Malartic Mines - East Canadian, Barnat, Sladen, Canadian Malartic |
|
Quebec, Canada |
Main commodities:
Au
|
|
 |
|
 |
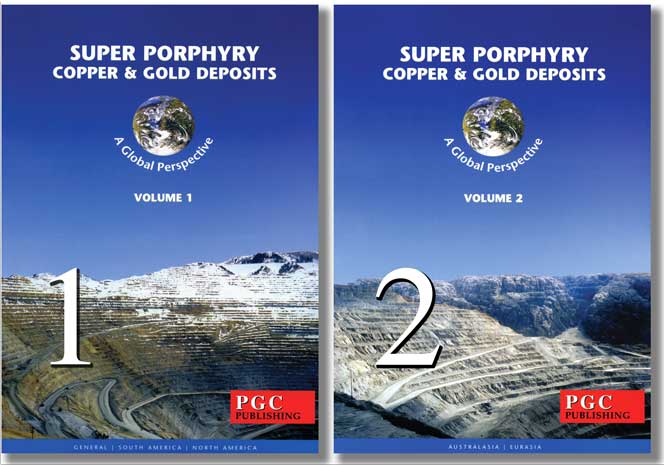 |
Super Porphyry Cu and Au

|
IOCG Deposits - 70 papers
|
All papers now Open Access.
Available as Full Text for direct download or on request. |
|
 |
The Archaean Malartic gold mines are located along the Larder Lake-Cadillac Fault zone, in the Abitibi sub-province of the Superior Province, some 20 km west of Val d'Or in Quebec,Canada.
Historically the main mines of the district - East Canadian, Barnat, Sladen and Canadian Malartic - had produced over 159 tonnes of gold to 2011 from 4 separate underground mines (Helt et al., 2014), namely:
• Canadian Malartic - 9.93 Mt at 3.37 g/t Au;
• Barnat - 4.55 Mt at 6.20 g/t Au;
• Sladen - 3.90 Mt at 2.43 g/t Au;
• East Malartic - 17.95 Mt at 4.92 g/t Au.
The remaining total resource in 2011 was 417 t of Au (Helt et al., 2014), comprising (using a 0.32 g/t Au lower cutoff):
• Measured + indicated resources - 372.9 Mt @ 1.02 g/t Au,
• Inferred resources - 50.4 Mt @ 0.71 g/t Au.
In 2014, the remaining reserves and resources (Osisko web site, 2015) were:
• Proved + probable reserves - 253.9 Mt @ 1.06 g/t Au
• Measured + indicated resources (in addition to reserves) - 45.3 Mt @ 0.76 g/t Au.
Regional Setting
The Malartic gold district is situated in the southeastern Superior Province within the Cadillac-Larder Lake tectonic zone that defines the boundary between the metavolcanic-plutonic and metasedimentary rocks of the Abitibi Greenstone Belt and the metasedimentary rocks of the Pontiac Group/Subprovince, to the north and south respectively (Robert, 1989. The metavolcanic rocks of the Abitibi Greenstone Belt range from ultramafic to felsic in composition and from tholeiitic to calc-alkaline in chemical affinity, and belong to the Malartic, Blake River and Piché groups. The Piché Group is composed of a greywacke-mudstone sequence, and is confined to the Cadillac-Larder Lake tectonic zone).
The earliest igneous activity was the emplacement of volcanic and coeval plutonic rocks between 2747 and 2700 Ma, followed later calc-alkaline only plutons without known extrusive equivalents between 2694 and 2690 Ma (Corfu et al., 1989; Corfu, 1993; Mortensen, 1993; Ayer et al., 2002). During the latter plutonism, flyschoid sedimentary rocks were deposited between 2696 and 2687 Ma (Davis, 1992; Ayer et al., 2002).
Significant D1 contractional deformation, between 2687 and 2680 Ma produced tilting, folding and local thrusting, accompanied by the emplacement of tholeiitic to calc-alkaline affinity diorite to tonalite-trondjhemite plutons at ~2685 Ma (Corfu et al., 1991; Sutcliffe et al., 1993; Robert, 2001).
