Greens Creek |
|
Alaska, USA |
Main commodities:
Au Zn Ag Pb
|
|
 |
|
 |
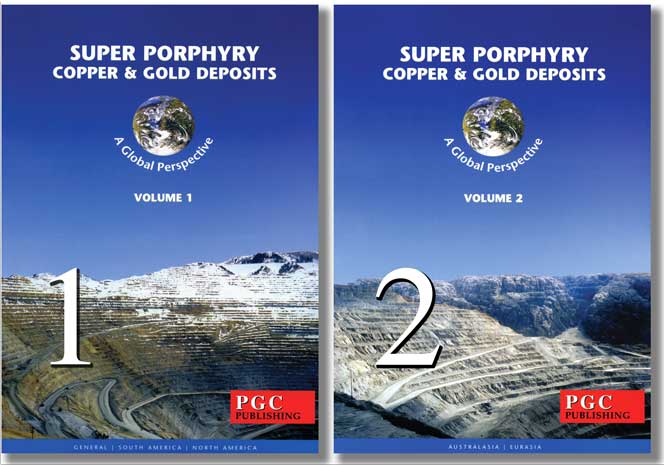 |
Super Porphyry Cu and Au

|
IOCG Deposits - 70 papers
|
All papers now Open Access.
Available as Full Text for direct download or on request. |
|
 |
The Greens Creek polymetallic gold-silver-zinc-lead (Sb-As-Hg-Mo-Tl-Cu) massive sulphide deposit is located on northern Admiralty Island, ~25 km SSW of Juneau in the southern 'pan-handle' of Alaska, USA,
(#Location: 58° 4' 58"N, 134° 38' 14"W).
The Pan Sound Joint Venture, a consortium that included Noranda Exploration (29.73%), Marietta Resources (29.73%), Exhalas Resources (29.73%) and Texas Gas Exploration (10.81%), was formed in 1973 to undertake regional exploration in south-eastern Alaska. Mineralisation was discovered in 1974 at the 'Big Sore', a gossanous/ferricrete vegetation kill-zone over sub-cropping copper sulphide mineralisation. This subcrop was discovered during follow-up of Zn and Cu anomalies from a regional geochemical stream sediment sampling program. The anomalous sample returned a value of 1300 ppm Zn, with float of basalt at the sample site containing disseminated pyrite and chalcopyrite. Soil sampling grids, geophysical surveys and drilling expanded the footprint of mineralisation, with numerous soil anomalies of ~100 ppm Ag and up to 1.7% Zn. The first diamond drill hole, which was commenced in 1975, sited some 50 m above the Big Sore, intersected 27 m @ 0.43% Cu, 8.03% Zn, 2.04% Pb, 4.2 g/t Au, 198 g/t Ag. The hole was terminated at 90 m with caving problems, still in dolomitic graphite-quartz-mica schist, with local bands of massive sulphide. Exploration continued with Noranda becoming the operator in 1976 when the first mineral resource was calculated. Various of the partners sold their shares in the JV which was dissolved in 1978 when the remaining and incoming partners, and the Bristol Bay Native Corporation, formed the Greens Creek Joint Venture. A feasibility study was commenced in 1983 and mine and mill construction began in late 1986. Hecla Mining acquired an interest in 1987. Production commenced in early 1989, with Rio Tinto Zinc (RTZ) having entered the venture and becoming manager. Further orebodies were discovered in 1990 and 1993, smaller partners were bought out, and by 1994 the joint venture was RTZ (through Kennecott) 70.27%; Hecla Mining 29.73%. The mine was placed on care and maintenance in early 1993 due to low metal prices, and exploration accelerated, resulting in further extensions of the known mineralisation. The mine re-opened in early 1996. Hecla obtained a 100% interest in the Project in 2008 and has continued production to the present (2021).
Greens Creek mineralisation is hosted in a polyphase deformed lower greenschist facies orogenic setting, within the low-grade metamorphic core of the Admiralty Subterrane of the Alexander Terrane. The host succession was deposited in a shallow water environment in an evolving intra-arc rift basin. The host setting is represented by a discontinuously exposed, 600 km long belt of rocks comprising a 200 to 800 m thick Triassic rift fill sedimentary sequence, intercalated with bimodal volcanic rocks, and intruded by mafic-ultramafic dykes and sills.
For the setting of the Alexander Terrane (one of the Insular terranes), see Rocky Mountain Tectonic Summary, and for background on the regional setting see the Niblack record.
Six deformation events affect the sequence at Greens Creek: a 273 to 260 Ma Permian pre-mineral compression event (D1), three post-mineral Cretaceous folding phases (F2 to F4), a related shearing event (S2.5), and a mid- to late-Tertiary brittle faulting phase, D5, as described below.
The ore at Greens Creek is discontinuously developed along the strike of what is known as the 'mine contact', a structural/unconformable contact marked by a Late Triassic basal conglomerate/breccia and a narrow suite of dolomitic rocks and dolomite-silica sedimentary breccias. This contact is stratigraphically overlain by a thick section of ~227 Ma, Upper Triassic, graphitic quartz mica phyllite intercalated with dolomite beds. Distal to the deposit, stratigraphically equivalent, 227 Ma tholeiitic basalt with subordinate rhyolite underlie the hanging wall argillite. The 'mine contact' is stratigraphically underlain by a suite of 340 to 330 Ma Carboniferous volcanic and volcaniclastic rocks that include intercalated graphitic sedimentary rocks. This footwall succession is variably altered to chlorite, sericite, quartz and pyrite in the vicinity of massive sulphide of the Greens Creek deposit. The footwall sequence is intruded throughout by serpentinised and carbonate-altered ultramafic rocks. Late Triassic, 219 ±8 Ma gabbroic dykes and sills crosscut both the footwall and hanging wall successions. Basement rocks comprise Neoproterozoic to Devonian quartz-muscovite schists with subordinate felsic volcanic rocks and marble (Steeves, 2018).
