1986), divided into five lithofacies (Milton et al., 2017), as follows,
• RR1 - 0 to 200 m of dominantly bedded, laminated or brecciated sulphate or chloride evaporites with common interbeds of red siltstone or mudstone and an upper contact zone of collapse breccias. The lower contact with the underlying TH4 evaporitic carbonate rocks to the RR1 evaporite member is gradational;
• RR2 - a carbonate boulder conglomerate, interpreted to be a fanglomerate restricted to the basin margins, grading upwards and basinward to a coarse, well-bedded sandstone to pebble conglomerate, and minor mudstone;
• RR4 - is up to more than 1200 m in thickness and is interpreted (Milton et al., 2017) to represent distal alluvial fan to flood-plain deposits which were largely derived from copper-rich mafic detritus eroded from the Little Dal Basalts. It comprises three facies,
i). thick sequences of red, thin bedded, hematitic and dolomitic, fine-grained sandstone, siltstone and minor mudstone that are planar- or cross-laminated and normally graded;
ii). a thick sequences of fine-grained sandstone and siltstone that is massive, hematitic and dolomitic;
iii). lenses of sandstone that are slightly coarser;
The RR4 sequence is characterised by dewatering structures, sedimentary slumps, desiccation cracks, and common gypsum veins. In the basin centre, RR4 generally directly overlies the basal RR1 conglomerates.
Coppercap Formation, which is dominated by carbonate-rocks overlying the Redstone River Formation, represents a major, regional marine transgression and a change from sub-aerial and shallow water to a marine environments of sedimentation (Ruelle, 1982; Jefferson, 1983). The contact between the two formations is transitional, with the lowermost member of the Coppercap Formation, CP1, informally known as 'The Transition Zone'. The formation is divided into seven lithofacies members, as follows (Milton, 2015):
• CP1 - which is up to 110 m thick and is composed of several transgressive-regressive cycles of red mudstone, siltstone and fine-grained sandstone interbedded with evaporites and massive to laminated dolostone or dolomitic limestone. Some of the carbonates are microbially laminated and some exhibit laminoid to bird's-eye shaped fenestrae infilled with quartz, chert, dolomite or calcite. Gypsum and anhydrite occur as massive beds or nodules. CP1 is interpreted to have formed in a marginal-marine or sabkha environment, with stratigraphy that is highly variable between depositional basins. It is a common host for sediment hosted copper mineralisation. This mineralisation is most strongly concentrated in the lowermost three of seven microbial laminate intervals (named from base to top as Beds B1 to B7), each of which is 0.2 to ~6 m in thickness, and separated by up to 15 m of red and green siltstones and mudstones of the CP1 member (Jefferson and Ruelle, 1986);
• CP2 - foetid, black to dark grey, pyritic, shaley limestones;
• CP3 - impure, normally graded and rhythmically bedded grey limestones;
• CP4 - light grey weathering calcarenites, rudstones, bedded cherts, stromatolites and dolostones;
• CP5 - grey, pure, normally graded, rhythmically bedded limestones which are shaley at the base, and have a similar appearance to CP3;
• CP6 - light grey weathering calcarenites, rudstones, bedded cherts, stromatolites and dolostones which are similar appearance to CP4;
• CP7 - blue-grey dolomitic siltstone and microbially laminated dolostone.
Units 2 to 7 inclusive have a total thickness of ~350 m. Members CP2 to CP4 and CP5 to CP7 represent two gradational, shallowing-upwards cycles of marine carbonate sedimentation. These two shallowing upwards cycles are interrupted by shallow-marine facies in CP4, CP6 and CP7. CP2, CP3 and CP5 are composed of normally-graded rocks and represent Bouma cycles, containing reworked intrabasinal carbonate material, and are interpreted to have been deposited from turbidity currents (Jefferson, 1983).
A 187Re/187Os whole rock model 1 date of 732.2±3.9 Ma was interpreted as a direct depositional age by for member CP4 (Rooney et al., 2014).
WINDERMERE SUPERGROUP
Which is of Neoproterozoic to Lower Cambrian age, is 5 to 7 km thick and is composed of the following units:
Rapitan Group, the lowermost stratigraphic unit of the Windermere Supergroup which unconformably or disconformably overlies the Coates Lake Group. Where the Coates Lake Group was either not deposited or was removed by pre-Rapitan erosion, the Rapitan Group rests unconformably over units of the Mackenzie Mountains Supergroup, including the Little Dal Group (Jefferson, 1983). It comprises the
Mt. Berg Formation - a laterally discontinuous diamictite and orthoconglomerate that is typically no more than a few tens of metres thick, but may locally exceed 300 m, although it is also frequently absent. It is best developed in the vicinity of growth faults cross-cutting the underlying Coates Lake and Little Dal groups;
Sayunei Formation - an up to 700 m thick, rhythmically bedded, massive to laminated maroon to purple sequence of hematitic sandstones, siltstones and mudstones with dropstones and interbeds of conglomerate and iron formation;
Shezal Formation - up to 800 m of massive, recessively weathering grey or tan diamictite with minor sandstone and siltstone intervals. Like
the Sayunei Formation, it has been interpreted to be submarine diamictite transported and deposited by sediment-gravity flows.
The Sayunei and Shezal formations are more areally widespread (Yeo, 1981), and in general, are thicker where the underlying Coates Lake Group is also thicker, reflecting the reactivation of synsedimentary growth faults that were also active during Coates Lake Group deposition (Jefferson, 1983). The Rapitan Group is interpreted to have a maximum age of 717.43±0.14 Ma (Macdonald et al., 2010), and has been correlated with the global Sturtian glacial event which includes the Grand Conglomérat in the Central African Copperbelt.
Hay Creek Group, which forms the upper section of the Windermere Supergroup, and overlies the Rapitan Group. It has a much greater areal persistence than the underlying rift-related rocks of the Rapitan and Coates Lake groups, and is interpreted to represent slope to shelf deposition along a thermally subsiding passive margin (Narbonne and Aitken, 1995; Turner et al., 2011). It comprises the:
Twitya Formation, 550 to 1100 m thick, commencing with a sharp change to a black pyritic or dark bituminous limestone followed by dark mudstone, siltstone and fine sandstone;
Keele Formation, 450 to 600 m thick, orange weathering dolomitic sandstone, sandy dolostone and dolostone with local mafic lava flows;
Ice Brook Formation, up to 300 m of diamictites that are correlated with the ~635 Ma global Marinoan glacial event which includes the Petit Conglomérat in the Central African Copperbelt. The Ice Brook Formation occurs locally as a lateral equivalent to, but was deposited after the Keele Formation;
Teepee Dolostone, which is up to 30 m thick and caps the Hay Creek Group.
Sheepbed Formation, which is up to 900 m thick, and is part of the Windermere Supergroup. It overlies the Hay Creek Group and is almost entirely composed of dark shale deposited in a deep-shelf environment. It is overlain by the
Gametrail Formation, an up to 320 m thick dolomitised carbonate cap, which comprises two main facies, the first of which is a platformal suite to the NE, characterised by stromatolites and grainstones, and a southwestern, slope facies of dolomitised, ribbon-bedded limestones with debris-flow breccias.
Blueflower Formation, is up to 1000 m thick, and largely composed of dark shales and thin turbiditic sandstones. Sandy limestones and lime
mudstones occur in the lower part, carbonate-boulder debris flows appear near the middle, whilst medium- to thick-bedded and crossbedded sandstone is found towards the top.
Risky Formation, which is up to 150 m thick in the Mackenzie Mountains, and is completely dolomitised, such that the original fabric is only poorly preserved. Ooid and oncoid grainstones are prominent and stromatolite and thrombolite bioherms occur in the lower part. It lies immediately beneath a pronounced sub-Cambrian unconformity, which is overlain by the Ingta Formation which straddles the Neoproterozoic-Cambrian boundary (Narbonne and Aitken, 1995).
The oldest unit within the Selwyn Basin, the Late Neoproterozoic to Lower Cambrian Hyland Group, locally rests on the Ediacaran Blueflower Formation of the Windermere Supergroup on the northern margin of the basin, although elsewhere its basal sections may be equivalent to the Gametrail Formation. Overall though, the Hyland Group is a correlative of the Risky and Ingta formations and includes the Vampire Formation detailed below (Moynihan et al., 2014). In general, it comprises a sequence of ~3 km of gritty, quartzose clastic rocks, passing up into a limestone unit (Yusezyu Formation), overlain by ~750 m of maroon to dark grey shales (Narchilla Formation) and the laterally equivalent grey phyllites of the Vampire Formation.
NEOPROTEROZOIC TECTONICS AND DEPOSITION
The Mackenzie Mountains Supergroup represents a period of rift controlled sedimentation. Growth faults are mapped that displace contacts within this supergroup, but are terminated against the unconformity at the base of the Windermere Supegroup Rapitan Group, whilst other similar faults persist into the Rapitan Group. Abrupt thickness variations of Mackenzie Mountains Supergroup strata are interpreted to reflect control by regional structures with loosely-constrained NE-SW trends, as described below (Turner and Long, 2008).
A period of episodic rift activity, known as the Hayhook Extensional Event, began during deposition of the mid to upper Little Dal Group, and continued through the Little Dal Basalts and the Coates Lake Group to the Rapitan Group (Jefferson and Parrish, 1989). This extension reflects the early stages of the Rodinia supercontinent breakup. The Coates Lake Group is best developed in a series of embayments or basins on the flanks of the main NW-SE elongated zone of rifting, separated by much thinner accumulation of basin-margin facies rocks or discontinuities due to structural breaks (Jefferson, 1983). The embayments are, from north to south: the Mountain River (~100 km NW of Keele River), Hay Creek, Keele River, Hayhook, Coates Lake and Thundercloud Ranges (~50 km SSE of Coates Lake) embayments. The rift basins and embayments in which the Coates Lake and Rapitan groups were deposited are related to ENE-WSW to NW-SE oriented synsedimentary normal and related transfer faults (Eisbacher, 1981). On the basis of palaeocurrent directions and location of growth faults, the provenance of the Redstone River Formation detritus is interpreted to have been from local scarps, whilst sedimentary structures throughout the Coates Lake Group imply frequent synsedimentary seismicity. Deposition of the rift-controlled lower Rapitan Group marked the final episode of the Hayhook Extensional Event (Jefferson and Parrish, 1989). The rift sequences were followed by the more areally extensive shelf, slope and platform strata of the Hay Creek Group, deposited throughout much of the remainder of the Neoproterozoic (Narbonne and Aitken, 1995). The transition from rift to passive margin sedimentation reflects a change from an active extensional tectonic environment to a period of regional thermal sag along the western margin of Laurentia (Narbonne and Aitken, 1995).
In the Central Mackenzie Mountains, the major, arcuate, east vergent Plateau Thrust transposes the west dipping Mackenzie Mountains and Windermere supergroup over Palaeozoic sedimentary rocks to the east. This structure trends NW-SE in the north, to north-south in the south. In the southern section of the copperbelt, it fades and is replaced by parallel to en echelon structures ~20 km to the east. Further to the west, the Windermere Supergroup is overlain by the same Palaeozoic succession. To the east, a number of elongate belts of Neoproterozoic rocks of the Mackenzie Mountains Supergroup are exposed, separated by Palaeozoic rocks, occurring as anticlinal cores or thrust slivers.The Coates Lake Group and significant sediment hosted copper mineralisation are largely restricted to a corridor within 10 km to the west of the Plateau Thrust and its en echelon continuation to the south. In the immediate Coates Lake area, the Mackenzie Mountains Supergroup is thrust eastward over Devonian sedimentary rocks, while to the west the Neoproterozoic rocks are both overlain and overthrust by Palaeozoic successions.
The Plateau Thrust is thought to be of Late Cretaceous to Eocene Laramide age. Estimates for total displacement range from 35 km for an overthrust structural ramp-plateau model (Cecile and Cook, 1981) to just 6 km for a model calling for displacement along a sedimentary ramp that formed due to thinning or wedging of strata to the east (Gordey et al., 2011). It is likely the Plateau Thrust represents the reactivated of an earlier major extensional listric fault controlling Neoproterozoic deposition.