This was followed by uplift and erosion, leading to the deposition of Timiskaming-type subaerial conglomerates and fluvial sandstones between 2680 and 2672 Ma, uncomformably overlying the volcano-plutonic assemblages (Corfu et al., 1991; Davis, 1992; Corfu, 1993), and broadly contemporaneous with the emplacement of syntectonic plutons and their volcanic equivalents (Mortensen, 1987; Corfu et al., 1989). The latter plutonic rocks comprise an early, syntectonic calc-alkaline, tonalite-granodiorite-granite suite and a late subalkaline to alkaline, monzonite-monzogranodiorite-syenite suite that Sutcliffe et al., (1993) reported to be large ion lithophile element (LILE)-enriched. Small intrusions of diorite, gabbro, clinopyroxenite, hornblendite and lamprophyre accompanied the younger suite (Ayer et al., 2002). These syntectonic plutonic rocks were emplaced during the main D2 period of deformation that produced the regional east-west lithological trend, involving north-south shortening and local sinistral transpression (Robert, 2001).
The D2 event evolved into dextral D3 transcurrent deformation, that was localised along major fault zones, and was partly accompanied and post-dated by granitic plutonism that continued until approximately 2660 Ma (Robert, 2001).
Between 2665 and 2640 Ma, post-tectonic biotite-granite-pegmatite intruded the regionally metamorphosed strata (Feng and Kerrich, 1992; Ayer et al., 2002). All of the supracrustal rocks in the district were affected by regional metamorphism, grading from sub-greenschist to amphibolite facies (Dimroth et al., 1983; Powell et al., 1995), which Powell et al. (1995) bracketed as being between 2677 and 2643 Ma. Retrograde metamorphism was largely restricted to the Cadillac-Larder Lake tectonic zone, which was also the locus of hydrothermal activity and related gold deposition, which occurs within second- and third-order shear zones (Helt et al., 2014).
District Geology
The main Canadian Malartic deposit lies within and immediately to the south of the Malartic tectonic zone, which is part of the Cadillac-Larder Lake tectonic zone, and is the dominant local feature. This tectonic zone is defined by major east-west trending faults, specifically the Malartic and Sladen faults to the north and south, respectively, as well as several NE-SW and NW-SE trending subvertical structures that together create lozenge-shaped fault blocks (Fallara et al., 2000). This tectonic zone, which is ~650 m wide, is restricted to the mafic-ultramafic rocks of the Piché Group, which has been converted to typically bluish-grey and pervasively foliated, strongly deformed, biotite and carbonate altered, magnesian basaltic to komatiitic volcanics, with numerous veinlets of talc-carbonate-chlorite (Sansfaçon and Hubert, 1990). These rocks pass under the Pontiac Group rocks in the vicinity of the South Barnat orebody, and are not exposed south of the Cadillac-Larder Lake tectonic zone (Helt et al., 2014).
Whilst gold mineralisation is found within the Piché Group, the majority of the Canadian Malartic deposit occurs to the south of the tectonic zone, hosted by sedimentary rocks of the Pontiac Group and felsic to intermediate porphyritic intrusives that have undergone potassic, carbonate and silicic alteration. The sedimentary succession of the Pontiac Group is comprises greywackes, shales and minor siltstones, i.e., turbiditic clastic sedimentary rocks that are generally rhythmically layered with beds ranging from ~1 mm to 1 m in thickness. These rocks typically have a well-developed foliation and are dark grey to black, locally with a brownish tint due to the development of metamorphic and/or hydrothermal biotite proximal to porphyritic intrusions. Both the Piché and Pontiac Group rocks are intruded by porphyries, which vary from light grey to reddish in colour with progressive alteration, and are feldspar-phyric with fine-grained to aphanitic matrices. These porphyries are monzodioritic, ranging from subalkaline to alkaline dacite to andesite and trachyandesite. The porphyritic monzodiorite has been dated at 2677 to 2679 Ma (zircon U-Pb ages; Clark et al., 2014), while the intruded Pontiac Group has a minimum age of 2682 ±3 Ma (Davis, 2002). These latter ages are comparable to the 2680 to 2676 Ma reported previously for late tectonic, dominantly alkalic, felsic to intermediate intrusions elsewhere in the southern Abitibi (Frarery and Krogh, 1986; Corfu et al., 1989; Jemielita et al., 1990; Davis et al., 2000; Helt et al., 2014).