The 'mine contact' is variably, but almost continuously mineralised over the deposit area. Three main 'trends' of mineralisation have been traced along the 'mine contact' with multiple centres of mineralisation along those trends. Although the trends are folded, the general mineralisation trends strike at ~160° and plunge 20°S. The distribution of these centres suggests Greens Creek constitutes a cluster of overlapping deposits, controlled by pre-mineral D1 basement faulting. Most of the deposit has been overturned, lying on the lower limb of a large, east vergent, F2 recumbent antiform. A plan of the surface projection of the mineralisation shells of the nine 'mineralised zones' at Greens Creek (see below) covers an area that is ~2.5 km long, and 1 km wide in the north, tapering to 500 m in the centre and the south. The thickness of ore is variable, generally ranging from 0.3 to 10 m, but locally up to 91 m (Jensen et al., 2019). The mineralised zones are in order, from easternmost and highest elevations to westernmost, the East, West, 9A, Northwest-West, Upper Plate, 5250, Southwest, 200 South and Gallagher (Jensen et al., 2019). These zones are described later in the record.
Geology
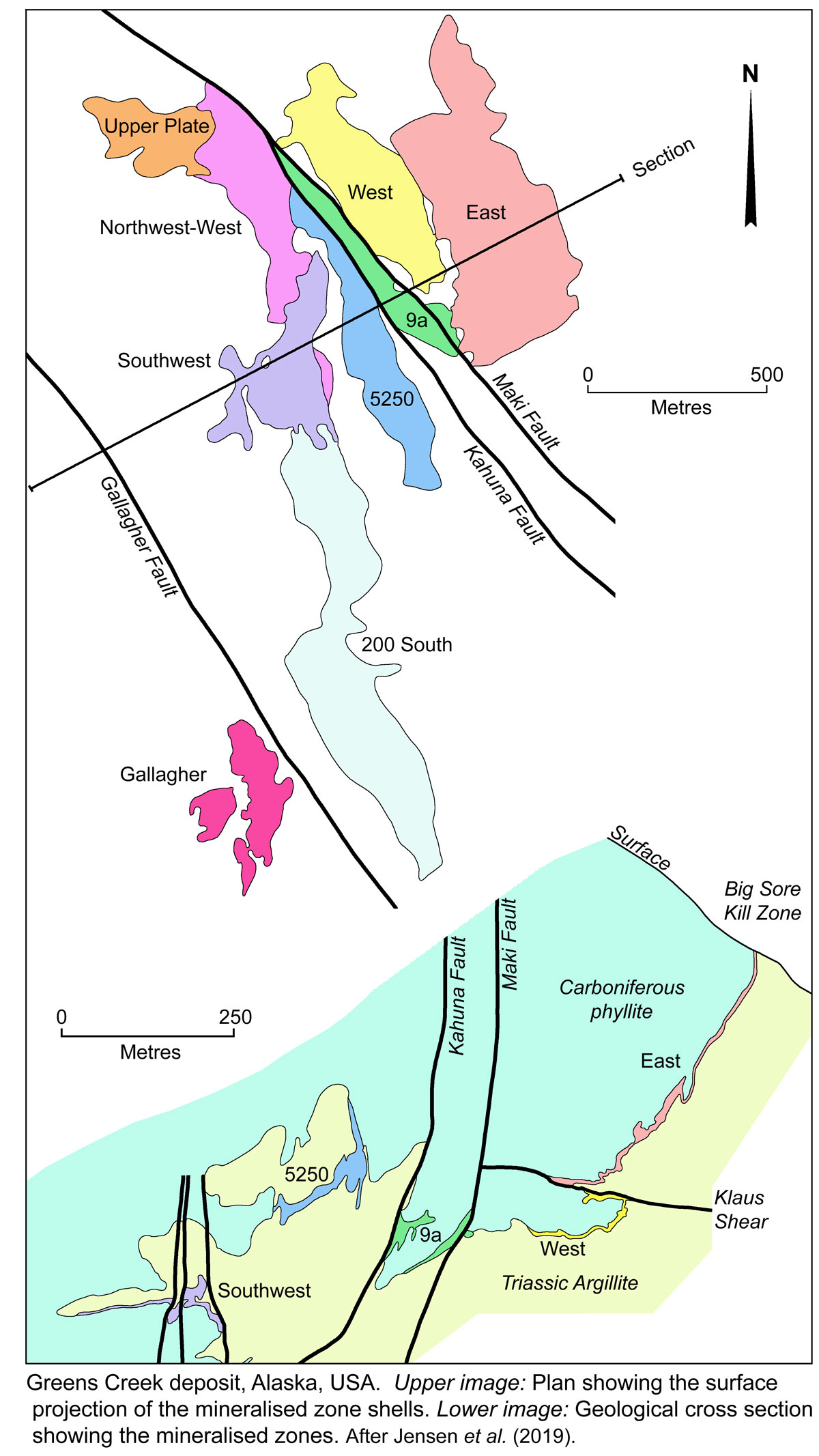
The sequence in the Greens Creek District of the Admiralty Subterrane, from the base (after Steeves, 2018 and in part Duke, Lindberg and West, 2010):
• Neoproterozoic to Mid-Cambrian Retreat Group - which is dominantly composed of quartz-rich schist and semi-schist that locally contain garnet, calcite and/or biotite. The oldest rocks are biotite, chlorite, actinolite, graphite and calcareous schists and amphibolite, intruded by the metamorphosed Ediacaran (545 to 547 Ma) False Point Retreat tonalite and diorite. The rocks of the Retreat Group may correlate with the Wales Group in the Craig Subterrane to the south (see the Niblack record).
• Middle to Late Ordovician to Devonian Hood Bay Formation, from 485 to 393 Ma, which some authors include within, or interpret to be the Retreat Group. It is composed of deep-water black argillite with subordinate black chert (also referred to as black graphitic quartzite), impure black limestone and metre-scale lenses of pillow basalt.