PALAEOZOIC
The Mackenzie Mountains and Windermere supergroups are respectively unconformably, and conformably to unconformably overlain by a late Neoproterozoic to Cambrian succession. From the late Neoproterozoic, a >100 km wide corridor to the east and NE of the current Plateau Thrust was a depositional high, known as the Mackenzie Arch, which constituted a westward inclined sedimentary ramp. This arch is a northwest trending locus of coalescing unconformities and stratigraphic thinning that divided Early and Middle Cambrian deposition into the Mackenzie Platform to the NE and the continental margin to deeper Selwyn Basin to the SW. Equivalent strata are missing or thin over the top of the arch. To the east, stratigraphic thicknesses increase, but only modestly, while to the west, thicknesses increase substantially into the Selwyn Basin (Morrow 1991). The arch persisted from the Late Neoproterozoic, where it appears to have influenced deposition of the Windermere Supergroup, and continued through the Ordovician to Late Devonian, and is part of a consistent depositional pattern along the Laurentian margin from Nevada to the Yukon (Gabrielse, 1967; Lund, 2008; Gordey and Roots, 2011).
During the Late Neoproterozoic to Lower Cambrian, the succession to the NE and east of the arch was dominated by quartz arenites of the Mount Clark Formation, passing upward and to the NE into more argillaceous rocks of the Mount Cap Formation, capped by shale, dolostone, anhydrite and halite of the Saline River Formation (MacNaughton et al., 2013). To the west of the arch, a thicker succession was composed of sandstones and quartzites of the Ingta, Backbone Ranges and Vampire formations, overlain by the basal siltstones and succeeding thick limestone and dolostone of the Sekwi Formation. This succession was deposited on a shelf-ramp along a passive margin, from coastal plain through continental shelf to continental slope (Dewing et al., 2006). These structural, depositional and facies variations imply a major syndepositional structure was present in the zone now occupied by the Plateau Thrust and its offset southern extension.
These rocks were transgressively to unconformably overlain by an Upper Cambrian to Lower Silurian succession that comprise the Mackenzie Trough/Platform, namely the Rockslide, Franklin Mountain, Sunblood/Duo Lake, Whittaker and Mount Kindle formations. These are predominantly above and to the east of the Mackenzie Arch, and are composed of dolostone, argillaceous dolostone, shale, siltstone, minor mafic volcanic rocks and local quartzite. They pass westward into the thicker deeper water silty limestones and calcareous siltstones of the Rabbitkettle Formation and the overlying carbonaceous, pyritic shales, argillaceous limestones and minor chert of the Early Ordovician to Mid Devonian deep water Road River Group in the Selwyn Basin.
These were followed by a Lower to Middle Devonian succession that includes limestone to dolomite with lesser sandstones to quartzites of the Camsell, Delorme, Sombre, Anica, Landry and Nahanni-Headless formations that also grade westward into the Road River Group. After a break, these are overlain by the Late Devonian to Lower Carboniferous marine to turbiditic shales, mudstones, siltstones and bituminous shales of the Imperial, Horn River and Besa River formations. These formations, deposited over and to the east of the Mackenzie Arch, are equivalents of the thick Earn Group in the Selwyn Basin to the west, which comprises grey to black chert and siliceous or cherty argillite, chert pebble-conglomerate, dark grey to black fetid limestone, black slaty, siliceous cherty argillite and local quartzite.
Stratabound Copper Mineralisation
Extensive, laterally continuous stratabound copper-silver mineralisation occurs throughout much of the length of the Redstone Copperbelt, predominantly localised in the Transition Zone (CP1 Member) between the Redstone River and Coppercap formations. The most economically significant of the three main deposit/occurrence clusters embraced by this mineralised halo is the Coates Lake or Redstone deposit, which outcrops over a strike length of ~7 km. It is confined to an embayment defined by a thickening of the host Coates Lake Group. This embayment was developed at right angles to the overall trend of the Mackenzie Mountains Fold Belt and was controlled by syn-depositional faults (e.g., the Basin Fault to the south of the deposit). The embayment appears to be bounded by fault scarp ridges of Little Dal Group sedimentary and volcanic rocks, the indicated source of the Coates Lake Group sediments, which thin and lens out against the margins of the embayment both to the NW and SE. Sedimentary structures indicate current directions from the NE, or up-dip. The Coates Lake Group progressively thickens and grades from fanglomerate to fine clastics from the NE to the SW. The Hayhook and Keele River occurrences are developed in the same unit in similar fault controlled embayments to the north.
The mineralised CP1 Member 'Transition Zone' at the base of the Coppercap Formation marks a gradation from the red, oxidised Redstone River Formation to the grey to black reduced upper Coppercap Formation. Mineralisation occurs as disseminated, veinlet and vein-hosted chalcocite, bornite and chalcopyrite. Pyrite, and to a lesser extent sphalerite and galena, are common in the CP1 Member distal to copper mineralisation (Jefferson, 1983). Copper and iron sulphides are found within seven, reduced, carbonate-evaporite beds (numbered B1 to B7 from the base), individually ranging from 0.2 to ~6.0 m in thickness (Ruelle, 1982), distributed over a stratigraphic interval of up to 110 m and separated by more oxidised rocks. These beds are composed of massive to laminated dolostone or dolomitic limestone, some of which are microbially laminated and exhibit laminoid to bird's-eye shaped fenestrae infilled with quartz, chert, dolomite or calcite. They also include massive beds or nodules of gypsum and anhydrite. Individual B1 to B7 beds may comprise multiple bands of reduced carbonate rocks, typically microbial laminites. The individual carbonate-evaporite bands within the bed are separated by dolomitic siltstone and mudstone. Each of the individual or composite beds is flanked immediately above and below by a green (weathering to tan) coloured fringe of chlorite-bearing reduced siltstone and mudstone. These reduced fringes, in turn, pass rapidly into an up to 15 m thick package of red, oxidised, hematite-bearing, siltstone, dolomitic siltstone, fine sandstone and mudstone which separate each of the seven reduced carbonate-evaporite bands. These oxidised intervals are of the same facies found in the underlying Redstone River Formation. The ratio of the comparative thickness of red to green siltstone and mudstone separating the carbonate bands decreases rapidly upwards, until a green colouration predominates between the third and fourth carbonate bands. The tan coloured reduced carbonate-microbial dolostone-evaporite beds can be seen on the accompanying image of the east face of Coppercap Mountain, each separated by red facies (Milton, 2015).
The bulk of the copper mineralisation at the Coates Lake deposit is localised within the lower three of the seven mineralised carbonate-evaporite bands. The lowest, B1, has the highest grade of 3.92% Cu and 11.3 g/t Ag over an area of just under 12 km2, but only averages just under one metre in thickness. B2 is thinner with similar grades while the third is thicker (around 5 m) but has a lower Cu grade. The upper four beds mostly only contain pyrite, whilst the intervening red-beds are completely devoid of sulphide mineralisation. The rocks that are mineralised are predominantly composed of microbially laminated dolostone or dolomitic limestone. The microbial laminae are generally thin and 'crinkled' although they have an overall, planar, bedding-parallel geometry. Green, dolomitic siltstones immediately adjacent to the microbial laminites may also host disseminated copper sulphides. Copper mineralisation in beds B1, B2 and B3 occurs as stratabound disseminated and fine veinlets of a hypogene assemblage of chalcocite, bornite and chalcopyrite (Milton, 2015).
There is a pronounced zonation of sulphides within the deposit, both across stratigraphy and laterally along individual beds. Sulphide suites transition via gradational boundaries from chalcocite-bornite → bornite → bornite-chalcopyrite → chalcopyrite → chalcopyrite-pyrite → pyrite. The lowest bed, B1, is dominated by an assemblage of chalcocite, with lesser digenite and bornite and only traces of chalcopyrite and pyrite. Never-the-less, there is a lateral zonation in a SSE to NNW direction within bed B1, from a chalcopyrite-bornite zone in outcrop to the SSE, progressively grading through chalcocite-bornite assemblages down-dip to the NNW to the chalcocite dominated bulk of the B1 mineralisation. Bed B2 has a similar assemblage, although bornite predominates, with an increased chalcopyrite content, including laterally peripheral chalcopyrite-pyrite and pyrite zones. Copper sulphides in bed B3 are dominated by chalcopyrite with chalcopyrite-pyrite and pyrite zones. However, mineral zonation in this bed tends to progress along stratigraphy from chalcocite or bornite dominated assemblages in the south, becoming more bornite or chalcopyrite rich towards the north with some local variations. This is the reverse of the lateral zoning of bed B1. The thickness of the mineralised zone within Bed B3 varies below Coppercap Mountain from south to north. Whilst the stratigraphic thickness of the Bed 3 microbial laminite is constant at ~6 m, the southern section has thicker intervals of copper mineralisation, up to 4 m, thinning to ~0.3 m some ~6 km to the north. Sulphide assemblages are also zoned on an outcrop scale across individual microbial laminite beds perpendicular to stratigraphy. On the southern section of Coppercap Mountain, Bed B1 has a central core dominated by bornite with zones of chalcopyrite forming an envelope stratigraphically above and below. At the lateral transition to the chalcopyrite dominant section of the bed, the central chalcopyrite zone is sandwiched by pyrite zones in the beds above and below. Beds B4 to B7 and to a lesser degree the intervening green/tan beds, contain only pyrite while Bed B8 has minor galena and sphalerite. (Ruelle, 1982; Jefferson, 1983; Chartrand and Brown, 1985). In the oxidised zone, hypogene sulphides often have superimposed oxidised rims or cracks with supergene copper minerals commonly developed, including malachite, covellite, cuprite, digenite and chalcocite, minor azurite and traces of native copper. Other hypogene sulphide minerals include abundant pyrite and minor galena (Milton, 2015).
Coates Lake deposit - Upper image: sample of the microbially laminated central core of the Coppercap Formation, CP1 Member, Bed B1. This sample is the weathered face of a surface sample. It has a well defined but 'wavy' bedding/banding with partially oxidised, elongated bornite-chalcopyrite crystals and crystal clusters as indicated by the arrowhead, elongated parallel to the banding.
Lower image: the broken fresh surface of the same sample. The bedding direction is indicated by the arrowhead. It can be seen to have abundant disseminated sulphides (e.g., as shown by second arrowhead), but also thin sulphide veinlets developed both parallel, normal and at an angle to bedding. Note the disseminated sulphide crystals are not elongated in this plane of the sample. The weathered surface is the upper edge of the sample in this image. Scale bar graduations are in cm and mm. Collected in 1991 and photographed by Mike Porter, 2018.
The zones of disseminated and veinlet sulphides are locally accompanied by bedding parallel veins of bornite-dolomite-calcite that have a maximum thickness of ~15 cm. Bed B1 is typically composed of one bed of microbial laminite containing disseminated and fine veinlet sulphides, sandwiched between two bedding-parallel veins, one above and the other below the microbial laminite. This relationship has been traced over strike lengths of 2 km. The veins follow bedding contacts between lithological units, particularly between microbial laminites and green dolomitic siltstones, and are typically found in direct contact with zones of disseminated copper sulphides. Bedding parallel veins are rarely found in Bed B3 and have not been observed in areas devoid of mineralisation. In areas of disseminated bornite mineralisation, 1 to 5 mm thick, and <20 cm long transgressive veinlets containing massive bornite are also found, steeply inclined to bedding. Within Bed B1 there is also and apparent zonation. For example, Milton (2015) illustrates an intersection where bornite is the dominant sulphide in the main stratabound disseminated mineralisation, the enclosing stratabound veins carry bornite and chalcopyrite, while the enclosing green dolomitic siltstone above and below contain chalcopyrite, and sulphides are absent from the progressively enveloping pink and dolomitic siltstone.
The textures and character of mineralisation in the Coates Lake area has been influenced by post-mineralisation deformation. This deformation has produced a pervasive cleavage that is locally developed in Redstone River Formation mudstones, the CP1 Member and in the Devonian shales in the footwall of the Coates Lake thrust. This foliation, which is defined in the deposit area by the development of Fe-hydroxides, has a consistent NNW-SSE strike and steep dip, and is axial planar to regional scale NNW-SSE trending folds observed by Gabrielse et al. (1973). Tabular, flattened sulphide grains are also aligned parallel to the foliation, as are local thin calcite-sulphide veinlets (Jefferson and Ruelle, 1986). En echelon sigmoidal tension gashes filled with coarse calcite and chalcopyrite have been observed. Strain shadows of fibrous calcite±rare chalcopyrite and bornite are formed on opposite sides of competent grains of authigenic quartz or feldspar within the CP1 Member (Milton, 2015).
