Main Tintic |
|
Utah, USA |
Main commodities:
Au Ag Cu Pb Zn
|
|
 |
|
 |
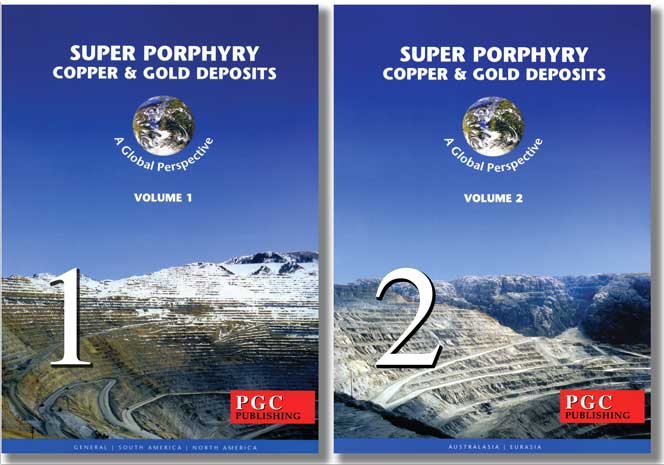 |
Super Porphyry Cu and Au

|
IOCG Deposits - 70 papers
|
All available as eBOOKS
Remaining HARD COPIES on
sale. No hard copy book more than AUD $44.00 (incl. GST) |
|
 |
The Main Tintic sub-district lies within the Tintic district of Utah which is located near the town of Eureka, approximately 90 km to the SSW of Salt Lake City in north-central Utah. For background information and geological setting see the Tintic District record.
The metalliferous ore deposits of the Main Tintic district include both fissure and "replacement veins" as well as the extensive "replacement" bodies. Together these styles of mineralisation form persistent linear zones, or "ore runs" that extend in a NNE direction across the Silver City stock and adjacent rocks, and northerly through the Palaeozoic sediments. In the carbonates to the north of the stock five main "ore runs" of "replacement" ore have been recognised and exploited, as shown on the accompanying plan. The linear character of the three principal ore zones persists through the Silver City stock, and beyond into the altered lavas and tuffs. The Main Tintic district ore zones are aligned with those of the East Tintic sub-district, separated by an un-explored interval covered by volcanics and intrusives (Morris, 1968).
The variation in the form and character of the Tintic orebodies is a result of the dissimilarities in the physical and chemical characteristics of the host rocks, and the distance from the Silver City stock. In the low reactivity siliceous, argillaceous and some contact metasomatic rocks, the orebodies are restricted to the confines of the host fissures, occurring as tabular veins. As these veins extend into zones of weaker contact metasomatism, they degenerate into a series of pods or steeply dipping lenses (Morris, 1968).
In contrast, in the more reactive and easily brecciated dolomites and limestones beyond the contact zone, the orebodies are characteristically diverse in form and unpredictable in habit. In this zone they tend to form irregular, pipe-like, columnar or bulbous forms which only conform to the structures with which they are associated in a general way. The gradation from fissure veins, to "replacement veins", to "replacement" orebodies is almost imperceptible, although each form is distinct (Morris, 1968).
The "replacement" orebodies within folded and faulted Palaeozoic carbonates account for more than 90% of the ore from the Tintic district. These bodies range from insignificant stringers and small kernels, to large columnar, linear and bulbous masses, some containing several hundred thousand tonnes of ore. Several of the columnar and linear orebodies of the district resemble the chimney and manto deposits described from mines in Mexico and Colorado previously, although, in general the structural complexity and varied lithology of the host rocks precludes such an elementary comparison (Morris, 1968).
The most productive deposits in the field have been the large columnar masses (or chimneys), locally termed "pipes", particularly those of the Mammoth, Chief, Gemini, Centennial Eureka and other mines (see the accompanying plan). The largest was the Apex orebody of the Mammoth mine which followed the hangingwall of the Sioux-Ajax fault, plunging steeply from the surface to the 730 m level (2400 ft) of the mine, where it merges with the Gold Chain fissure. It is roughly elliptical in plan, averaging 60 m in breadth, by 10 to 30 m in width. The comparable Granite pipe, near the Centennial fault in the Chief No. 1 mine has a vertical extent of near 250 m, and a diameter which varies from 40 to 275 m. Others of the larger pipes have vertical extents of between 330 and 400 m (Morris, 1968).