• Devonian Gambier Bay Formation, from 419 to 359 Ma, unconformably overlying the Hood Bay Formation, composed of green chloritic phyllite, interpreted as meta-volcanic rocks, locally intercalated with subordinate black to grey phyllite and marble (Karl et al., 2010; Sack et al., 2016). Near Greens Creek, these rocks form the footwall to the deposit and have been divided by Sack et al. (2016) into coherent mafic metavolcanic and layered meta-volcaniclastic rocks. U-Pb dating of in situ zircon from these rocks at the Greens Creek ranges from Silurian to Devonian and Lower Carboniferous.
• Late Devonian to Permian Cannery Formation, from 419 to 252 Ma, which overlies and partly overlaps the Hood Bay Formation and the Palaeozoic metamorphosed rocks of Gambier Bay Formation. It is composed of chert, greywacke-chert turbidites, volcaniclastic sandstones, and is capped by the Pybus Formation limestone (Karl et al., 2010). The latter formation comprises pale fossiliferous dolostone with minor chert and is up to 300 m thick (Loney, 1964). The Pybus Formation is found in both the Admiralty and Craig subterranes, indicating the two were joined at that time. The contact between the Pybus and Cannery is gradational and interfingering. The formation is strongly deformed and may be several hundred metres thick (Lathram et al., 1965; Karl et al., 2010). It is distinguished from the Hood Bay Formation by its highly carbonaceous black chert, graptolitic argillite, massive mafic volcanic rocks, less intense deformation, and the presence of quartz veins (Karl et al., 2010).
In the immediate Greens Creek area, the Cannery Formation is capped by two metamorphosed, hydrothermally altered, tholeiitic mafic volcanic units, M1 and M2 (Sack et al., 2016) which have been dated in the range 340 to 330 Ma (U-Pb zircon) in the Lower Carboniferous, and comprise the footwall to most of the Greens Creek ore (Sack, 2009). M1 is a massive, commonly feldspar-phyric 'greenstone', whilst M2 is composed of 7 subunits of thinly to thickly layered chloritic-, sericitic-, mariposite-, carbonate-, graphitic- and siliceous-phyllite, as well as siliceous rocks. Preservation of volcanic textures is rare due to their destruction by metamorphism, alteration and deformation. Both have been metamorphosed to upper prehnite-pumpellyite to lower greenschist facies, and their mineralogy and composition are primarily a function of hydrothermal alteration.
• Late Triassic Hyd Group (Loney, 1964; Taylor et al., 2008). Only structural contacts between this unit with the Cannery Formation and underlying rocks have been observed in the Greens Creek area, with the Pybus Formation limestone absent (Karl et al., 2010; Duke et al., 2010). The base of the group is occupied by a polymict breccia containing locally derived angular to rounded clasts of silt to pebble sized clasts of quartz, argillite, altered volcanic and possibly altered ultramafic rocks. The Greens Creek Ore is hosted, in part, by this breccia. This unit is overlain by a thick package of metasedimentary rocks which form the immediate hanging wall to ore. The base of this package is dominated by 5 to 50 cm thick beds of massive black dolostone to argillite of possible evaporitic origin, separated by thin carbonate-poor, graphite-rich siliciclastic phyllite beds, referred to as 'slaty argillite'. Dolomite within the massive black dolostone/argillite may have been derived either by precipitation from hydrothermal fluids, or by hydrothermal alteration of marine carbonate. The massive black dolostone/argillite is cut by abundant white quartz and carbonate ladder veins, that are perpendicular to layering. These veins are characterised by variable bright green Ba-muscovite, bronze cymrite (BaAl2Si2(O,OH)8 ยท H2O) and sulphide, particularly where close to the contact with mineralisation. Apatite veinlets occur near the contact locally and may be related to mineralisation (Gemmell, 2012). Due to deformation, the true thickness of the massive black dolostone/argillite is difficult to determine, although best estimates are several hundreds of metres (Duke et al., 2010). The 'slaty argillite' beds within the black dolostone/argillite range from centimetres to tens of metres in thickness, and appear more frequently near the base of the package.
• Triassic Mafic and Felsic Volcanic Units of the Hyd Group, which are exposed a few kilometres south of the Greens Creek mine portal. A single, ~10 m thick rhyolite body is exposed locally, structurally overlying a thin rhyolite and basalt clast breccia, which in turn, structurally overlies the thick hanging wall Hyd Group dolomite/argillite package. The rhyolite has been dated at 226.86 ±0.24 Ma (U-Pb; zircon; Sack et al., 2011). It is overlain by a basalt unit that is the most extensive Triassic volcanic unit in the deposit area. It is less altered than the footwall mafic volcanic rocks and has preserved primary textures such as pillows and autoclastic breccia. These basalts are correlated with the uppermost basaltic rocks that cap the Alexander Terrane regionally.
• Late Jurassic to Early Cretaceous basalt and sericitic siltstone are exposed on the northeastern coast of Admiralty Island.
Intrusives - Three intrusive units have been recognised by Sack (2009):
• A quartz-carbonate-mariposite which is restricted to the Palaeozoic footwall rocks. It is a light grey, with a green to purple tinge from the presence of mariposite (a green Cr-rich mica) and kämmererite (a purple clinochlore), and is cut by a network of quartz-carbonate veins (Sack, 2009). It is interpreted to represent altered mafic to ultramafic subvolcanic sills and dykes (Freitag, 2000; Sack, 2009)
• A dark green serpentinite which occurs throughout the footwall succession, often associated with large shears and brittle faults, but is also found locally in the hanging wall. It is mainly composed of serpentine, with lesser chlorite, carbonate, mariposite, magnetite, talc and quartz. Individual bodies vary in size and thickness. Where found in the hanging wall, there is a possibility of it having been structurally emplaced.