The bedding parallel veins associated with Bed B1 have also been deformed to varying degrees. The original wall-parallel banding of carbonate and sulphide within these veins becomes increasingly obscured with deformation. Poorly deformed veins have a crystalline vein-fill composed of irregular bands of sulphide-carbonate minerals with stylolite bounded margins. Moderate deformation produces an anastomosing foliation defined by microlithons of carbonate minerals separating 10 to 20% spaced cleavage foliations of bornite-iron hydroxide, with shear textures including 's-c' like fabrics (e.g. Lister and Snoke, 1984). Strongly deformed veins comprise 50 to 60% by area of spaced cleavage domains containing fibrous bornite-iron hydroxides enclosing 1 to 5 mm augen composed of carbonate minerals and larger (up to 10 cm) wall rock fragments, resembling a durchbewegung texture. Deformation is apparently focussed along bedding planes and within the bedding parallel veining. This deformation is reflected by offset of transgressive calcite veining and slickensides on bedding surfaces. Otherwise there is little evidence of internal deformation of actual lithological units, such as the microbial laminites which are apparently undeformed with sedimentary laminae preserved (Milton, 2015).
Four mineral associations have been recognised at Coates Lake (after Milton, 2015):
• Pre-copper Association - which is recognised in microbial laminites distal to copper mineralisation. These rocks are composed of calcite, dolomite, quartz, K feldspar, albite, pyrite and gypsum occurring as variably rounded detrital grains with primary carbonate cements infilling the early porosity. The primary detrital silicate grains include quartz, K feldspar and albite, which have authigenic, euhedral overgrowths. Rounded detrital feldspar grains may have originally been plagioclase that has been replaced by albite or K feldspar during authigenic overgrowth, leaving no trace of the original mineralogy, as is suggested by the absence of any recognised proximal sources of detrital K feldspar or albite. Other less abundant detrital grains include zircon and rutile. Primary, fine grained calcite and dolomite cements are ubiquitous and occur as irregular, interlocking, typically 5 to 10 µm grains of yellow luminescent calcite or 5 to 10 µm rhombs of yellow-orange cathodo-luminescent dolomite (Dol1). Gypsum and anhydrite occur as nodules, laths or infill of fenestral porosity. Framboidal pyrite (Py1) typically comprises 5 µm diameter grains and clusters of framboids up to 25 µm across. Fine grained (submicron to 35 µm) disseminated euhedral cubes of pyrite (Py2) are common throughout Beds B3 to B7. The microbial laminae, which are defined by thin layers of organic matter, contain abundant Py2. On the basis of the framboidal texture of Py1 and the fine grain size of Py2 and remnants of inferred Py2 surrounded by chalcopyrite, both are included in the Pre-Copper Association. There is no direct data on the relative timing between Py1 and Py2. The detrital grain assemblage and primary carbonate cements detailed above are included as the primary association or stage 1A, which have been over printed by a stage 1B assemblage of authigenic sulphate (gypsum/anhydrite), carbonate and pyrite that post-date sedimentary deposition but pre-date copper mineralisation.
• Copper Association - which is characterised by the copper sulphides chalcocite, bornite and chalcopyrite as well as secondary albite, quartz, dolomite (Dol2), K feldspar, monazite, apatite and pyrite (Py3). Dol2 occurs as euhedral to subhedral, non to weakly cathodo-luminescent orange, grains between 30 and 900 µm that are coarser than Dol1 grains. Coarse copper sulphides and Dol2 are commonly interlocked, and Dol2 contains many solid inclusions of copper sulphides. Quartz, albite and K feldspar all occur as euhedral to anhedral grains of up to 450 µm diameter. From the deposit to thin section scale, either albite or K-feldspar may be the dominant secondary feldspar phase. Coarse grains of secondary quartz contain abundant solid inclusions of a copper sulphide assemblage containing chalcocite; chalcocite-bornite; bornite; bornite-chalcopyrite; or chalcopyrite.
Grains of copper sulphide vary from sub-micron disseminations, to semi-massive, irregular accumulations of mm-scale grains. Where two copper sulphide minerals occur together, sulphide-sulphide grain boundaries are frequently irregular, with common myrmekitic intergrowths of chalcocite and bornite, whilst in other areas basket-weave or gridded exsolution lamellae of bornite and chalcopyrite are evident. Copper sulphides may psuedomorph gypsum or anhydrite, and locally enclose quartz, albite, K feldspar and Dol2 but rarely calcite. Semi-massive copper sulphides locally enclose Dol2 and K feldspar without calcite, which is characteristic of mm-scale zones of the Copper Association copper sulphides-Dol2-quartz-albite-K feldspar assemblage. Apatite is found as zoned grains (defined by BSE brightness) and as solid inclusions aligned along zone boundaries. The rims of apatite grains are subhedral to euhedral with euhedral overgrowths of albite. Within mineralised rocks, monazite occurs as up to 50 µm grains with anhedral, subhedral or euhedral forms, which share euhedral straight edge grain boundaries with chalcopyrite grains. Chalcopyrite has been seen to completely enclose grains of monazite. Rare grains of silver telluride have been identified (semi-quantitative EDS analysis), whilst fine-grained sphalerite is locally evident in association with pyrite or pyrite-chalcopyrite. Sparse galena was observed accompanying bornite.
Fine-grained Py2 of the Pre-copper Association 1 is occasionally overgrown by chalcopyrite, with irregular grain contacts. Semi-massive chalcopyrite may also completely surrounding pyrite euhedra. Bornite has been observed completely enclosing irregular grains of pyrite or alternatively forming the core of pyrite crystals. Euhedral disseminated Py3 is common in zones of chalcopyrite mineralisation and adjacent pyrite-rich zones and is distinguished by it course crystal size (in contrast to Py2) and cubes with faces of up to 1 cm. Because of its coarse grain size and close spatial association with copper minerals, Py3 is included in the Copper Association, although there is no direct relative timing constraints on its formation.
The Copper Association assemblage is shared by both the bedding parallel veins and the immediately adjacent disseminated stratabound mineralisation at the Coates Lake deposit, although the veins predominantly contain bornite ± minor chalcopyrite, with chalcocite rarely being present in the veins.
The Copper Association assemblage replaced carbonate phases of the Pre-copper Association, as implied by the enclosure of other minerals of the former by copper sulphides and the marked absence of minerals from the latter within these enclosures. Milton (2015) proposed a dissolution-replacement mechanism for the development of the Copper Association assemblage, owing to the mm-scale of the enclosed features, which are too large and abundant to have originated from the infill of a pre-existing porosity.
• Deformation Association - which comprises an assemblage of calcite, bornite and chalcopyrite that formed within structural features generated during post-mineralisation deformation, e.g., cleavage, strain shadows and sigmoidal veins. This assemblage post-dates the Copper Association, as tabular grains of copper sulphides are orientated parallel to the foliation, interpreted to be the result of either flattening of pre-existing sulphides or dissolution-precipitation.
• Weathering Association - is the result of chemical weathering and comprises an assemblage of malachite, covellite, chrysocolla, chalcocite and iron oxides, minor azurite and traces of native copper. Malachite occurs as radial splays of well formed needles infilling porosity, and is associated with sulphides and iron oxides. Covellite rims bornite and chalcocite crystals with iron oxides. At Coates Lake, malachite is the dominant secondary copper mineral whereas throughout the rest of the Copperbelt, azurite dominates with lesser malachite, chrysocolla and iron oxides. In places, weathering has obliterated all sulphides at surface, particularly where primary chalcocite or bornite dominated.
In addition to the pattern of distribution of sulphides described above, there is also a zoning of the secondary 'alteration' assemblages of K feldspar-albite and dolomite-quartz-calcite with similar trends in beds B1 and B3. There is a relatively high abundance of K feldspar in the south, decreasing to the north in both beds, whereas albite dominates the north and decreases southwards. A similar antithetic relationship is observed between calcite and dolomite. In the main central section of the Coates Lake deposit, there is a higher proportion of dolomite and lesser calcite whereas at both the northern and southern extremities, calcite is the more common carbonate mineral. However, changes in the ratio of K feldspar:albite is not spatially related to the boundaries or distribution of sulphide mineral zones, e.g., the distribution of these two feldspar is similar in both beds B1 and B3, while the sulphide zonation from chalcopyrite to chalcocite-bornite in Bed B1 is the reverse of that in Bed B3, as detailed above. There is no systematic spatial relationship between zones of sulphide minerals and changes in relative abundance of feldspars. In areas that are many kilometres removed from stratabound copper mineralisation, authigenic albite is common in the Transition Zone between the Redstone River and Coppercap Formations, but authigenic K feldspar is less so (Milton, 2015). Jefferson (1983) reports the common occurrence of authigenic albite throughout the Redstone River Formation sandstone and siltstone. This is consistent with the interpretation that K feldspar and albite are Pre-Copper Association authigenic minerals, whilst much of the dolomite (Dol1 and Dol2) are part of the Copper Association.
The preceeding has concentrated on the Coates Lake area where the bulk of exploration has been concentrated. Less detail is known of the other areas of recorded stratabound copper mineralisation, which may be summarised as follows:
• Hayhook Embayment
The Hayhook Embayment is located ~80 km NNW of Coates Lake and includes >10 known copper occurrences distributed over a strike length of ~15 km, hosted within the Redstone River-Coppercap formations transition. Unlike at Coates Lake, but similar to Keele River, there is only a single cryptalgal-laminated unit that is as much as 8 m thick within the CP1 Member. Much of the prospective stratigraphy in the Hayhook Basin is masked by glacial cover. Exposures of high grade chalcocite-rich dolostones have been recorded, as per the accompanying image, returning assays of >10% Cu, >15 g/t Ag (Copper North website, viewed 2018).
• Keele River Embayment
The Keele River Embayment is some 160 km to the NNW to NW of Coates Lake. Stratabound Cu occurrences are distributed over a strike interval of >50 km within rocks of the Redstone River-Coppercap formations transition. Three main prospects have been investigated, June Creek, Jay and Hutch. Mineralisation occurs over narrow lateral widths of ≤100 m (parallel to bedding), but for distances of kilometres (along strike), and is controlled by abrupt facies changes in places and cuts facies boundaries elsewhere. Drill-indicated thicknesses as great as 52 m, with grades of 2.3% Cu were intersected at June Creek, with an average thickness of 12.5 m. The average grade is 2.7% Cu at the Jay prospects (Ruelle, 1982). Resources at Jay are 1.2 Mt @ 2.7% Cu; at June Creek 0.25 Mt @ 3.4% Cu (Ruelle, 1982).
• Coates Lake Extensions
Exposed mineralisation has been investigated to the north and south of Coates Lake. At North Redstone, which is 40 km to the north of Coates Lake, an ~2 m wide quartz vein containing chalcopyrite and bornite is hosted in a limestone unit immediately below the Rapitan Formation. This same limestone unit also contains other zones of disseminated chalcopyrite-bornite in the same area. Hidden Valley, located 26 km to the north of the Coates Lake deposit, contains tetrathedrite and chalcopyrite and pyrite + bornite and galena, and is described below. South Redstone, 53 km south of Coates Lake (also known as the McBean leases) contains abundant galena, sphalerite and pyrite mineralisation, but only rare copper minerals, and is hosted in limestone and dolostone, capped by a prominent gossan. Drill hole to depths of <50 to 110 m remained in gossan with no sulphides encountered.