Structure and Metamorphism
Three deformational events have been identified that affected rocks in the Malartic region (Sansfaçon and Hubert, 1990), namely:
• D1, between ~2687 and 2680 Ma, manifested by a series of weakly to moderately dipping anticlines and synclines, F1, with NE-SW axial planes and a weak, penetrative S1 schistosity. F1 folds are rare, but occur locally in the hinges of F2 folds, where S1 is at a high angle to S2.
• D2, between ~2680 and 2675 Ma, characterised by a series of subvertical, sub-isoclinal F2 folds, with NE-oriented axial planes that refold D1 structures, to generate asymmetric S-shaped folds that plunge at 40 to 80°east. The NW-SE oriented S2 schistosity was much more penetrative, as reflected by oriented biotite, than the weaker D1 schistosity, which it crenulated. D2 deformed the calc-alkaline intrusions in the Malartic region, which are therefore probably pre-D2 and likely pre- to syn-D1 in age. The intrusions hosting Malartic gold mineralisation are syn-D2 at 2677 to 2679 Ma.
• D3 between ~2675 and 2660 Ma, produced N-NW trending, vertically dipping S3 cleavages and fractures that are most conspicuous along the contact between the Piché and Cadillac Groups. This deformation produced the Malartic synform a regional structure, the fold axis of which trends WNW and plunges steeply to the north (Helt et al., 2014).
The grade of metamorphic increases towards the southerly, grading from sub-greenschist facies in rocks of the Cadillac Group immediately north of the Malartic tectonic zone, to upper greenschist facies in the Piché Group rocks within the tectonic zone, to upper greenschist and amphibolite facies in the Pontiac Group further to the south (Dimroth et al., 1983; Powell et al., 1995). The garnet-isograd is located on the southern margin of the Canadian Malartic deposit, whilst the staurolite-isograd is ~1.7 km further south (Sansfaçon, 1986). Rocks of the Piché Group have subsequently undergone retrograde metamorphism, occurring as chloritisation of biotite, formation of actinolite after hornblende, and albitisation of calcic plagioclase. This is regarded to be a consequence of the extended, high flux of hydrothermal fluids through the Malartic tectonic zone (Helt et al., 2014).
Mineralisation and alteration
The main gold bearing ore shoots at Barnat and East Malartic were located within the Malartic tectonic zone, whereas those within the Canadian Malartic and Sladen deposits were concentrated south of the zone, within the Pontiac Group and subjacent to the Sladen fault (Sansfaçon and Hubert, 1990).
The current (2015) Osisko open-pit mine exploits ore remaining from the Canadian Malartic, Sladen and Barnat underground operations as well as more recently defined near-surface, low-grade mineralisation south of the Malartic tectonic zone within and spatially associated with small porphyritic bodies intruding Pontiac Group metasedimentary rocks, all of which are part of a single, large, low-grade mineralised system. This system comprises two contiguous zones, the 3.2 km long, east-west trending Canadian Malartic orebody and its eastern extension, the South Barnat orebody. The latter is truncated by the southern structure of the Malaritc tectonic zone. The maximum width of the mineralised system is 900 m, whilst the vertical open-pit mineable depth is 420 m. The system remains open down-plunge to the east. The Canadian Malartic orebody is hosted by Pontiac Group metasedimentary rocks and monzodiorite porphyry intrusions, whilst the South Barnat orebody, is also hosted by Piché Group rocks (Helt et al., 2014).