• Porphyritic gabbro sills and dykes, that are weakly layered to massive, with plagioclase, clinopyroxene and hematite, and altered to chlorite and biotite. These gabbro dykes and sills cut the hanging wall argillite, often have chilled margins, and are interpreted to have fed the Triassic basalt.
Structure
The rocks at Greens Creek have undergone at least six deformation events (Proffett, 2010). A pre-mineral S1 fabric is only found within the footwall Palaeozoic rocks and formed during deformation and metamorphism between 330 and 250 Ma (U-Pb zircon; Sack, 2009; Proffett, 2010, Sack et al., 2016), possibly during thrusting of the Craig subterrane over the Admiralty subterrane (Karl et al., 2010). S1 occurs as segregation layering of quartz-rich and mica-rich layers that predates, and is typically overprinted by the more dominant S2 fabric (Proffett, 2010). It is truncated by the Carboniferous to Triassic 'mine contact' and is recognised within Triassic breccia/conglomerate clasts.
D2, the first post-mineral deformation event, produced tight to isoclinal F2 folds and a related axial planar cleavage (S2), the dominant fabric in the mine area, overprinting all Paleozoic and Triassic rocks of the deposit. S2 is near parallel to bedding (S0) in argillite in fold limbs. Triassic massive argillite beds contain quartz and calcite ladder veins at high angles to, and to a lesser degree parallel to S2 (Figs. 3.4 and 3.5). These veins have not been folded by F2, but are by F3, and likely formed as tension veins parallel the principal stress during F2 shortening (Proffett, 2010). They are both overprinted and offset by S2 pressure solution cleavage, indicating veins and cleavage were coeval.
Flat-lying ductile shear zones (S2.5) found throughout the mine area, typically with a strong mylonitic foliation, postdate and deform S2/F2, but predate and are folded by F3 (Proffett, 2010). Two major shear zones define the mine block; the Upper and Lower shear zones. The Upper shear zone is 3 to 60 m thick, is underlain by ultramafic rocks, and is overlain by Triassic argillite (Proffett, 2010). C-S fabrics indicate displacement as top to the west (Proffett, 2010). Smaller shears are well understood, such as the Klaus Shear that truncates the East Zone ore down dip. It is relatively horizontal, with a top to the NW displacement of ~200 m, and terminates against the post-D4, NW-SE Maki fault zone to the west (Lindberg, 1994). The notation S2.5 has been used by Proffett (2010) to suggest this shearing may be related to the F2 folding event.
The second post-mineral folding event, F3, overprints all previous structures. It produced the most obvious folds observed underground; tight to open structures with axial traces that plunge gently to the south and axial planes that dip moderately to steeply to the east, strike NNW in the northern part of the mine, and north-south in the southern part (Proffett, 2010). Synforms typically have steeply dipping, longer east limbs, and shorter, shallower west limbs. Folds have mine-scale amplitudes of >100 m, with cm- to metre-scale amplitude parasitic folds, mainly in the hinge zones, but less abundant on the limbs (Proffett, 2010). The East Zone lies on the steep eastern limb of an F3 synform, the West Zone is in the hinge, and an antiform and synform pair to the west of the Maki Fault host the other ore zones. S3 is an axial planar cleavage that may crenulate S2 and is variably present. Upper Cretaceous 85 ±4 Ma (Sack 2009) dolerite dykes are unaffected by S2/F2, but are overprinted by a spaced S3 foliation.
The final folding event, F4, is only locally developed, and is most obvious when folding F3 structures. F4 folds are open, have axial planes that dip gently to the south, and fold axes that plunge gently south to SE (Proffett, 2010). S4 is rare, occurring as either a spaced cleavage or a crenulation cleavage (Proffett, 2010). Proffett (2010) noted quartz veins that cut F3 folds but are folded by F4, suggesting an intervening fracturing and veining event. Ductile deformation events from D2 to D4, are related to metamorphism during the mid-Cretaceous collision between the Alexander Terrane and western North America (Himmelberg et al., 1995; Karl et al., 1998; Haeussler et al., 1999). Metamorphism appears to have been short lived, reaching a maximum of prehnite-pumpellyite to lower greenschist facies with chlorite and biotite in mafic volcaniclastic rocks of the Hyd Group (Himmelberg et al., 1995; Sack, 2009; Taylor et al., 2010).
The youngest deformation event, D5, took place during the Mid- to Late Tertiary and involved brittle faulting, which produced three large, evenly spaced, NNW to NW striking and steeply dipping brittle fault zones in the deposit area, namely the Gallagher, Maki and Big Sore fault zones. The similar orientations of D3 ductile and D5 brittle structures suggest the D3 structural grain was in part reactivated in D5 (Jensen et al., 2019). The Maki Fault Zone is ~200 m wide in the south, tapering to 10 m in the north, and exhibits a 'Reidel' shear pattern bounded by the Maki and Kahuna faults to the east and west respectively, and a series of internal fault strands (Lindberg, 1994). Fault strands are manifested by up to 10 m wide zones of soft, sheared, clay-rich gouge and breccia, often containing abundant graphitic material. Locally these strands may contain very high grade precious metals. Displacement along the Maki Fault has been calculated at ~550 m of dextral offset and 34 m of east side down vertical movement (Lindberg, 1994). This displacement gives a rake of ~3.5° to the south, consistent with slickensides mapped underground (Lindberg, 1994). The parallel Gallagher fault zone, ~1 km to the SW, has two main strands (East and Middle Gallagher) that taper at depth to form a single fault plane. On the basis of offset on post-Triassic dolerite dykes, Proffett (2004) calculated a horizontal dextral displacement of 830 m and reverse, west up offset of ~200 m along the fault zone. The parallel Big Sore Fault, ~750 m NE of the Maki Fault, is less well understood, although it defines a topographic lineament and is shown to have dextral offset (Duke et al., 2010). Unlike the Maki and Gallagher fault zones, the Big Sore Fault Zone does not intersect mineralisation. Dextral brittle faulting around Greens Creek is likely related to the mid- to late-Tertiary Denali Fault System, manifested near Admiralty Island as the Chatham Strait fault (Duke et al., 2010).