The largest manto-like orebody in the Tintic district follows a near horizontal section of the axis of the Tintic syncline northward for nearly 1.6 km. In the Colorado mine this manto has a width of 6 to 18 m and averages 6 m in height, although elsewhere along its course it has cross-sections with double these values. In places a manto (or horizontal pipe) may follow a particular bed along the crest of an antiform or synform for a distance of nearly 2 km, with a width of 6 to 50 m, and a thickness of 6 to 18 m, as in sections of the Iron Blossom ore zone. The deepest manto-like body within the field replaces fractured dolomite beneath a gently dipping bedding plane fault. It consists of three separate, sinuous orebodies that locally branch and coalesce, surrounding un-replaced masses of host rock. The individual bodies are 5 to 12 m wide and 1 to 7.5 m thick and have been followed down dip for 250 m where they were abandoned due to high mining and pumping costs (Morris, 1968).
The replacement orebodies that are not specifically either chimneys or mantos, are mostly pod like, although they are unpredictable and irregular in habit, differing widely in size and character. They may have manto-like offshoots that follow flat-lying beds, and vein-like, pipe-like and sinuous extensions that locally follow steep faults, fissures and steep bedding planes. One of the largest of these bodies is the 18-316 of the Chief No. 1 mine. This pod is found near the intersection of several major faults, where extensive breccia zones have been developed in the Victoria, Pinyon Peak and Fitchville Formations. In plan view it has a crab-like shape with a central bulbous body and several leg-like appendages extending to the south, north and north-east along faults and favourable beds. The central body is 100 m long and 50 m wide and extends for around 75 m vertically. The contacts with the brecciated country rocks are very ragged, with many un-replaced residual blocks of altered dolomite near the edges of the ore mass (Morris, 1968).
Essentially all of the "replacement" ore bodies of the district are siliceous, consisting of jasperoid, barite and metallic minerals. In the northern section of the mineralised area the jasperoid is flinty or cherty, and commonly only includes a few plates of barite, with varying amounts of sphalerite, galena, pyrite, enargite, argentite and less abundant silver sulpho-salts. In the central sections of the ore field the jasperoid is coarser and the plates of barite are larger and more fully developed. In both the north and central areas the jasperoid is strongly banded. Some bands have developed as a result of the desiccation of hydrated colloidal silica, while others are interpreted to have formed by rhythmic deposition of sulphides and other minerals from the original colloidal mass. In the southernmost "replacement" bodies, nearest the Silver City stock, the orebodies are more coarse grained, locally resembling quartzite or fine grit, which in places have barite dominating over silica. This silica occurs as well formed crystals, coating as many as three generations of platy barite crystals (Morris, 1968).
In all of the replacement orebodies there is evidence of two or more phases of silicification and brecciation. Barite is more abundant in jasperoid of the second phase. The ore minerals were emplaced in volume with the quartz and barite of the second phase, with galena in particular being deposited as large crystals or grains. The transition between the ore and country rock is typically sharp and distinct, locally marked by a thin selvage of clay separating the jasperoid casings of the ore shoots from the un-silicified, but commonly pyritic and 'sanded' carbonate hosts. A characteristic feature of these ores above the water table, is a halo like zone of "chocolate rock" in the adjacent limestones and dolomite. This results from the oxidation of an outward zone of diminishing weakly "sanded", iron stained rock produced by the oxidation of disseminated pyrite (Morris, 1968).
Replacement veins, south of the east-west Sioux-Ajax fault and adjacent to the northern margin of the Silver City Stock, occur in pyrometasomatically altered carbonate rocks. Essentially these are narrow relative to their length and height, but unlike the fissure veins in the adjacent intrusive, they more completely replace the brecciated fillings of the fissure zones and locally expand on cross-fractures and bedding breccias. Most are only a hundred metres or more in length, although one has been mined continuously for a distance of more than 750 m. Within these veins, ore occurred as shoots that locally expand from a few cms to 15 to 20 m in diameter, to tabular masses that are 100 m or more in length and height, and are 1 to 2 m thick. With few exceptions all are found within 250 m of the current surface. The texture of the veins is very similar to that of the southern sections of the "replacement orebodies" described above (Morris, 1968).