Gold mineralisation is associated with a virtually continuous shell of 1 to 5% disseminated pyrite with widespread potassic (K feldspar and biotite) and carbonate alteration, accompanied by minor sericitisation, which is locally overprinted by silicification and late-stage chloritisation. This alteration produces strongly altered rocks in both the monzodiorite porphyry and greywacke, characterised by broadly similar mineral assemblages, dominated by albite, quartz, carbonate (mainly calcite with lesser ankerite), K feldspar and biotite. Quartz and biotite are more abundant in greywacke. They also include minor pyrite, sericite and chlorite, plus traces of hematite (observed in the porphyries only, where it is after magnetite), barite (only in porphyries), rutile, titanite and scheelite. In addition to pyrite, the sulphides include minor to trace chalcopyrite, galena, molybdenite, pyrrhotite and sphalerite. This assemblage is accompanied by traces of native gold, hessite (Ag2Te), petzite (Ag3AuTe2), altaite (PbTe), and calaverite (AuTe2), as well as bismuth and nickel tellurides. The main alteration minerals in the Piché Group rocks are biotite, plagioclase, actinolite and carbonate minerals (calcite with lesser ankerite), which are accompanied by subordinate pyrite, magnetite and talc. The sulphide and telluride minerals in the porphyry and greywacke are similar (Helt et al., 2014).
The paragenesis of alteration and mineralisation commences with an early, pre-ore stage alteration, characterised by quartz with minor albite and carbonate (mainly calcite), replacing the monzodiorite porphyry intrusion and greywacke matrix, but also occurs within V1 veinlets that cut all lithotypes. The carbonate alteration is commonly accompanied by sericite, which is intergrown with the carbonate minerals, in the cores of feldspars, and throughout the porphyry groundmass and greywacke matrix (Helt et al., 2014).
The pre-ore assemblage was overprinted by extensive pervasive and vein-related V2 main potassic alteration, which is intimately associated with the gold mineralisation. In the monzodiorite porphyry, this pervasive potassic alteration takes the form of a replacement of plagioclase by K-feldspar (commonly accompanied by finely disseminated hematite) and biotite. Within the greywacke, pervasive potassic alteration is characterised by an increase in biotite abundance relative to the unaltered greywacke, where biotite is also an important metamorphic phase, and in Piché Group rocks by the replacement of mafic minerals by biotite (Helt et al., 2014).
Metamorphic biotite in poorly altered greywacke is characterised by Fe/[Fe + Mg] = 0.44, and hydrothermal biotite by Fe/[Fe + Mg] = 0.37 to 0.31, whilst the Al content decreases with increasing alteration. Similarly, the biotite in the unaltered metamorphic precursor is low in fluorine (avg 0.2 wt.%), whereas in strongly altered rocks, hydrothermal biotite contains, on average 0.5 to 0.6 wt.% fluorine, which increases with increasing alteration and mineralisation toward the porphyries, in which altered igneous biotite is also fluorine-rich (Helt et al., 2014).
Vein-related potassic alteration produced biotite-K feldspar-calcite±pyrite haloes around quartz, quartz-carbonate±biotite or quartz-carbonate-albite V2 main veinlets. Biotite formed during earlier stages of alteration and veining is replaced by minor chlorite, which is most conspicuous in haloes around V2 main veinlets. Pyrite was deposited in conjunction with potassic alteration, and is ubiquitous within the deposit, occurring both as disseminated grains and in mineralised veinlets. The main-stage veins form local stockwork breccias where the vein density is relatively high (Helt et al., 2014).
Massive silicification and quartz veining in the late ore-stage locally overprints potassic alteration, with late ore-stage V2 late veins averaging 1.5 cm in width, containing coarse-grained quartz, and commonly, large muscovite and biotite crystals in addition to subordinate pyrite and calcite. Although late ore-stage veins are hosted strong potassic altered rocks, they lack the haloes characteristic of main ore-stage veinlets. Locally significant but volumetrically minor hydrothermal breccias, with angular to subangular fragments consisting almost entirely of quartz, overprint V2 main veins and potassic alteration (Beaulieu, 2009). Carbonate alteration was extensive and long lived, outlasting the ore-stage, and occurs as patchy aggregates of calcite, as well as post-ore calcite-only veinlets, having sharp contacts with the host rocks that overprint and crosscut early quartz±albite±carbonate, potassic and later silica alteration occurring as V3 veins (Helt et al., 2014).