Mineralisation and Alteration
Nine orebodies, or mineralised zones, (as listed earlier) have been outlined, separated by faults, shear zones, or by structural attenuation. The ore is generally stratabound, and occurs in contact with both Late Triassic hanging wall massive dolostone to argillite, and footwall 'phyllite' of the Devonian to Permian Cannery Formation. These contacts are relatively sharp, and often weakly sheared or fault juxtaposed.
Mineralised lithologies fall into two broad groups, i). massive ores with >50% sulphides and ii). white ores with <50% sulphides. These are further divided into 5 styles, which within individual orebodies have gradational contacts and represent end members. They are:
• Massive pyrite-rich, in which the pyrite content exceeds that of all other sulphides. The dominant sulfide minerals are, in decreasing order of abundance: pyrite, sphalerite, galena, chalcopyrite, arsenopyrite, and tetrahedrite with common electrum. Gangue minerals are carbonate, quartz, barite, barian muscovite (ganterite/oellacherite), fuchsite, graphite, and locally cymrite. The gangue mineralogy varies depending on adjacent ore styles. Textures vary and include compositionally layered, massive, domainal and brecciated. Fine layering is common in zones of abundant barite, muscovite, quartz and base metal sulphide, occurring as discontinuous mm- to cm-scale alternating pyrite-rich and base metal-rich or gangue-rich layers;
• Massive base metal-rich, where pyrite is less than other combined sulphides, comprising in decreasing order of abundance: sphalerite, pyrite, galena, tetrahedrite, minor chalcopyrite and colusite (Cu13VAs3S16), with rare arsenopyrite and common electrum. Gangue mineralogy includes dominant quartz, barite or carbonate, and may include muscovite, mariposite, cymrite, albite and rare chlorite. Graphite is common. Most is compositionally layered, although massive, domainal and brecciated textures are also observed. The layered ore has banding that varies from cm-scale to sub-mm laminations that are locally intercalated with boudinaged and veined sedimentary layers. In some cases, sulphide bands can be up to several tens of cm thick, have a homogeneous, massive texture, and contain argillite clasts. Durchbewegung textures are common in more deformed areas with breccia like textures comprising µm- to cm-scale pyritic clasts in a sphalerite and galena ±gangue matrix. Sphalerite and galena are frequently strongly recrystallised and coarser-grained than pyrite. Argillite clasts are common, typically surrounded by abundant graphite which occurs interstitial to the surrounding sulphide grains. These clasts are interpreted to represent remnants of the host rock, with the more permeable layers preferentially replaced and more impermeable, finer-grained and graphite-rich layers brecciated and boudinaged.
• White barite-rich, where sulphides are <50% and a barite gangue predominates. Sulphides, in decreasing order of abundance include: pyrite, sphalerite, galena, tetrahedrite, colusite, proustite-pyrargyrite, stromeyerite and chalcopyrite, with local trace pearceite-polybasite. A distinct Cu-Ag assemblage of stromeyerite and covellite, often accompanied by bornite, enargite, tennantite, chalcocite and electrum, is common in local, metre-scale zones, occurring as disseminations, late cross-cutting veins, or with replacement textures. Compositional banding is the dominant texture, although
massive and breccia textures are locally evident. Banding is on a scale of mm to tens of cm of alternating barite- and sulphide-dominant layers, with the thicker bands being homogenous and massive. These layers are discontinuous and can generally not be followed for more than a few metres. Boudins of white to grey, mm- to cm-scale, barite ±carbonate are found throughout the banded mineralisation, and often contain coarser grained concentrations of mobile minerals, e.g., proustite-pyrargyrite, tetrahedrite and galena. Clasts of argillite and/or abundant graphitic material are commonly found in this ore style. Where carbonate is abundant, massive and heavily veined textures dominate, forming a breccia texture. The veins are predominantly composed of barite, carbonate, tetrahedrite and galena, ±other sulphosalts and mobile sulphides such as enargite, proustite-pyrargyrite and pearceite-polybasite. They resemble layer-parallel boudins of remobilised barite and sulphide and locally may include the Cu-Ag-S assemblage minerals. These carbonate-rich layers are intercalated with laminated baritic layers and locally slaty argillite layers.
• White carbonate rich, with <50% sulphides, dominated by a carbonate gangue, which is principally composed of dolomite with lesser locally distributed calcite and Ba-carbonate minerals. The main sulphides in decreasing order of abundance include pyrite, sphalerite, galena, tetrahedrite and chalcopyrite, with rare electrum. Other gangue minerals comprise barite, quartz, graphite, cymrite and muscovite. The ore is grey and blocky, composed of massive, variably recrystallised carbonate with disseminated sulphide, and is pseudo-brecciated by meandering, diffuse, sulphide stockworks dominated by pyrite, and by abundant discontinuous, irregularly shaped white carbonate veins. The carbonate groundmass is massive, but is variable in colour, texture and grain size, suggesting multiple dolomitisation or recrystallisation events. Pyrite occurs has framboidal, colloform, skeletal, radiating and open space-fill textures. Stylolites and pressure solution bands are common, with many pyritic veins filling stylolites that contain abundant framboids and graphitic material. White carbonate tension veins do not cross pyritic bands, and often contain green Ba-muscovite, chalcopyrite, pyrite and tetrahedrite. Locally, this ore style contains up to 20 vol.% vein material. Layers of White carbonate rich ore range from several cm to tens of metres in thickness and occur stratigraphically
above, below, or within other mineralization styles.