Fissure veins with well defined contacts and crustification textures are common in the Tintic Quartzite, Ophonga Limestone, volcanic rocks and the majority of the intervals where the ore zones cut the Silver City stock. These veins may be up to 6 m thick, but average 0.6 m. Most are less than a hundred metres or so in length, although one is continuous for around 1200 m. They generally occupy small normal faults which trend at around 20° and dip at 75 to 85°W, although some are steep to east dipping. Such veins are longest and widest where they cut massive intrusive and sedimentary rocks, but tend to form groups of short, sub-parallel veins, or disappear in altered tuffs and in incompetent argillaceous sediments. The banded veins of the Silver City stock consist of vertical layers of quartz, pyrite, galena and other minerals that lie parallel to the fissure walls. Some veins contain central vugs, while other enclose bands of country rock, which may in some cases be replaced by silica. The most extensively mined vein was exploited over a vertical interval of 230 m and a horizontal distance of 270 m, with an average thickness of 1 m, but ranging from a narrow streak to 3 m. Oxidation was complete to almost the 75 m level, forming cerussite, anglesite, plumbojarosite, jarosite, argentojarosite and cerargyrite in a matrix of iron-stained, honey-combed quartz (Morris, 1968).
The more productive of the Tintic orebodies are preferentially developed within certain stratigraphic units and structural features, despite the through going nature of the ore runs. Most of the "replacement" ores have come from a limited number of host formations, principally the Cole Canyon and Ajax Dolomites, the Fish Haven, Bluebell and Victoria Formations, the Pinyon Peak, Fitchville and Gardison Formations and the upper part of the Deseret Limestone. Orebodies in the Ajax, Bluebell, Fitchville and Deseret Formations are estimated to have yielded more than 75% of all of the ore produced from the district. Replacement veins within the calc-silicate [mainly pyritic jasperoid] aureole of the Silver City stock do not apparently show a preference for a particular protolith (Morris, 1968).
Structural controls are equally as obvious for the fissure and replacement vein deposits and some of the "replacement" orebodies. The breccia zones are of particular importance in the development of the replacement deposits. Individual ore runs may be traced more or less continuously over distances of up to 3.5 km. In this interval the mineralised zone may proceed from a series of veins within the Silver City stock in the south, to replacement veins in the calc-silicate altered aureole of the stock, to replacement orebodies within the Palaeozoic carbonates. The "replacement" orebodies take the form of chimneys, mantos, pod-like or irregular bodies which may follow particular carbonate beds, both up dip and along strike, or along the intersection of a fault and a favourable carbonate bed. In places along the path of the ore run, mineralisation may cut across the sequence along a fault/shear/fracture, forming vein ore in silicates and replacement bodies or veins in carbonates. When a replacement chimney or manto encounters a fault that truncates the unit being followed, and juxtaposes another unit, mineralisation may form an irregular body in the hangingwall of the fault and spread either up or down, or both to form an irregular body parallel to the fault. The path of the ore runs is not exclusively upwards (Morris, 1968).
The ores have been segregated into a number of classes, as follows:
1). Lead ores, containing from 5 to 50% Pb, as much as 1500 g/t Ag, rarely small quantities of Zn and Cu, and traces of Au;
2). Siliceous lead ores, with a few percent Pb and minor Zn and Cu in a siliceous gangue of >70% SiO2. Ag is locally high;
3). Siliceous silver ores, which carry 600 g/t Ag or less, and traces of Au, but locally lack any identifiable metallic minerals;
4). Lead-zinc ores, with 5 to 15% of each Pb and Zn and 250 to 300 g/t Ag;
5). Copper-gold ores, containing a few percent or more of Cu, 300 to 600 g/t Ag and commonly 15 g/t Au or more; and
6). Siliceous lead-copper ores, generally containing only a few percent of each Pb and Cu, and occurring where Cu gives way to Pb ores (Morris, 1968).
The principal primary minerals for the individual elements are as follows: Au - sylvanite and native gold [occurring with enargite]; Ag - argentite [which is ubiquitous in association with the galena ore], but with lesser proustite, pearceite, freibergite, native silver, pyrargyrite and stephanite; Pb - galena; Cu - enargite [commonly intergrown with famatinite], tennantite and tetrahedrite, both of which are commonly argentiferous, but less common, while chalcopyrite is also observable in some mines; Zn - sphalerite/marmatite [Cd is consistently present in the Zn ores, probably within the sphalerite lattice]; Fe - pyrite, although marcasite and arsenopyrite are also present, but are not abundant. The paragenesis commences with jasperoid, and barite of several generations, followed by pyrite, some of which is intimately associated with late quartz. This is followed by enargite and famatinite which are almost simultaneous. Galena follows and then sphalerite. Locally rich shoots of Ag in the form of native silver, proustite, argentian tennantite and pale, reddish sphalerite are later than the galena, ferroan sphalerite and tetrahedrite ores. In some mines calcite, dolomite and ankerite fill late vugs and cement fractures (Morris, 1968).