Formation of Stratabound Copper Deposits
Interpretations of the origin, distribution and controls of mineralisation within the Redstone Copper Belt, from its discovery to recent times, have been synthesised by Milton (2015), as follows. Previous genetic models for stratabound Cu mineralisation in the Redstone Copperbelt have suggested that the mineralisation occurred during early diagenesis (Coates, 1964; Ruelle, 1982; Jefferson, 1983; Jefferson and Ruelle, 1986; Chartrand and Brown, 1985; Lustwerk, 1990). Jefferson (1983) interpreted sulphide grains as occupying early diagenetic pore space and replacing diagenetic minerals. Chartrand et al. (1989) proposed that copper minerals formed after an early diagenetic assemblage of gypsum-anhydrite-dolomite-calcite-pyrite-quartz-feldspar but within the period of early diagenesis. This interpretation was based on petrographic observations of copper sulphides interstitial to diagenetic minerals and having replaced or enclosed diagenetic minerals. Chartrand et al. (1989) proposed that mineralisation occurred within 100 m of the sediment-water interface, significantly before the deposition of the Rapitan Group. They based this interpretation on the preservation of fenestrae in uncemented microbial-laminated carbonate rocks following burial. They argued that fenestrae were subsequently filled with copper sulphides rather than compacting during deeper burial. However, Milton et al. (2017) suggest cupriferous sulphides did not fill open pore spaces in fenestrae, but rather replaced earlier diagenetic infill that had preserved the noncompacted fenestrae shape. Lustwerk and Wasserman (1989) asserted that water escape structures, some of which are filled with pyrite or copper sulphides, within the Redstone River Formation red siltstones are the product of mineralising fluids that escaped from the sediment pile and migrated upwards towards the Transition Zone during compaction and early burial. The underlying red-beds of the Redstone River Formation were formed in part from the copper-rich mafic detritus eroded from the Little Dal Basalts (Lustwerk, 1990). Depletion of copper in the red-beds relative to typical crustal or sedimentary rock, and the common association of thick sequences of red-beds with areas of stratabound copper mineralisation suggest that the red-beds were the likely source of metals in mineralising fluids (Lustwerk, 1990). Transport of copper was mediated by an oxidised chloride brine, which may have moved upward and landward away from the basin (Jefferson and Ruelle, 1986). Progressive reduction in chemical traps was accomplished through a generally upward migration of the fluid across the Transition Zone (CP1 Member). Stratiform copper mineralisation is not confined to the CP1 Member and has been observed at many stratigraphic intervals, including: i). the dolostone in the TH4 Member of the Thundercloud Formation (Jefferson, 1983); ii). CP7 microbial laminites at the top of the Coppercap Formation south of the June showing at Keele River (these are host to disseminated tetrahedrite, chalcopyrite and pyrite); and iii). the Sayunei Formation at many minor showings between the Coates Lake and Hayhook embayments. Throughout the Redstone Copperbelt, minor disseminated chalcopyrite can commonly be found in sandstones at the base of the Sayunei Formation, just above the contact with the underlying Coppercap Formation. Coarse euhedral pyrite is also present throughout much of the CP1 Member and overlying Coppercap Formation and locally the Rapitan Group.
Milton (2015) and Milton et al. (2017) have constrained the age of mineralisation relative to the depositional age of the host sedimentary rocks by in situ U-Th-Pb chemical dating of detrital monazite grains associated with mineralisation at the Coates Lake stratabound copper deposit. The monazite grains have rounded, Th-U-heavy rare earth element (HREE) rich, detrital cores that are surrounded by Th-U poor, light rare earth element-S-Sr rich rims. The rim stage of monazite growth is observed to be intergrown with and enveloped by cupriferous sulphide and is paragenetically constrained as being part of the disseminated copper mineralising event. Eleven detrital cores yielded dates of between 1843 and 1025 Ma, older than the depositional age of the CP1 Member, host to mineralisation, which has previously been constrained to be between 775 and 732 Ma. Monazite rims from 10 samples yielded dates between 661 and 607 Ma, giving a weighted average of 635±13 Ma which is regarded as a maximum estimate of the absolute age of copper mineralisation at the Coates Lake deposit. As such, mineralisation would have formed during the thermal sag phase of continental rifting, ~100 m.y. after deposition of the host rocks. This is calculated to have followed 2 to 4 km of burial below the upper Coppercap formation and deposition of the Rapitan Group Ice Brook Formation glacials, which are equated with the global ~635 Ma Marinoan cryogenic event. Milton et al. (2017) address the possibility this age may represent the last of a series of earlier to diagenetic overprinting Cu mineralising events and present observations to suggest that whilst deposition of Py1 and Py2 may have been early diagenetic, there is no evidence to indicate earlier Cu mineralisation. They suggest the sulphur required for cupriferous sulphide growth could have been derived directly from the replacement of early diagenetic pyrite and/or by thermochemical reduction of the authigenic sulphates within the hosts, rather than by bacterial sulphate reduction during early diagenesis.
Deposition of the Redstone River and Coates Lake groups took place in an elongate rift basin during a period of episodic intracontinental rifting heralding the breakup of the supercontintent Rodinia. This rifting transited through the period of Rapitan deposition into areally extensive sag-phase sedimentation over the interval in which the Hay Creek Group was laid down. The latter marked the end of rift faulting, ~30 m.y. prior to the Cu mineralising event at ~635 Ma. The shales of the 662.4±3.9 Ma Twitya Formation at the base of the Hay Creek Group formed an affective aquitard above the rift phase sequences and the inactive rift faults they truncated. The inactive rift margin faults are considered likely to have been sealed and healed very early within the ensuing 30 m.y. (e.g., Tenthorey and Cox, 2006; Giger et al., 2007). Milton et al. (2017) demonstrate that the ~1.4 km of Rapitan Group and 300 m of Coppercap Formation (estimates of the decompacted thicknesses at 635 Ma), especially the reduced shaly limestones of the CP2 to CP5 members, would have formed a low-permeability cap over the host CP1 Member. Below the CP1 Member, the relatively clean, red, oxidised siltstone and fine sandstone of the Redstone River Formation RR4 facies would have had a higher permeability, aided in part by the ubiquitous sandy dewatering structures crosscutting silty bands between sand layers and by the basin margin fanglomerates of the RR2 facies.
The reddening of the basin sediments of the Redstone River and Thundercloud formations is interpreted to be the result of protracted influx of cool, strongly oxidised (Eh=~0.4 v), slightly acidic, meteoric fluid following deposition, but prior to emplacement of the Coppercap Formation. These fluids are interpreted to have progressively oxidised detrital iron-bearing silicate minerals derived from the Little Dal Group mafic volcanic rocks (e.g., Walker et al., 1981; Walker, 1989). This oxidation also resulted in the liberation of Cu from the lattice of detrital grains. Subsequent scavenging and transport of Cu to the site of deposition, requires high salinity brines to promote solution of Cu previously liberated during the oxidation of the sedimentary sequence. These brines must have optimum Eh (~0.0 to 2.0 v) and pH (~7) levels to dissolve liberated Cu. Significant copper solubility also requires a sufficient salinity and available ligands for soluble copper-chloride complexes to form (Brown, 2005). During early diagenesis, salinity is likely produced by leaching of the abundant evaporites, ±mixing with evaporated seawater sinking downward from evaporite layers (e.g., Walker, 1989; Brown, 2005, 2009). The two significant glacial episodes at ~717 to 660 Ma (Sturtian) and ~635 Ma (Marinoan) may have also produced cryogenic brines that are a potential source of salinity for mineralising fluids. Major global glaciation events freeze seawater at the sea-ice interfaces to produce pure H2O ice and the precipitation of mirabilite (Na2SO4•10H2O), leaving behind large volumes of relatively oxidised residual hypersaline brine (Herut et al., 1990). The dense hypersaline brine has a depressed freezing point and migrates downward into the basin through permeable sediment or fractures displacing lighter fresh pore waters, a process known as 'diagenetic brine reflux'.
The presence of bedding-parallel veins accompanying the disseminated and veinlet mineralisation in the CP1 Member indicates the development, at least transiently, of a tensile effective stress that was normal to layering in that member. Such stresses require pore fluids that are overpressured to the point of being supralithostatic at that location. To operate, this process needs a fluid connection to deeper parts of the basin where lithostatic pressure is greater, and is transmitted hydraulically to the level of a seal that precludes further upward expulsion of that fluid. This supralithostatic overpressure fractures, delaminates and inflates the sequence below an aquitard seal, preferentially along planes of weakness, often bedding planes, competancy contrasts and pre-existing fractures. This is natural 'fracking'. It permits enhanced access of overpressured mineralising fluids to permeate the sequence and provides space in which to precipitate bedding parallel veining as well as narrower fracture and veinlet mineralisation. This delamination of the sequence along bedding planes, and the bedding parallel veining that was introduced, provided a focus of strain during post-ore deformation.
Milton et al. (2017) present observations and evidence suggesting the key driving force of cupriferous fluid flow at Redstone was 'free convection' within the Thundercloud and Red River formations, driven upward by the thermal and lithostatic pressure gradient and downwards by thermohaline convection. They also suggest convective upflow was focused at the southern end of the Coates Lake basin, adjacent to inferred rift margin faults, e.g., the Basin Fault (see Coates Lake map). As the cupriferous fluid moved up, it was focused into and transmitted internally along the CP1 Member. The zoning patterns of Cu sulphides suggest the following: i). chalcocite to pyrite from beds B1 to B3 implies Cu was introduced from below with the bulk of Cu in the first reduced sulphate rich bed encountered; ii). zonation within Bed B1 from bornite in the microbial laminites of its core, to chalcopyrite selvedges in the enclosing dolomitic shales above and below suggest transmission of fluids along the overpressured core of the bed; the development of conformable mineralised veins on both margins of this core also support overpressuring, delamination and inflation along the core of Bed B1 and ; iii). chalcopyite to chalcocite-bornite zoning from SSE to NNW within Bed B1 suggests fluids flowed within that bed from the NNW, while the reverse in Bed B2 suggests a SSE source, such as from the Basin Fault.
On the basis of the observations above, as well as mapping, fluid inclusion, paragenetic and S-Sr-O-C isotope evidence Milton (2015) concluded that the mineralising fluids were introduced at ~190 to 250°C, had salinities of ~32 wt.% NaCl equiv., were overpressured and had been circulated by free convection through red-beds of the Thundercloud and Redstone River formation that underlie mineralisation and are the implied source of scavenged Cu.
Order of magnitude mass balance calculations suggest that: i). if the ~1.3 Mt of Cu in the Inferred Mineral Resource at Coates Lake represents 5% of the Cu in the 200 km long Redstone Copper Belt, which would therefore contain ~25 Mt of Cu; ii). the Cu content of the Thundercloud and Red River formations sedimentary rocks (SG of 2.5) derived from the Little Dal Basalts is 100 ppm and 50 ppm elsewhere; iii). ~50% of that Cu, i.e., ~30 ppm is scavenged transported and precipitated in the CP1 Member, then ~330 km3 of those sequences would have had to be scavenged to provide the required Cu.
Mineral Resources - Stratabound Copper Deposits
The Redstone/Coates Lake Cu deposit has inferred resources as follows, based on broad spaced drilling (Hildebrand, 1978; Gourlay, 2005):
• 33.4 Mt @ 3.92% Cu, 11.3 g/t Ag over the actual average 0.98 m thickness of the 'ore bed', or,
• 61.7 Mt @ 2.13% Cu, 5.5 g/t Ag when diluted to a mining width of 1.83 m.
Vein-Vug and Breccia Copper-Pyrite Mineralisation
In addition to the stratabound mineralisation, there are a number of vein-vug occurrences developed over the length of the Redstone Copper Belt mostly in the hanging wall of the Plateau Thrust and parallel structures representing its southern extension. These occurrences have a characteristic mineral assemblage of coarse, sparry, dolomite-quartz-calcite-copper sulphide-tetrahedrite-pyrite forming a cement infilling breccias, veins and irregular vuggy cavities. The presence of tetrahedrite, absence of primary chalcocite, and the vuggy, coarse and sparry nature of the mineral associations distinguishes these occurrences from the stratabound copper mineralisation. Mineralisation is closely associated with dolomitisation and is hosted by the middle section of the Coppercap Formation of the Coates Lake Group, particularly the CP3, CP4 or CP5 members, and in permeable grainstones of the Little Dal Group Silverberry or Gayna formations. Locally, dolomite-quartz-calcite-pyrite vugs are developed with no accompanying copper minerals (Milton 2015).