• White siliceous, also contains <50% sulphides and has a dominant fine-grained, hard quartz gangue. The main sulphides include, in decreasing order of abundance: pyrite, sphalerite, galena, tetrahedrite, chalcopyrite, colusite and locally bornite and chalcocite, with rare electrum. Other gangue includes carbonate, minor graphite, albite, muscovite and rare barite. This ore style occurs in three stratigraphic locations, each with its own distinct texture: i). occurring as local, <1 m thick siliceous horizons between the hanging wall argillite and the ore, where it commonly contains significant graphitic material and is typically pyrite-rich. Stratigraphy above and below are locally tightly folded into these horizons; ii). layers within sections of the massive pyrite-rich style that contains <50% sulphide and where quartz is the dominant gange. The resultant layer is composed of alternating carbonate- and quartz-dominant layers. Microtextures show quartz partially replacing carbonate locally, and infers partial silicification of a previous carbonate rock; iii). which are zones that are up to several metres thick at the base of the mineralised horizon, generally with a gradational contact with overlying massive sulphides, and occur as quartz-altered host rock that has been pseudo-brecciated by a sulphide stockwork. Siliceous clasts within this 'breccia' are typically matrix supported, have a jigsaw fit, without significant rotation due to deformation. Clasts often contain white quartz tension veins that are perpendicular or parallel to S2 layering and are folded by F3 folds. The transition from this ore style to barren quartz-altered footwall is not well defined.
• Footwall quartz-carbonate-chlorite-sulphide veining, is found locally within chloritic and sericitic altered rocks, immediately below the main sulphide ore and the 'mine contact' and contains semi-massive sulphides. These veins carry sphalerite, galena, chalcopyrite and pyrite, and occur at angles to and are overprinted by S2. They are typically several cm thick and folded, and occupy a relatively thin interval sub-parallel to the footwall/hanging wall 'mine contact'. They immediately underlie most of the mined zones, and overlap the lower white-siliceous mineralisation style, although their distribution and abundance is not well documented (Steeves, 2018).
This mineralisation has been shown to be zoned over several kilometres of strike length, with a more siliceous, high temperature, copper-rich core north of the mine area, while only the zinc-rich and copper-poor portion underlies the preserved section of the deposit (Jensen et al., 2019).
Jensen et al. (2019) suggest this pattern is also reflected in the main massive sulphide ore, and that rather than the Greens Creek hydrothermal system being low in copper overall (as the reserves suggest), only the cooler zinc, lead and silver southern section has remained un-eroded. Zonation within the preserved mineral deposit indicates a hotter copper-rich core on the northern end and cooler baritic mineral styles on the southern end. The mineral styles do not zone back to cooler copper-poor types north of the Greens Creek mineral body as they were eroded off above the copper rich footwall zone north of Greens Creek (Jensen et al., 2019).
Both Steeves (2018) and Jensen et al. (2019) interpret this zone of footwall veining to represent a feeder zone to the mineralised centres.
Steeves (2018) and Jensen et al. (2019) describe an idealised zonation of these ore styles, distributed around centres of mineralisation. The core of each centre is focussed on a zone of 'massive pyrite-rich sulphides' characterised by Fe-Ti-As/Sb ±Au, passing down into the stratigraphic footwall 'white siliceous' style and 'footwall quartz-carbonate-chlorite-sulphide veining' to 'semi-massive sulphides'. This footwall mineralisation contains Fe-Cu sulphides and has a higher Cu:Fe ratio. The central 'massive pyrite-rich sulphides' pass outwards, laterally, into 'massive base metal-rich sulphides', which in turn grades outward and interfingers with 'white carbonate rich sulphides' containing an assemblage with Zn-Pb-Au-Ag-Sb-Hg. This zone then grades into a peripheral annulus of massive and layered baritic mineralisation.
Four such centres of mineralisation have been recognised along the linear mineralised trend. The largest of these massive pyrite and base metal sulphide centres is focussed on the West and Northwest-West zones, with two more in the Southwest and upper 200 South zones, and yet another in the deeper and more southern section of the 200 South Zone. Others are suggested further to the south and deeper still. The massive pyrite- and massive base metal-sulphides, and the barite rich zones typically have the highest grades, the latter being particularly silver rich, and sometimes has high sphalerite contents. The lowest grades are within the white siliceous style.
Four Hydrothermal alteration zones have been defined, reflecting a general increase in muscovite and quartz towards massive sulphide accumulations. These zones are, from distal to proximal:
• Chlorite, which affects the massive M1 metabasalt, with minor sericite, carbonate and quartz. Much of the chlorite is interpreted to have formed by greenschist facies metamorphism of the submarine mafic volcanic rocks and a such is not mineralisation related alteration.
• Sericite, which dominates in the main immediate footwall M2 metabasalt, that is now a sericitic phyllite.
• Quartz-sericite, which represents a transition from sericite- to quartz-dominated alteration of the M2 metabasalt with increasing proximity to ore. The protolith of the most intense alteration is not known, although lenses that are several metres thick of monomict breccia occur locally at the ore contact, and are defined as intense quartz-pyrite alteration cut by sulphide veins. Graphitic footwall phyllites, which are thin, finely layered, dark grey units within M1 and M2, have been variably quartz and sericite altered.
The following descriptions of the mineralised zones is drawn from Jensen et al. (2019) and affords an impression of the distribution of mineralisation in the nine orebodies and the structural complexity of the deposit:
The East Zone outcrops near the 'Big Sore' gossan, and on its eastern edge, dips at 60 to 80°W and is overturned. It is basically one long, steeply dipping limb, that shallows and folded to horizontal near its base where it is truncated by the flat lying Klaus Shear. Mineralisation varies for 0.3 to 9.0 m in thickness. The Klaus Shear and related F2 fold deforms and truncates the East Zone, and offsets it ~200 m to the NW, over the top of the West Zone. The West Zone emerges below the Klaus Shear and is a faulted extension of the East Zone. A tight F2 fold rotates the West Zone from a nearly flat orientation to a nearly vertical wall dipping steeply to the west, below the Klaus Shear. The thickness of mineralisation is very highly variable, from <3 to >91 m in its central portions. It has a well developed mineralogical zonation with silver rich fringes around a central high Fe:Cu and high Zn:Pb ratioed core of massive sulphides, with baritic peripheries. The East and West zones are bounded on the west by the NW-SE trending Maki Fault system which offsets the mineralised horizon to the north in a dextral sense.