Gold mineralisation - The bulk of historically mined gold mineralisation occurred as microscopic grains, generally concentrated in large veins of quartz±carbonate, and within pyrite, biotite, carbonate, sericite, chlorite and hematite haloes around these veins (Sansfaçon and Hubert, 1990). Visible gold grains were occasional associated with tellurides in quartz veins (Helt et al., 2014).
In the larger, bulk mining resource, apart from this vein association, gold occurs predominantly as fine-grained native gold with an average gold:silver weight ratio of 9:1, locally accompanied by subordinate gold telluride minerals, chiefly petzite and locally calaverite. The gold-bearing phases occur with pyrite and minor (<0.1%) fine-grained chalcopyrite, galena, sphalerite, hematite, hessite and other telluride minerals. Native gold, tellurides, sulphides, and hematite occur both as isolated grains and as complex intergrowths in ore-stage V2 main and V2 late veinlets and veins, and as grains disseminated within the host rocks.
Gold-bearing minerals generally display a strong spatial association with pyrite, K feldspar and biotite, as is evident in both vein haloes and in the pervasively altered wall rocks. This is illustrated by the distribution of native gold grains in the Canadian Malartic orebody, where most gold mineralisation is directly associated with pyrite. Studies reported by (Helt et al., 2014) show that of a suite of 291 native gold grains from that orebody that ranged from 1 to 30, averaging 4 µm, 35% were associated with pyrite with 18% being inclusions within pyrite, whilst 7% occurred along pyrite-pyrite boundaries, and a further 10% were on the contacts of pyrite with other minerals (mainly K feldspar, biotite, plagioclase and quartz). In addition, most of the gold grains associated with pyrite are larger than average, such that by volume, inclusions in pyrite constitute ~49% of the native gold, and grains along contacts of pyrite with pyrite, K feldspar, and biotite constitute ~26%. Volumetrically, most of the remaining native gold occurs along contacts involving K feldspar and biotite. An analogous study at the higher grade South Barnat orebody (349 grains) yielded similar results, with most of the native gold being associated with pyrite and lesser amounts with K feldspar and biotite. Appreciable amounts of native gold also occur with amphibole in altered mafic-ultramafic rocks. In both orebodies, gold-bearing tellurides are of minor abundance compared to native gold, but have similar distributions (mainly as inclusions within or at contacts with pyrite; Helt et al., 2014)
For more detail consult the reference(s) listed below.
The most recent source geological information used to prepare this decription was dated: 2014.
This description is a summary from published sources, the chief of which are listed below. © Copyright Porter GeoConsultancy Pty Ltd. Unauthorised copying, reproduction, storage or dissemination prohibited.
|
|
Helt K M, Williams-Jones A E, Clark J R, Wing B A and Wares R P, 2014 - Constraints on the Genesis of the Archean Oxidized, Intrusion-Related Canadian Malartic Gold Deposit, Quebec, Canada : in Econ. Geol. v.109 pp. 713-735
|
Herzog, M., LaFlamme, C., Beaudoin, G., Marsh, J. and Guilmette, C., 2023 - U-Pb vein xenotime geochronology constraints on timing and longevity of orogenic gold mineralization in the Malartic-Val-d Or Camp, Abitibi Subprovince, Canada: in Mineralium Deposita v.58, pp. 105-133.
|
Perrouty, S., Linnen, R., Lesher, C.M., Olivo, G.R., Piercey, S., Gaillard, N., Clark, J.R. and Enkin, J., 2019 - Expanding the size of multi-parameter metasomatic footprints in gold exploration: utilization of mafic dykes in the Canadian Malartic district, Quebec, Canada: in Mineralium Deposita v.54, pp. 761-786.
|
Porter GeoConsultancy Pty Ltd (PorterGeo) provides access to this database at no charge. It is largely based on scientific papers and reports in the public domain, and was current when the sources consulted were published. While PorterGeo endeavour to ensure the information was accurate at the time of compilation and subsequent updating, PorterGeo, its employees and servants: i). do not warrant, or make any representation regarding the use, or results of the use of the information contained herein as to its correctness, accuracy, currency, or otherwise; and ii). expressly disclaim all liability or responsibility to any person using the information or conclusions contained herein.
|
Top | Search Again | PGC Home | Terms & Conditions
|
|