The key occurrences are Johnson Vein, Hidden Valley and Colwell No. 1 which occur within the development of the Mackenzie Mountains Supergroup between Coates Lake and Hayhook; Hayhook B on the southern margin of the Hayhook Embayment and Bart on the southern margin of the Keele River Embayment, whilst a further cluster is found on the northern margin of the latter embayment.
These occurrences may be summarised as follows (after Milton, 2015):
• Hayhook B - is located in close proximity to known stratabound Cu mineralsiation towards the southern margin of the Hayhook Embayment. Chalcopyrite mineralisation occurs within a network of irregular dolomite veining that cements an angular discordant breccia of fragmented and dolomitised carbonate rocks of the Coppercap Formation. This brecciation is concentrated within the CP3 Member, but extends from the top of CP2 to the base of CP5. The occurrence is located in the hangingwall of a north-south trending, steeply dipping extensional fault that juxtaposes the CP2 Member against the Redstone River Formation. The breccia cement is composed of abundant coarse crystals of white dolomite and occassional euhedral prismatic quartz crystals that are several cm long. This dolomite-quartz-chalcopyrite assemblage also occurs in vuggy cavities up to 15 cm wide that are distributed irregularly throughout the host rocks adjacent to the brecciated zones. Both the enclosing host rocks and breccia clasts have been subjected to extensive recrystallisation and pervasive dolomitisation to produce a very fine grained irregular, interlocking mass of dolomite crystals. The bulk of the coarse, euhedral grains of the breccia cement dolomite have a cloudy appearance in thin section due to the presence of abundant submicron fluid inclusions and solid mineral inclusions of chalcopyrite. The rims of these crystals, in contrast are clearer, reflecting a lower density of fluid inclusions. Chalcopyrite is the last phase to fill porosity in dolomite-lined vugs. Rare quartz and later calcite has been observed growing on euhedral dolomite crystals in vug-cavities.
• Johnson Vein - is hosted in the Little Dal Group, ~50 km north of Coates Lake and 35 km SSE of Hayhook B. The main vein is described as being near 2 m wide, enclosing up to 65 cm of massive bornite-chalcopyrite-geothite, striking at 95° (roughly normal to bedding of the country rock) and dipping 85°, fringed by disseminated sulphides in the surrounding wallrock. Near surface the sulphides have been oxidised to iron oxides, azurite and
malachite. Mineralised talus has been traced for ~500 m to the ESE along trend from the main trenched exposure. Mineralisation occurs as angular fragments of brecciated carbonate rock cemented by coarse crystals of dolomite, barite, chalcopyrite and bornite with minor tetrahedrite. The enclosing Little Dal Group host rocks are pink, orange or light grey and dolomitic immediately adjacent to the mineralised structure, but are medium grey and calcareous some 10 m outward from the vein margin. The dolomite in the vein wall rock is much coarser than calcite and dolomite found 15 m away from the vein. The mineralised breccia is composed of isolated, angular clasts of Little Dal Group wall rock cemented by coarsely crystalline dolomite crystals that are as much as several mm in size and have crystal boundaries that are irregular but interlocking. Quartz is rare. Coarse chalcopyrite and minor bornite occur in the cement, as do finer grains of chalcopyrite, typically 5 µm in diameter. Three generations of dolomite veining have been recognised. The first two form a pervasive network of cross-cutting veins typically spaced at 1 to 5 mm, followed by a late vein episode of iron-rich dolomite with minor quartz. All generations of veins are cross cut by weakly developed stylolites (after Milton, 2015).
• Colwell No.1 - is ~8 km NNE of the Johnson Vein and is exposed in historic exploration trenching, hosted by carbonate rocks of the Little Dal Group. The unaltered host, 20 m from the mineralisation, comprises massive, fine grained carbonates and peloidal grainstones with minor pyrite. Mineralisation occurs as vuggy cavities lined with coarse crystals of dolomite, quartz and tetrahedrite within zones of coarse dolomite that are several metres wide containing lenses of massive tetrahedrite, some of which are over a metre long. No structural control is evident and the cavities are patchily distributed throughout the host sequence. Coarse crystals of brownish-red rutile, up to several mm long, are intergrown with the coarse dolomite in the cavities and in the adjacent dolomitised country rock. The host rocks adjacent to the mineralisation are recrystallised to coarse dolomite, and may also be brecciated. Where brecciated, the host rock can contain dolomite-quartz±tetrahedrite±rutile cement with minor chalcopyrite. The cements are coarse, with crystals several mm across. Stylolites cross-cut the breccia and carry concentrations of white mica, rutile, tetrahedrite, fine-grained pyrite and iron oxy-hydroxides.
• Hidden Valley - which is ~15 km SE of Johnson Vein, 30 km north of Coates Lake, but only ~10 km north of the Coates Lake Northern Extension strabound Cu prospect. It comprises numerous copper showings hosted in dolostone and limestone rocks of the Little Dal Group, and is located within 100 to 200 m of a number of inferred faults with displacements of hundreds of metres. Mineralisation occurs within either vugs lined with coarse
dolomite-quartz-barite-tetrahedrite-chalcopyrite, or within breccia composed of angular wallrock fragments cemented by dolomite-barite-tetrahedrite-chalcopyrite. Successive generations of dolomite crystals up to several mm across were formed within the breccias and vugs, accompanied by minor amounts of euhedral quartz, and are overprinted by tetrahedrite, and subsequently by trace amounts of copper sulphides, more rarely, as spectacular pods. Tetrahedrite also occurs in irregularly distributed pods as much as a metre in length containing up to 35% Cu, 1800 g/t Ag, 0.65% Zn and 0.45% Bi. Veins, vugs and breccias are irregularly developed and approximately follow bedding. Dolomite-barite-quartz-pyrite veins, breccia cements and vugs, devoid of copper minerals also occur widely throughout the prospect area.
• Bart - is located on the southern margin of the Hayhook Embayment, ~50 km NNW of Hayhook B and is one of a number of this style of mineralisation within an 80 km long interval of the embayment. It comprises breccias or coarse vuggy occurrences of dolomite-quartz-tetrahedrite with minor chalcopyrite, developed within limestones of the middle Coppercap Formation, towards the top of the CP4 Member. The host rocks have been pervasively dolomitised adjacent to brecciated areas, with multiple generations of euhedral medium grained dolomite, differentiated on the basis of texture and colour. Tetrahedrite is the principal copper phase in the breccias and vugs, intergrown with minor chalcopyrite. Copper minerals either form straight edge contact with coarse euhedral dolomite or less commonly can be seen to embay dolomite crystals particularly on vug cavity edges where tetrahedrite replaces dolomite crystals along cleavage planes. Another example, 1.5 km to the east has spectacular developments of coarse dolomite-quartz-pyrite in vugs and veins within the Coppercap Formation. Vugs are up to 30 cm in diameter, with much of the void-space remaining empty in the larger vugs. Successive generations of pink-weathering, white and grey dolomite form the outermost vug lining, followed successively by opaque white quartz, clear colourless quartz, pink to orange weathering coarse dolomite and finally dodecahedra of pyrite up to 5 mm in diameter.
Open-space mineral growth and infilling of cavities within the carbonate host rocks at these occurrences is suggested by the coarse, sparry nature of many of the minerals and residual vuggy porosity. In addition, episodic pulses of fluid are indicated by repeated generations of dolomite and quartz lining vugs, whilst an evolution over time of the fluid composition, chemistry and/or ambient physical conditions (e.g. temperature) is suggested by subtle variation in mineral chemistry, colour and texture of successive generations of quartz and dolomite (Milton 2015).
The host carbonate rock is pervasively dolomitised adjacent to zones of mineralisation, overprinting and obliterating all trace of the original sedimentary fabric of the host to produce dolostone or limy dolostone. Hidden Valley and Hayhook B are found in close proximity to faults whilst the Johnson Vein is hosted by a steep discordant structure, suggesting mineralisation occurred with or following a period of brittle deformation. The occurrence of a similar suite of dolomite-quartz-pyrite without copper sulphides in veins and vugs in areas surrounding these copper showings suggests that copper mineralisation occurred locally within a spatially more extensive system of vuggy dolomitisation (Milton 2015).
Rutile crystals with solid inclusions of euhedral dolomite and quartz have been dated at 395±63 Ma (U-Pb dating) in the mid-Palaeozoic at Colwell No.1 (Milton, 2015). As quartz and dolomite of the same generation are associated with the copper sulphides, the rutile is interpreted to have formed coevally with the vein-vug Cu mineralisation, 240 m.y. after the Neoproterozoic stratabound Cu.
Fluid inclusions suggest the fluids associated with mineralisation were weakly acidic and reduced, and had total salinities in the range of ~20 to 23 wt.% NaCl equiv.. There is a variation in the temperature of these fluids between the main occurrences, ranging from ~260 to 190°C, although, in general, Colwell No.1 and Bart have higher primary homogenisation temperatures of ~250°C whereas the Hayhook B and Johnson Vein are centred around 200°C (Milton 2015). Sulphide δ34S values from vein-vug mineralisation are as high as 19.5‰ largely precluding an igneous source of sulphur, but imlpying a source from evaporite reservoirs (13.9 to 17.7‰ Little Dal Group; Turner, 2009; mid-Palaeozoic seawater sulphate, from ~14 to 30‰ Strauss, 1997). The range of δ13C values for coarse dolomite from each of the key vein-vug occurrences is consistent with that of the hosting carbonates, interpreted to reflect incorporation of local carbonate-rock sources of carbon or equilibration of the fluids with the host rocks during the formation of the coarse dolomites (Milton 2015). Similarly δ18O values of coarse vuggy dolomite is comparable to that of the host rocks, interpreted to be the result of partial or complete equilibration of mineralising fluids with the host rocks at the temperatures estimated for vein-vug mineralising fluids. 87Sr/86Sr isotope signatures of the different deposits is also consistent with interaction with sequences found in the wallrocks and footwall of their respective host sequence (Milton, 2015).
Milton (2015) details the data summarised above and interprets it to suggest the mineralising fluids may have originated from depths of 6 to 13 km or more, and interacted with Proterozoic siliciclastic rocks containing highly radiogenic Sr, to be deposited within overlying Neoproterozoic strata. At 395±63 Ma, the Redstone Copper Belt was within the Mackenzie Arch where it would have been unroofed by erosion to shallow depths of ~1 to 5 km. The open space and vuggy nature of the mineralisation also suggests emplacement t shallow depths. During this same period, large scale fluid cells were also circulating through the Selwyn Basin to the west to form regional scale Mid- to Late-Devonian sediment hosted base metal mineralisation e.g., the Macmillan Pass cluster (see below). It has been suggested the same extensional regional tectonic driver of that system may have also been in play in the Mackenzie Arch. A single grain of monazite at the Coates Lake stratabound Cu deposit was dated at 374±14 Ma (Milton, 2015), raising the possibility that a second pulse of Cu from this Palaeozoic event may have also reinforced grades in the older deposit.
Stylolites that post-date mineralisation, and other structural relationships suggest vein-vug mineralisation preceeded the Cretaceous-Paleogene age Laramide orogenic event that formed the Mackenzie Mountains fold and thrust belt, the major deformation event in the region (Gordey et al., 2011).
While producing spectacular mineralisation and being an integral part of the Redstone Copper Belt, none of these occurrences appear as yet to be large enough to be of substantial economic significance. However, Turner, Broughton and Brooks (2018) suggest they may be analogous to the Kipushi deposit in the Central African Copperbelt.
Sediment hosted Zinc-Lead ±Copper ±Silver Mineralisation
Sediment hosted deposits and occurrences of Zn-Pb, with or without Cu and Ag, are widespread, both within the Palaeozoic rocks of the Selwyn Basin to the west of the Redstone Copper Belt, and within the carbonate-rich rocks of both Neoproterozoic and Palaeozoic age on the Mackenzie Platform/Arch, overlapping the Copper Belt and its projected extension to the northwest.