The western deformation boundary of the Maki Fault tends to be a continuous fault splay known as the Kahuna Fault. The mineralisation hosted within the fault zone is referred to as the 9A Zone. The general orientation of the mineralised body strikes NW and dips steeply to the west, although many internal fault splays cut the mineralised unit into sheets with differing orientations. In plan, mineralised widths vary from <1.5 up to 30 m. This zone represents the fault-bounded connection between the East, West and Northwest-West zones, and contains elements of each, including base metal and pyritic massive sulphides with lesser carbonate, siliceous and baritic material intermixed.
West of the Kahuna Fault, the Northwest-West Zone represents the offset portion of the West Zone. It is structurally dominated by a pair of recumbent F2 folds, an upper hanging wall argillite-cored syncline, while the lower fold is a footwall phyllite-cored anticline. Mineral types and mineralization are similar to that of the West Zone, mainly base metal and pyrite massive sulphides with some carbonate and silica style intermixed. IN the NW of the zone, mineralisation climbs up to 30 m above the 'mine contact' into the hanging wall argillites where the mineral styles become a mixture of mostly massive sulphides and white-siliceous types, with lesser carbonate and baritic mineralisation styles.
The Upper Plate is a smaller body in the far northern end of the deposit, located structurally above the Northwest-West Zone. It occurs on the upper limb and hinge of a very large recumbent F2 isoclinal fold, on the underside of the major D2.5 Upper Shear Zone. It strikes ~50° and generally
dips at ~15°S. Mineralisation is mostly restricted to the upper and lower contacts of the fold, but does in places cross into the argillite core. Mineralisation is relatively thin but is generally base metal massive sulphides.
The 5250 Zone is found immediately west of the Kahuna-Maki Fault system and is interpreted to represent a faulted offset of the upper mineralised trend in the East Zone. It is a lower temperature lens of barite-rich mineralisation, with lesser massive sulphides, and minor carbonate and siliceous material. It links to the north with the Northwest-West Zone. Silver grades are typically above average. The northern half is interpreted to be an antiformal F2 structure with a 30°WSW dipping western limb and an ~80°W dipping second limb, whilst to the south the eastern overturned
limb pinches out and the upright limb thickens. Overall, the zone has a length of ~900 m, is ~200 m wide and up to 30 m thick. It plunges at ~20° with an azimuth of 150° (Steeves, 2018).
The Southwest Zone comprise a large footwall phyllite cored F2 antiform with a nearly horizontal hanging wall argillite syncline (also F2) on its upper limb. The lower limb of the antiform is steeply east dipping to moderately west dipping, increasing with depth. Mineralisation wraps around the structure's 'mine contact'. Some late F3 folding has significantly deformed the 'mine contact' on the limbs of the synform. It has been subdivided into a northern Upper and a southern Lower Southwest. The Upper Southwest Zone comprises several thin mineralised bands along several tight F2 folds. The Lower Southwest is a continuous sheet folded by 3 major F2 folds and contains ore thicknesses of up 25 m in the fold hinges, tapering to 3 m on the limbs. The orebody is cut by several near vertical D5 brittle faults with dextral displacement of >100 m and by several low angle S2.5 shears.
The Southwest Zone continues down plunge and passes directly into the 200 South Zone. The boundary between the two is arbitrary, mainly to divide it into two smaller areas to simplify mine modelling. The 200 South Zone has the same general antiformal geometry as the Southwest Zone, with a steeply dipping eastern and flat-lying western limb. F2 folding is overprinted by an F3 fold with an east-dipping axial plane, while a major flat D2.5 shear has an offset of top to the NW. Mineralisation is bounded on the east by a steep, brittle fault that offsets the 'mine contact' by 75 to 100 m in a dextral sense. On the northern end of the zone, a mixed group of mineral types is present, including pyrite and base metal massive sulphides, carbonate- and barite-rich mineralisation, whilst at the southern end, baritic mineralisation dominates with high silver grades. The lower limb mineralisation follows the 'mine contact', varies between 1.5 and 6 m in thickness, and dips moderately to the SW. The upper limb extends ~400 m further south than the lower limb and is typically between 0.5 and 3 m thick, and is tightly folded, repeating the sequence in at least 2 flat-lying, isoclinal F2 folds, and/or is also affected by shearing (Steeves, 2018).
The Gallagher Zone lies to the west of the 200 South Zone and is west of a second major fault zone known as the Gallagher Fault, has a dextral displacement of ~830 m and reverse offset of ~200 m. Offset on a post-mineral dyke swarm, the trend of the 200 South Zone into the Gallagher Fault and the similar structural and chemical styles between the southern 200 South and Gallagher mineral zones all indicate that the Gallagher Zone is simply the fault offset of the 200 South Zone. The ore horizon is folded by an overturned west dipping antiform, cored by quartz- and/or carbonate altered Triassic argillite. Most ore occurs on the upper flat-lying limb and the hinge of the antiform. Further south, ore is mainly on the lower limb and in the hinge of an underlying syncline.
Reserves and Resources
Reserves in 1992 amounted to - 12.5 Mt @ 12.8% Zn, 4% Pb, 140 g/t Ag, 4.3 g/t Au, 0.5% Cu, after having been mined from 1989.
Ore Reserves and Mineral Resources remaining at the end of 2003 (Tio Tinto Annual Report, 2003) were:
Probable Ore Reserve - 6.8 Mt @ 10.7% Zn, 4.02% Pb, 3.95 g/t Au, 483 g/t Ag, (No Proved Reserve calculated)
Indicated + Inferred Resources of 4.5 Mt @ 7.2% Zn, 2.9% Pb, 3.9 g/t Au, 399 g/t Ag,
TOTAL Mineral Resources+ Ore Reserve - 11.3 Mt @ 9.3% Zn, 3.6% Pb, 3.9 g/t Au, 450 g/t Ag.