In all of the Tintic mines oxidation has penetrated to the water table, but nowhere is it complete. Residual masses of enargite, pyrite and galena may be found at all levels, embedded in the generally soft, friable masses of cellular and cavernous, gossan like oxidised ore. The oxidised copper ores commonly contain malachite, azurite, impure chrysocolla, copper arsenates and rarely turquoise, cuprite and native copper. Covellite and chalcocite are widespread although at low levels. The matrix is typically fractured and corroded iron stained jasperoid, most of which contains barite. The argentiferous lead ores are oxidised to produce anglesite, cerussite, plumbojarosite, pyromorphite and mimetite. Silver in the oxidised zone commonly takes the form of native silver, cerargyrite and argentojarosite. The nearly complete oxidation of mixed sphalerite and galena ores has in some mines led to the downward migration of zinc forming residual cerussite and anglesite and separate secondary zinc mineralisation at the contact with carbonate wall rock. The nearly lead free secondary zinc ore was deposited as ferruginous smithsonite, hemimorphite [calamine], hydrozincite, aurichalcite and other minerals, accompanied by a gangue of un-replaced limestone, dolomite, chert, jarosite and the hydrous oxides of iron and manganese and by calcite and gypsum. Most of this ore contained 20 to 30% Zn (Morris, 1968).
Supergene sulphide enrichment is evident in some mines, where secondary zinc sulphides, such as wurtzite [ZnS], was developed below the water table, while only zinc free ore was found above. Evidence of Zn enrichment persists in these mines for up to 50 m below the water table. Examples of supergene enrichment occur in ores that are found in extensive masses of carbonate free jasperoid containing abundant primary pyrite (Morris, 1968).
Alteration is evident within the wallrocks of mineralised quartz veins cutting the Silver City Stock and other igneous bodies in the Tintic district. This alteration takes the form of selvages commencing with quartz-sericite-pyrite on the margin of the vein which retains the porphyry texture of the host. This grades outwards into a wider zone of nearly argillised rock containing clay minerals, chiefly kaolinite and endellite. In places where it is widely developed, the argillised zone contains dickite on the vein-ward side and merges with the propylitic zone containing decreasing amounts of kaolinite and increasing quantities of montmorillonite, epidote, chlorite group minerals and carbonates (Morris, 1968).
The probable counterpart of this quartz-sericite-pyrite alteration in the adjacent carbonates is the sericitic-jasperoid-pyrite that is commonly fractured and recemented by sulphides, baritic jasperoid and fine grained quartz of the ore stage. The argillised zones are represented by the irregular masses of "sanded" limestone and dolomite or by solution breccias, except near shale beds or layers of argillaceous limestone which locally provided sufficient alumina and silica to produce clay minerals. In the sedimentary rocks the quartz-sericite-pyrite mineralisation was preceded by pervasive dolomitisation (Morris, 1968).
A general horizontal zonation of metals, and to a lesser degree texture, is evident in the ore-runs at Tintic. The replacement veins and "replacement" orebodies in the south, nearest the margin of the Silver City stock, carry predominantly Cu and Au, while the mines at intermediate distance produced mainly Pb and Ag. The northern most orebodies carry as much Zn as Pb and contain significantly less Ag. It should be noted however that much Pb and some Zn ore has also been exploited in the south, while no Cu and only insignificant Au is found in the northern or outer Zn rich sections of the ore-runs. The texture of quartz varies from well developed crystals in veins within and adjacent to the stock, to granular aggregates of small quartz crystals in the Cu zones adjacent to the stock, to a finer grained, chert like jasperoid in the Ag-Pb zone, to fine grained jasperoid in the Zn rich zone. Vertical zonation of the individual orebodies is not evident (Morris, 1968).
For detail also consult the reference(s) listed below.
The most recent source geological information used to prepare this decription was dated: 1996.
This description is a summary from published sources, the chief of which are listed below. © Copyright Porter GeoConsultancy Pty Ltd. Unauthorised copying, reproduction, storage or dissemination prohibited.
|
Porter GeoConsultancy Pty Ltd (PorterGeo) provides access to this database at no charge. It is largely based on scientific papers and reports in the public domain, and was current when the sources consulted were published. While PorterGeo endeavour to ensure the information was accurate at the time of compilation and subsequent updating, PorterGeo, its employees and servants: i). do not warrant, or make any representation regarding the use, or results of the use of the information contained herein as to its correctness, accuracy, currency, or otherwise; and ii). expressly disclaim all liability or responsibility to any person using the information or conclusions contained herein.
|
Top | Search Again | PGC Home | Terms & Conditions
|
|