The best examples in the central to eastern section of the Selwyn Basin are the giant Howards Pass deposits, hosted by the Early Silurian section of the Road River Group shale, chert and minor limestone succession; and the Macmillan Pass - Tom and Jason cluster in the Middle to Late Devonian 'Black Clastic' or Earn Group with mineralisation concentrated at the transition between clastic rocks, and the overlying black carbonaceous argillite and siliceous shale facies. These two deposit clusters appear to represent stratabound, late diagenetic mineralisation emplaced in poorly consolidated muds in the sub-sea floor (Goodfellow and Lydon, 2007). They are located ~120 km west of the Redstone Copper Belt and are significantly younger than the Neoproterozoic stratabound sediment hosted Cu mineralisation of the latter. Both of these stratabound Zn-Pb clusters define stratigraphic corridors of mineralised sedimentary rocks that may be traced over tens of kilometres.
To the NE, straddling the transition from the Selwyn Basin to the Mackenzie Platform, but mainly within the latter, there are >230 Zn-Pb (±Cu, Ag, Ba, Sb, Ga) occurrences, prospects and deposits that form the 'Mackenzie Mountains Zinc District' (Ootes et al., 2013) which extends for ~450 km from Gayna River in the NW to Prairie Creek in the south. These occurrences overlap the Redstone Copper Belt from south of Coates Lake to Keele River and continue parallel to the Plateau Thrust for another 200 km to the NW. Virtually all are 'carbonate hosted' occurrences within both Neoproterozoic and Palaeozoic rocks. They are characterised by a mineralogy that commonly includes sphalerite, smithsonite and galena, with variable amounts of barite and copper-sulphide/sulphosalts. Fluid-inclusion data indicate temperatures of formation from 160 to >200°C (Fraser 1996; Carriére and Sangster 1999; Gleeson 2006; Paradis 2007; Wallace 2009). Examples include:
• Gayna River - ~15 km NE of the Plateau Thrust and ~180 km NW of Keele River. Mineralisation is hosted in the Neoproterozoic Little Dal Group, within a 160 m thick dolomite unit of the lower Gayna Formation, which is found over a 30 kilometre strike length. It is preferentially developed within discontinuous cemented breccias, interpreted to represent disaggregated stromatolite reefs. The mineralised zone is characterised by breccia-cemented, gallium-rich sphalerite with lesser pyrite and hematite. The sphalerite varies from pale green to orange-red, filling fractures or locally in structurally-controlled massive beds, or disseminated within the dolomite. The historic (possibly exaggerated) resource is quoted at ~50 Mt @ >5% Zn+Pb (Hewton 1982; Dewing et al., 2006; Wallace 2009).
• Tic - which is located between the Gayna River and Bear-Twit occurrences, is hosted by the ~300 m thick, Lower Cambrian Sewkwi Formation that overlies the Hyland Group. This unit is composed of dolostone and sandy dolostone, overlain by dark grey, medium to coarsely crystalline, medium bedded dolostone with beds of oolites and oncolites. These are followed by the immediate host to mineralisation, a light grey, finely to coarsely crystalline dolostone with abundant vugs and solution collapse breccia, cemented by sparry dolomite, quartz and sulphide minerals, pyrite>sphalerite>galena. The breccias are locally continuous over up to 20 m widths within a 60 m interval. They are composed of randomly sized and oriented fragments of light grey dolomite set in a matrix of internal sediment or secondary dolomite and pyrite. The matrix may constitute 25% of the rock and hosts Pb-Zn mineralisation which occurs in coarse vugs, randomly oriented veins and stringers interpreted to be cement to solution breccias. Bedding parallel pyrite and sphalerite is found over a strike length of >4 km. One of the cluster of occurrences at Tic, the C Zone, is ~300 m long and 50 m thick enclosing a 15 m thick band of better Pb-Zn mineralisation (Dewing et al., 2006). Tic is one example of the entensively distributed occurrences, rather than a significant deposit.
• Bear-Twit, ~120 km SSE of Gayna River and ~80 km NW of Keele River, contains Zn-Pb-Cu-Ag in veins that cross-cut dolostone of the 350 to 425 m thick Upper Ordovician to Silurian Whittaker Formation (Dewing et al., 2006). The immediate host rocks comprises medium to dark grey weathering coarse grained dolarenite, stromatoporoid floatstone and cherty dolomudstone. The dolomite becomes more coarsely crystalline towards mineralisation. Eleven mineralised occurrences define a linear trend normal to strike over a distance of 135 m and width of 15 to 20 m. Mineralisation comprises red and white sphalerite, galena and tetrahedrite within a gangue of quartz, dolomite, barite and calcite. Smithsonite locally replaces sphalerite. Mineralisation occurs in bedding parallel and bedding normal veins and only rarely replaces or is disseminated within the host carbonate. The deposit cluster has a historic resource of ~7 Mt @ 5.4% Zn, 2.6% Pb, 17.1 g/t Ag, although the overall tonnage may be over-estimated (Dewing et al., 2006).
• Prairie Creek is located in the southern Mackenzie Mountains, ~150 km SE to SSE of Coates Lake. For a more detailed description, see the separate Prairie Creek record. The regional geology is dominated by a Late Ordovician to Devonian stratigraphy, deposited within a fault controlled palaeo-embayment of the Selwyn Basin into the Mackenzie Platform. In the deposit area, north-south trending faulting and folding is apparent, with the deposit being developed on the western flank of the fault-bounded, doubly-plunging, Late Cretaceous to Eocene Laramide Prairie Creek anticline. The Ordovician, Upper Whittaker Formation, which is composed of interbedded cherts and dolostones, occupies the core of the Prairie Creek antiform, and is overlain by the graphitic-shales/dolostones of the Road River Group. Four styles of base metal mineralisation have been recognised, the first three of which constitute the Mineral Resource in the Main Zone:
Quartz veining, with the most extensively developed being the Main Quartz Vein - that dips at 65°E, with a strike length of at least 2.1 km, vertical extent of >600 m and thickness of from 0.1 to 5 m, averaging 2.7 m. This interval is part of a mineralised trend that can be traced for ~10 km along the Prairie Creek Fault. Mineralisation comprises massive to disseminated galena and sphalerite with lesser pyrite and tennantite-tetrahedrite in a quartz-carbonate-dolomite matrix. The vein is best-developed in the more competent units of the Lower Road River Group and Whittaker Formation, and dissipates in the graphitic shales of the mid and upper Road River Group. Offsets observed within this vein may represent a more complex en echelon structure rather than a simple single vein.
Stockwork Mineralisation, which occurs as a localised development of a series of 0.1 to 0.5 m thick, sub-vertical, massive sphalerite-galena-tennantite veins that are separated by unaltered dolostone. These veins, which are not a stockwork, strike at ~40° to the Main Quartz Vein, and are interpreted to be splays from the latter.
Stratabound Mineralisation, which has been intermittently intersected over a strike length of ~3 km within the Mottled Dolomite unit of the Upper Whittaker Formation. Mineralisation totally replaces the host without any significant alteration. It is generally fine-grained, banded to semi-massive and comprises massive bands of fine-grained sphalerite, coarse-grained galena and disseminated to massive pyrite. Silver is in solid solution within both galena and sphalerite and the mineralisation is devoid of tennantite-tetrahedrite. It has very little Cu and ~50% of the galena, but has substantially more iron sulphide/pyrite compared to typical vein material. Fragments of this mineralisation style has been found in vein material.
Carbonate Hosted Mineralisation, occurs discontinuously within coarse biohermal reefs of the Silurian Root River/Cadillac Formation that immediately overlies the Road River Group. It occurs ~6 km NE of the Main Zone deposit, and comprises colloform rims of sphalerite, brassy pyrite-marcasite and minor galena, with or without later dolomite infilling. No mineralisation of this style that is of economic interest has been found. This description is largely after Smith et al. (2005).
Measured + Indicated + inferred Mineral Resources are 15.753 Mt @ 10.3% Zn, 8.4% Pb, 149 g/t Ag (Canadian Zinc Corporation website, viewed Oct. 2018).
Discussion
The deposits and occurrences within the Mackenzie Mountains Zinc District occur within a range of host-rock ages, and have differences in mineralogy and alteration, as well as representing an array of mineralisation styles, even within individual occurrences. An example of the latter is Prairie Creek with economic stratabound Zn-Pb sulphides hosted by Late Ordovician to Silurian rocks, overprinted by both carbonate hosted and later, Ag-rich vein style mineralisation (Fraser 1996; Paradis 2007). Ootes et al. (2013) point out that the mineralogy of these diverse deposits commonly includes sphalerite, smithsonite and galena, with variable amounts of barite and copper-sulphide/sulphosalts. Fluid-inclusion data indicate temperatures of formation from 160 to >200°C, which is greater than that of many so-called 'Mississippi Valley Type' Zn-Pb deposits (Fraser 1996; Carriére and Sangster 1999; Gleeson 2006; Paradis 2007; Wallace 2009). However, there is also a marked lack of age determinations of mineralisation due to the absence of reliable ore-associated geochronometers (Ootes et al., 2013). Constraints include that all are post Late Neoproterozoic, and most are younger than Upper Ordovician, interpreted to have been emplaced between the Cambrian and Early Carboniferous, while some may in part be related to the Cretaceous-Palaeogene Laramide Orogeny (Ootes et al., 2013).
Radiogenic Pb isotope data from galena has been collected from a number of carbonate-hosted Zn-Pb occurrences from the Mackenzie Mountains Zinc District, as well as sediment hosted Zn-Pb from the Selwyn Basin. Comparisons suggest the isotope ratios of stratabound mineralisation at Pairie Creek are very similar to those elsewhere in the Selwyn Basin and may be contemporaneous and therefore Silurian to Devonian in age (e.g., Paradis, 2007). However, Morrow and Cumming (1982), Fraser (1996) and Paradis (2007) have used this data to suggest that the Ag-bearing veins at Prairie Creek are related to Cretaceous deformation, although they are also consistent with Triassic shale hosted mineralisation. In contrast, data from Gayna River (Gleeson et al., 2007) do not match either style of Prairie Creek mineralisation. Isotopic Pb data on galena from a variety of other prospects fall within the range encompassing Gayna River or Prairie Creek data, or between them (Ootes et al., 2013).
Ootes et al., (2011) conclude that regional relationships and Pb isotopic data suggest a minimum of two generations, but more likely three or four of carbonate-hosted Zn-Pb occurrences are present in the Mackenzie Mountains, including: i). stratabound ore possibly related to the Cambrian to Lower Carboniferous sediment-hosted type of the Selwyn Basin; ii). vein and breccia-hosted Zn-Pb, e.g., Gayna River and Bear-Twit, which may or may not also be temporally related to sediment-hosted type of the Selwyn Basin; iii). possible solution collapse carbonate-hosted deposits, and; iv). ~250 to 60 Ma, vein-hosted, Ag-Zn–Pb deposits, as at Prairie Creek, related to Laramide deformation.
Milton (2015) notes that a wide range of straight carbonate-hosted Zn-Pb occurrences in the Mackenzie Mountains yield fluid inclusions with mean
homogenisation temperatures of ~110 to 240°C and mean salinities of 12 to 20 wt.% NaCl equiv.
(Carriere and Sangster, 1999; Gleeson, 2006). These fall within the range of 190 to 260°C NaCl-CaCl2 brines in fluid inclusions from the cupriferous vein-vug-breccia mineralisation in the Redstone Copper Belt as described above. The latter are also geochemically similar to the weakly acidic, reduced 'Selwyn-type' fluids in the sediment hosted Zn-Pb ores, suggesting the former are capable of Zn-Pb transport. Milton (2015) notes there are fluid inclusion characterisitics, mineralogies, deposit-form, textural features, association with carbonate host rocks, discordant structures, breccias and the coarse sparry nature of dolomite-quartz-calcite-sulphides in veins and vugs common to both vein-vug-breccia Cu and Zn-Pb mineralisation in the Mackenzie Mountains. Some of the key examples of carbonate hosted Zn-Pb-Ag mineralisation, also carry appreciable quantities of copper, chiefly as tetrahedrite-chalcopyrite, and appear to be transitional between vein-vug-breccia Cu and the Zn-Pb-Ag occurrences (Milton, 2015). Milton (2015) and Ootes et al., (2011) have therefore suggested that the carbonate hosted Zn-Pb style is transitional with vein-vug-breccia Cu ±Ag ±Zn mineralisation, as the two styles occur in the same geographic area, with the principal difference being metal tenor and ore mineralogy that is related to an evolving metal system. Such an evolution may relate to source rocks in the basin progressively passing through the Cu, then the Zn-Pb maturation windows. Milton (2015) and Ootes et al., (2011) further suggest, major structures, both NE and north to NW trending that were active during deposition of the Neoproterozoic succession (Aitken and Cook 1974; Turner and Long 2008), may have persisted and been reactivated through the Palaeozoic. These structures are indicated to have influenced Cu ingress during the Neoproterozoic, and may have also acted as conduits during the Palaeozoic, as well as influencing structural sites of deposition in the latter period (Turner and Long 2008). If the same structures permitted the introduction of cupriferous fluids during the Palaeozoic, they may well have reinforced grades in the existing Neoproterozoic stratabound Cu deposits. It is likely that the Plateau Thrust and parallel structures in the Mackenzie Arch were extensional listric faults, intermittently active during the long lived rifting in both the Neoproterozoic and Palaeozoic, and were reactivated and inverted during the Laramide compression.