Note: Resources are exclusive of Reserves.
Taylor and Johnson (2010; USGS Professional Paper 1763) quoted a global resource at Greens Creek of:
(24.2 M tons) 21.95 Mt @ 13.9% Zn, 5.1% Pb 5.14 g/t Au, 660 g/t Ag at a zero cutoff).
Note these figure were quoted as tons and troy ounces per ton. Tons is assumed to be US short tons, and have been converted to international units of tonnes and grams per tonne on that assumption.
Ore Reserves and Mineral Resources as at 31 December, 2020 (Hecla Mining Company, Website Reserves and Resources Statement - viewed August 2021) were:
Proved + Probable Ore Reserve - 8.145 Mt @ 7.3% Zn, 2.8% Pb, 3.0 g/t Au, 425 g/t Ag;
Measured + Indicated Mineral Resources - 8.069 Mt @, 8.2% Zn, 3.0% Pb, 3.4 g/t Au, 442 g/t Ag;
Inferred Mineral Resources - 1.603 Mt @ 7.0% Zn, 2.8% Pb, 2.75 g/t Au, 452 g/t Ag;
TOTAL Mineral Resources+ Ore Reserve - 17.817 Mt @ 7.7% Zn, 2.9% Pb, 3.2 g/t Au, 435 g/t Ag.
Note: Resources are exclusive of Reserves. These figure were quoted as tons and troy ounces per ton. Tons is assumed to be US short tons, and have been converted to international units of tonnes and grams per tonne on that assumption.
Total production milled from 1989 to 2018 was 16.320612 Mt @ 10.12% Zn, 3.95% Pb, 4.79 g/t Au, 566 g/t Ag to recover 1.306 Mt of zinc, 0.459 Mt of lead, 6967 t of silver and 51.5 t of gold (Jensen et al., 2019).
The information in this summary is largely drawn from Steeves, N.J., 2018 - Mineralization and Genesis of the Greens Creek Volcanogenic Massive Sulfide (VMS) Deposit, Alaska, USA; A thesis submitted in fulfilment of the requirements for the degree of Doctor of Philosophy, University of Tasmania, Hobart, Australia; 416p. and from Jensen, P., McDonald, D., Mehalek, K., Blair, K., Hancock, W., Butikofer, M.D. and Glader, P., 2018; Technical Report for the Greens Creek Polymetallic Mine Alaska USA; an NI 43-101 Technical Report prepared by Hecla Mining Company, 366p.
The most recent source geological information used to prepare this decription was dated: 2019.
Record last updated: 16/8/2021
This description is a summary from published sources, the chief of which are listed below. © Copyright Porter GeoConsultancy Pty Ltd. Unauthorised copying, reproduction, storage or dissemination prohibited.
Greens Creek
|
|
Duke, N.A., Lindberg, P.A. and West, A.W., 2010 - Geology of the Greens Creek Mining District: in Taylor, C.D. and Johnson, C.A., (Eds.), 2010 Geology, geochemistry, and genesis of the Greens Creek massive sulfide deposit, Admiralty Island, southeastern Alaska: U.S. Geological Survey, Professional Paper 1763, Ch.4, pp. 93-106.
|
Freitag K, Boyle A P, Nelson E, Hitzman M, Churchill J and Lopez-Pedrosa M 2004 - The use of electron backscatter diffraction and orientation contrast imaging as tools for sulphide textural studies: example from the Greens Creek deposit (Alaska): in Mineralium Deposita v39 pp 103-113
|
Proffett, J.M., 2020 - Geologic Structure of the Greens Creek Mine Area, Southeastern Alaska: in Taylor, C.D. and Johnson, C.A., (Eds.), 2010 Geology, geochemistry, and genesis of the Greens Creek massive sulfide deposit, Admiralty Island, southeastern Alaska: U.S. Geological Survey, Professional Paper 1763, Ch.7, pp. 141-162.
|
Taylor C D, Premo W R, Meier A L and Taggart J E, 2008 - The Metallogeny of Late Triassic Rifting of the Alexander Terrane in Southeastern Alaska and Northwestern British Columbia: in Econ. Geol. v103 pp 89-115
|
Taylor, C.D., Philpotts, J., Premo, W.R., Meier,A.L. and Taggart, J.E., 2010 - The Late Triassic Metallogenic Setting of the Greens Creek Massive Sulfide Deposit in Southeastern Alaska: in Taylor, C.D. and Johnson, C.A., (Eds.), 2010 Geology, geochemistry, and genesis of the Greens Creek massive sulfide deposit, Admiralty Island, southeastern Alaska: U.S. Geological Survey, Professional Paper 1763, Ch.2, pp. 13-59.
|
Taylor, C.D., West, A.W., Lear, K.G., Hall, T.E. and Proffett, J.M., 2010 - Geology and Metal Zoning of the Greens Creek Massive Sulfide Deposit, Southeastern Alaska: in Taylor, C.D. and Johnson, C.A., (Eds.), 2010 Geology, geochemistry, and genesis of the Greens Creek massive sulfide deposit, Admiralty Island, southeastern Alaska: U.S. Geological Survey, Professional Paper 1763, Ch.6, pp. 125-140.
|
Porter GeoConsultancy Pty Ltd (PorterGeo) provides access to this database at no charge. It is largely based on scientific papers and reports in the public domain, and was current when the sources consulted were published. While PorterGeo endeavour to ensure the information was accurate at the time of compilation and subsequent updating, PorterGeo, its employees and servants: i). do not warrant, or make any representation regarding the use, or results of the use of the information contained herein as to its correctness, accuracy, currency, or otherwise; and ii). expressly disclaim all liability or responsibility to any person using the information or conclusions contained herein.
|
Top | Search Again | PGC Home | Terms & Conditions
|
|