The possible tenuous temporal link between the Cambrian to Lower Carboniferous Selwyn Basin stratabound sediment hosted Zn-Pb, the ~Devonian to Lower Carboniferous (395±63 Ma) Redstone vein-vug-breccia Cu and the possible Palaeozoic Mackenzie Mountains carbonate hosted Zn-Pb mineralisation may be related to the Palaeozoic extension that is taken to be the driver attributed to fluid circulation in the Selwyn Basin.
Much of the information in this summary is drawn from Milton, J.E., 2015 - Sedimentary rock hosted copper mineralization in the Neoproterozoic Redstone Copperbelt, Mackenzie Mountains, Northwest Territories, Canada; A thesis submitted in partial fulfilment of the requirements for the degree of Doctor of Philosophy, University of British Columbia, Vancouver, Canada, 423p. and Gourlay, A.W., 2005 - Technical report on the Coates Lake copper deposit, Nahanni Mining District, western Northwest Territories; An NI 43-101 Technical Report prepared for Lumina Resources Corp., 38p. as well as Milton et al., 2017 cited below.
References
Aitken, J.D. and Cook, D.G., 1974 - Effect of antecedent faults on 'Laramide' structure, Mackenzie Arc: Geological Survey of Canada, Paper 74-1B, pp.
259-264.
Aitken, J. D. and Long, D. G. F., 1978 - Mackenzie tectonic arc-Reflection of early basin configuration?: Geology, v. 6, pp. 626-629.
Brown, A. C., 2005 - Refinements for footwall red bed diagenesis in the sedimenthosted stratiform copper deposits model: Economic Geology, v. 100,
pp. 765-771.
Brown, A. C., 2009 - A process-based approach to estimating the copper derived from red beds in the sediment-hosted stratiform copper deposit model: Economic Geology, v. 104, pp. 857-868.
Carriére, J.J. and Sangster, D.F., 1999 - A multidisciplinary study of carbonatehosted zinc-lead mineralization in the Mackenzie Platform (a.k.a. Blackwater and Lac de Bois platforms), Yukon and Northwest Territories, Canada: Geological Survey of Canada, Open File 3700, 146p.
Cecile, M. P. and Cook, D. G., 1981 - Structural cross section northern Selwyn and Mackenzie Mountains, scale 1:250,000: Geological Survey of Canada, Open File 807.
Chartrand, F.M. and Brown, A.C., 1985 - The diagenetic origin of stratiform copper mineralization, Coates Lake, Redstone Copper Belt, N.W.T., Canada: Economic Geology, v. 80, pp. 325-343.
Chartrand, F. M., Brown, A. C. and Kirkham, R. V., 1989 - Diagenesis, sulphides and metal zoning in the Redstone Copper Deposit, Northwest Territories, in Boyle, R. W., Brown, A. C., Jefferson, C. W., Jowett, E. C. and Kirkham, R. V., (Eds.), Sediment-Hosted Stratiform Copper Deposits: Geological
Association of Canada, Special Paper 36, pp. 189-206.
Coates, J.A., 1964 - The Redstone Bedded Copper Deposit and a discussion on the origin of red bed copper deposits: Unpublished M.Sc. thesis, The University of British Columbia, Vancouver, Canada, 75p.
Colpron, M., Nelson, J.L. and Murphy, D.C., 2007 - Northern Cordilleran terranes and their interactions through time: GSA Today, April/May 2007, pp. 4-10.
Dewing, K., Sharp, R.J., Ootes, L., Turner, E.C. and Gleeson, S., 2006 - Geologic assessment of known Zn-Pb showings, Mackenzie Mountains, Northwest Territories: Geological Survey of Canada, Current Research, 2006-A4, 12p.
Eisbacher, G. H., 1981 - Sedimentary tectonics and glacial record in the Windermere Supergroup, Mackenzie Mountains, northwestern Canada: Geological Survey of Canada, Paper 80-27, 40p.
Fraser, S.C., 1996 - Geology and geochemistry of the Prairie Creek Zn, Pb, Ag deposits, southern Mackenzie Mountains, N.W.T.: Unpublished M.Sc. thesis, University of Alberta, Edmonton, Alberta, 146p.
Furlanetto, F., Thorkelson, D.J., Gibson, H.D., Marshall, D.D., Rainbird, R.H., Davis, W.J., Crowley, J.L. and Vervoort, J.D., 2013 - Late Paleoproterozoic Terrane Accretion in Northwestern Canada and the Case for Circum-Columbian Orogenesis: Precambrian Research, v. 224, pp. 512-528.
Furlanetto, F., 2015 - The evolution of the late Paleoproterozoic Wernecke Supergroup, Wernecke Mountains, Yukon, from sedimentation to deformation: Unpub PhD thesis, Simon Fraser University, Vancouver, B.C., Canada, 250p.
Gabrielse, H., 1967 - Tectonic evolution of the northern Canadian Cordillera: Canadian Journal of Earth Sciences, v. 4, pp. 271-298.
Gabrielse, H., Blusson, S.L. and Roddick, J.A., 1973 - Geology of Flat River, Glacier Lake and Wrigley Lake Map-Areas, District of Mackenzie and Yukon Territory: Geological Survey of Canada, Memoir 366, Ottawa, Canada.
Giger, S.B., Tenthorey, E., Cox, S.F. and Gerald, J.D.F., 2007 - Permeability evolution in quartz fault gouges under hydrothermal conditions: Journal of Geophysical Research: Solid Earth, v. 112, B07202, doi:10.1029/2006JB004828.
Gleeson, S., 2006 - A microthermometric study of fluid inclusions in sulfides and carbonates from Gayna River and the Bear-Twit base metal prospects, Mackenzie Mountains, NWT: Northwest Territories Geoscience Office, NWT Open Report 2006-005, 9p.
Gleeson, S.A. Sharp, R.J., Dewing, K., Krstic, D., Turner, E.L. and Ootes, L., 2007 - A regional S and Pb isotope study of carbonate hosted Zn-Pb mineralization, Mackenzie Mountains, NT, Canada (Abstract): Proceedings of the 9th SGA conference, Dublin, Ireland, pp. 315–319.
Goodfellow, W.D. and Lydon, J.W., 2007 - Sedimentary Exhalative (SEDEX) deposits, in Goodfellow, W.D., (Ed.), Mineral Deposits of Canada: A Synthesis of Major Deposit Types, District Metallogeny, the Evolution of Geological Provinces, and Exploration Methods: Geological Association of Canada, Mineral Deposits Division, Special Publication No. 5, p. 163-184.
Gordey, S.P., Macdonald, J.D., Turner E.C. and Long, D.G.F., 2011 - Structural Geology of the Central Mackenzie Mountains; in Martel, E., Turner, E.C. and Fischer, B.J., (Eds.), Geology of the central Mackenzie Mountains of the northern Canadian Cordillera, Sekwi Mountain (105P), Mount Eduni (106A), and northwestern Wrigley Lake (95M) map areas, Northwest Territories; NWT Geoscience Office, Special Volume 1, pp. 215-250.
Gordey S.P. and Roots, C.F., 2011 - Regional Setting, in Martel, E., Turner, E.C. and Fischer, B.J., (Eds.), Geology of the central Mackenzie Mountains of the northern Canadian Cordillera, Sekwi Mountain (105P), Mount Eduni (106A), and northwestern Wrigley Lake (95M) map-areas, Northwest Territories; NWT Geoscience Office, Special Volume 1, pp. 18-30.
Gourlay, A.W., 2005 - Technical report on the Coates Lake copper deposit, Nahanni Mining District, western Northwest Territories; An NI 43-101 Technical Report prepared for Lumina Resources Corp., 38p
Government of Northwest Territories, 2016 - A Guide to Mineral Deposits, Northwest Territories 2016; NWT Geoscience Office, 187p.
Harlan, S.S., Heaman, L., LeCheminant, A.N. and Premo, W.R., 2003 - Gunbarrel mafic magmatic event: A key 780 Ma time marker for Rodinia plate
reconstructions: Geology, v. 31, pp.1053-1056.
Herut, B., Starinsky, A., Katz, A. and Bein, A., 1990 - The role of seawater freezing in the formation of subsurface brines: Geochimica et Cosmochimica Acta, v. 54, pp. 13-21.
Hewton, R.S., 1982 - Gayna River: a Proterozoic Mississippi Valley-type zinc-lead deposit, in Hutchinson, R.W., Spence, C.D. and Franklin, J.M., (Eds.), Precambrian Sulphide Deposits, H.S. Robinson Memorial Volume: Geological Association of Canada, Special Paper 25, pp. 667–700.
Hildebrand, A.R. , 1978 - Redstone Project, Progress Report March, 1978, Drill Results as of August, 1978 and Recommendations for the 1978 Field Season. Unpublished report to Shell Canada Resources Limited.
Jefferson, C. W., 1983 - The Upper Proterozoic Redstone Copper Belt, Mackenzie Mountains, Northwest Territories: Unpublished Ph.D. thesis, University of Western Ontario, London, Canada, 445p.
Jefferson, C.W. and Parrish, R.R., 1989 - Late Proterozoic stratigraphy, U-Pb zircon ages, and rift tectonics, Mackenzie Mountains, northwestern Canada: Canadian Journal of Earth Sciences, v. 26, pp. 1784-1801,
Jefferson, C.W. and Ruelle, R.C.L., 1986 - The Late Proterozoic Redstone Copper Belt, Mackenzie Mountains, Northwest Territories, in Mineral Deposits of the Northern Cordillera, J.A. Morin (Ed.), Canadian Institute of Mining, Metallurgy and Petroleum Special volume 37, pp. 154-168.
Johnston, S.T., 2001 - The Great Alaskan Terrane Wreck: reconciliation of paleomagnetic and geological data in the northern Cordillera: Earth and Planetary Science Letters, v. 193, pp. 259-272.
Johnston, S.T., 2008 - The Cordilleran Ribbon Continent of North America: Annual Review of Earth and Planetary Sciences, v. 36, pp. 495-530.
Johnston, S.T., 2013 - Advanced Field Geology Western Canada Cordilleran Transect, August 21 to 30, 2013; School of Earth and Ocean Sciences, University of Victoria, Canada, 117p.
Leslie, C.D, 2009 - Detrital zircon geochronology and rift-related magmatism: central Mackenzie Mountains, Northwest Territories: Unpublished M.Sc. thesis, University of British Columbia, Vancouver, BC, 224p.
Lister, G.S., and Snoke, A.W., 1984 - SC mylonites: Journal of Structural Geology, v. 6, pp. 617-638.
Lund, K., 2008 - Geometry of the Neoproterozoic and Paleozoic rift margin of western Laurentia: Implications for mineral deposit settings: Geosphere, v. 4, pp. 429-444.
Lustwerk, R.L., 1990 - Geology and geochemistry of the Redstone Stratiform Copper Deposit, NWT, Canada: Unpublished Ph.D. thesis, Pennsylvania State University, Pennsylvania, U.S.A., 417p.
Lusterk, R.L. and Wasserman, M.D., 1989 - Water escape structures in the Coates Lake Group, Northwest Territories, Canada, and their relationship to mineralization at the Redstone stratiform copper deposit, in Boyle, R.W., Brown, A.C., Jefferson, C.W., Jowett, E.C. and Kirkham, R.V., (Eds.), Sediment-hosted Stratiform Copper Deposits: Geological Association of Canada, Special Paper 36, pp. 207-224.
Macdonald, F.A., Schmitz, M.D., Crowley, J.L., Roots, C.F., Jones, D.S., Maloof, A.C., Strauss, J.V., Cohen, P.A., Johnston, D.T. and Schrag, D.P., 2010 - Calibrating the Cryogenian: Science, v. 327, pp. 1241-1243.
MacNaughton, R.B., Pratt, B.R. and Fallas, K.M., 2013 - Observations on Cambrian stratigraphy in the eastern Mackenzie Mountains, Northwest Territories; Geological Survey of Canada, Current Research 2013-10, 7p. doi:10.4095/292423.
Martel, E., MacNaughton, R. B. and Fischer, B. J., 2011 - Metamorphism and Thermal Maturity: in Martel, E., Turner, E. C. and Fischer, B. J., (Eds.), Geology of the central Mackenzie Mountains of the northern Canadian Cordillera, Sekwi Mountain (105P), Mount Eduni (106A) and northwestern Wrigley Lake (95M) map-areas, Northwest Territories: NWT Geoscience Office, Special Volume 1, pp. 251-254.
Milton, J.E., 2015 - Sedimentary rock hosted copper mineralization in the Neoproterozoic Redstone copper belt, Northwest Territories, Canada: Unpublished Ph.D. thesis, University of British Columbia, Vancouver, 396p.
Milton, J.E., Hickey, K.A., Gleeson, S.A., Falck, H. and Allaz, J., 2017 - In Situ Monazite Dating of Sediment-Hosted Stratiform Copper Mineralization in the Redstone Copper Belt, Northwest Territories, Canada: Cupriferous Fluid Flow Late in the Evolution of a Neoproterozoic Sedimentary Basin : Economic Geology, v.112, pp. 1773-1806.
Moore, T.E. and Box, S.E., 2016 - Age, distribution and style of deformation in Alaska north of 60°N: Implications for assembly of Alaska; Tectonophysics, v. 691, pp. 133-170.
Moynihan, D.P., Strauss, J.V., Colpron, M., Israel, S.A. and Abbott, G., 2014 - Stratigraphic relationships between the Windermere Supergroup and Hyland Group in the Rackla Belt of east-central Yukon: implications for the age of Selwyn Basin and formation of the Laurentian passive margin; Abstracts of the 2014 GSA Annual Meeting in Vancouver, British Columbia (19-22 October 2014), 1p.
Morrow, D.W., 1991 - The Silurian-Devonian sequence in the northern part of the Mackenzie Shelf, Northwest Territories: Geological Survey of Canada, Bulletin 413, 121p.
Morrow, D.W. and Cumming, G.L., 1982 - Interpretation of lead isotope data from zinc-lead mineralization in the northern part of the western Canadian Cordillera: Canadian Journal of Earth Sciences, v. 19, pp. 1070-1078.
Narbonne, G.M. and Aitken, J.D., 1995 - Neoproterozoic of the Mackenzie Mountains, northwestern Canada: Precambrian Research, v.73, pp. 101-121.
Ootes, L., Gleeson, S.A., Turner, E., Rasmussen, K., Gordey, S., Falck, H., Martel., E. and Pierce, K., 2013 - Metallogenic Evolution of the Mackenzie and Eastern Selwyn Mountains of Canadas Northern Cordillera, Northwest Territories: A Compilation and Review: Geoscience Canada, v.40, pp. 40-69, http://dx.doi.org/10.12789/geocanj.2013.40.005.
Paradis, S., 2007 - Isotope geochemistry of the Prairie Creek carbonate-hosted zinc-lead silver deposit, southern Mackenzie Mountains, Northwest Territories, in Wright, D.F., Lemkow, D. and Harris, J., (Eds.), Mineral and Energy Resource Potential of the Proposed Expansion to the Nahanni National Park Reserve, North Cordillera, Northwest Territories: Geological Survey of Canada, Open File 5344, pp. 131-176.
Rainbird, R.H., Jefferson, C.W. and Young, G.M., 1996 - The early Neoproterozoic sedimentary Succession B of northwestern Laurentia: Correlations and paleogeographic significance: Geological Society of America Bulletin, v. 108, pp. 454-470,
Reed, J.C., Wheeler, J.O. and Tucholke, B.E., 2005 - Geological Map of North America: Geological Society of North America, DNAG, scale 1:2 000 000.
Rooney, A. D., Macdonald, F. A., Strauss, J. V., Dudás, F. Ö., Hallmann, C. and Selby, D., 2014 - Re-Os geochronology and coupled Os-Sr isotope constraints on the Sturtian snowball Earth: Proceedings of the National Academy of Sciences, v. 111, pp. 51-56.
Ruelle, R.C.L., 1982 - Depositional Environments and Genesis of Stratiform Copper Deposits of the Redstone Copper Belt, Mackenzie Mountains, N.W.T., in Precambrian Sulphide Deposits, H.S. Robinson Memorial Volume, Hutchison, R.W. Spence, C.D. and Franklin, J.M. (Eds.), Geological Association of Canada Special Paper 25, pp. 701-737.
Smith, H.A., Staples, L.P., Elfen, S., Mosher, G.Z., Wright, F. and Williams, D., 2005 - Prairie Creek Property Feasibility Study; An NI 43-101 Technical Report prepared by AMC Mining Consultants (Canada) Ltd. for Canadian Zinc Corp., 337p.
Strauss, H., 1997 - The isotopic composition of sedimentary sulfur through time: Palaeogeography, Palaeoclimatology, Palaeoecology, v. 132, pp. 97-118.
Tenthorey, E. and Cox, S.F., 2006 - Cohesive strengthening of fault zones during the interseismic period: An experimental study: Journal of Geophysical
Research: Solid Earth, v. 111, B09202, doi: 10.1029/2005JB004122.
Turner, E.C., 2009 - Lithostratigraphy and stable isotope values of the early Neoproterozoic Gypsum Formation (Little Dal Group), Mackenzie Mountains Supergroup, NWT: NWT Geoscience Office Open Report, 2009-002, 26p.
Turner, E.C., Broughton, D.W. and Brooks, T., 2018 - Neoproterozoic carbonate lithofacies and ore distribution at the Kipushi Cu-Zn Deposit, Democratic Republic Of Congo, and Gayna River Zn Camp, Northwest Territories, Canada: Economic Geology, v.113, pp. 779-788.
Turner, E. C. and Long, D. G. F., 2008 - Basin architecture and syndepositional fault activity during deposition of the Neoproterozoic Mackenzie Mountains supergroup, Northwest Territories, Canada: Northwest Territories Geoscience Office Contribution 0040, Canadian Journal of Earth Sciences, v. 45, pp. 1159-1184.
Turner, E.C. and Long, D.G.F., 2012 - Formal definition of the Neoproterozoic Mackenzie Mountains Supergroup (NWT), and formal stratigraphic nomenclature for its carbonate and evaporite formations: Geological Survey of Canada, Open File 7112, 57p.
Turner, E.C., Roots, C.F., MacNaughton, R.B., Long, D.G.F., Fischer, B.J., Gordey, S.P., Martel, E. and Pope, M.C., 2011 - Chapter 3. Stratigraphy; in Martel, E., Turner, E.C. and Fischer, B.J., (Eds.), Geology of the central Mackenzie Mountains of the northern Canadian Cordillera, Sekwi Mountain
(105P), Mount Eduni (106A) and northwestern Wrigley Lake (95M) map-areas, Northwest Territories: NWT Geoscience Office, Special Volume 1, pp. 31-192.
Walker, T.R., Larson, E.E. and Hoblitt, R.P., 1981 - Nature and origin of hematite in the Moenkopi Formation (Triassic), Colorado plateau - a contribution to the origin of magnetism in red beds: Journal of Geophysical Research, v. 86, pp. 317-333.
Walker, T.R., 1989 - Application of diagenetic alterations in red beds to the origin of copper in stratiform copper deposits: in Boyle, R.W., Brown, A.C., Jefferson, C.W., Jowett, E.C. and Kirkham, R.V., (Eds.), Sediment-hosted Stratiform Copper Deposits: Geological Association of Canada, Special Paper 36, pp. 85-96.
Wallace, S.R.B., 2009, The genesis of the Gayna River carbonate-hosted Zn-Pb deposit: Unpublished M.Sc. thesis, University of Alberta, Edmonton, AB, 124p.
Wheeler, J.O. and McFeeley, P., (comp), 1991 - Tectonic Assemblage of the Canadian Cordillera and adjacent parts of the United States of America; Geological Survey of Canada, Map 1712A, scale 1:2 000 000.
Yeo, G.M., 1981 - The late Proterozoic Rapitan glaciation in the northern Cordillera: Proterozoic Basins of Canada: Geological Survey of Canadap, Paper 81-10, p. 25-46.
Young, G. M., Jefferson, C. W., Delaney, G. D. and Yeo, G. M., 1979 - Middle and late Proterozoic evolution of the northern Canadian Cordillera and Shield: Geology, v. 7, pp. 125-128.
The most recent source geological information used to prepare this decription was dated: 2017.
Record last updated: 26/10/2018
This description is a summary from published sources, the chief of which are listed below. © Copyright Porter GeoConsultancy Pty Ltd. Unauthorised copying, reproduction, storage or dissemination prohibited.
Coates Lake
|
We are endeavouring to ascertain why those pages that display Google Maps cannot now do so correctly, and will remedy as soon as practical.
|
|
Chartrand, F.M. and Brown, A.C., 1985 - The diagenetic origin of stratiform Copper mineralization, Coates Lake, Redstone Copper belt, N.W.T., Canada: in Econ. Geol. v80, pp. 325-343.
|
Haynes D W 1986 - Stratiform Copper deposits hosted by low-energy sediments: II. Nature of source rocks and composition of metal-transporting water: in Econ. Geol. v81 pp 266-280
|
Haynes D W 1986 - Stratiform Copper deposits hosted by low-energy sediments: I. Timing of Sulfide precipitation - an hypothesis: in Econ. Geol. v81 pp 250-265
|
Milton, J.E., Hickey, K.A., Gleeson, S.A., Falck, H. and Allaz, J., 2017 - In Situ Monazite Dating of Sediment-Hosted Stratiform Copper Mineralization in the Redstone Copper Belt, Northwest Territories, Canada: Cupriferous Fluid Flow Late in the Evolution of a Neoproterozoic Sedimentary Basin : in Econ. Geol. v.112, pp. 1773-1806.
|
Narbonne, G.M. and Aitken, J.D., 1995 - Neoproterozoic of the Mackenzie Mountains, northwestern Canada: in Precambrian Research v.73, pp. 101-121.
|
Ootes, L., Gleeson, S.A., Turner, E., Rasmussen, K., Gordey, S., Falck, H., Martel., E. and Pierce, K., 2013 - Metallogenic Evolution of the Mackenzie and Eastern Selwyn Mountains of Canadas Northern Cordillera, Northwest Territories: A Compilation and Review: in Geoscience Canada, v.40, pp. 40-69, http://dx.doi.org/10.12789/geocanj.2013.40.005.
|
Turner, E. C., Broughton, D. W. and Brooks, T., 2018 - Neoproterozoic carbonate lithofacies and ore distribution at the Kipushi Cu-Zn Deposit, Democratic Republic Of Congo, and Gayna River Zn Camp, Northwest Territories, Canada: in Econ. Geol. v.113, pp. 779-788.
|
Porter GeoConsultancy Pty Ltd (PorterGeo) provides access to this database at no charge. It is largely based on scientific papers and reports in the public domain, and was current when the sources consulted were published. While PorterGeo endeavour to ensure the information was accurate at the time of compilation and subsequent updating, PorterGeo, its employees and servants: i). do not warrant, or make any representation regarding the use, or results of the use of the information contained herein as to its correctness, accuracy, currency, or otherwise; and ii). expressly disclaim all liability or responsibility to any person using the information or conclusions contained herein.
|
Top | Search Again | PGC Home | Terms & Conditions
|
|