Kalgoorlie Gold Field - KCGM Fimiston Super Pit, Golden Mile, Mount Charlotte (Reward, Charlotte, Maritana, Charlotte Deeps), Hannans North, Mount Percy |
|
Western Australia, WA, Australia |
Main commodities:
Au
|
|
 |
|
 |
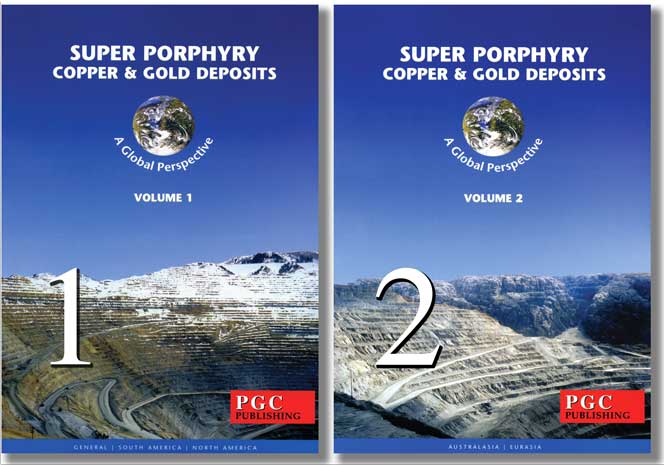 |
Super Porphyry Cu and Au

|
IOCG Deposits - 70 papers
|
All papers now Open Access.
Available as Full Text for direct download or on request. |
|
 |
The KCGM Super Pit at Fimiston exploits the Golden Mile deposit within the Kalgoorlie Gold Field, ~600 km ENE of Perth. The Mount Charlotte deposit, 3 km to the NW, is made up of four orebodies Reward, Charlotte, Maritana and Charlotte Deeps. Other deposits mined in the gold field include Mount Percy, ~1.5 km north of Mount Charlotte and Hannan's North, which is 750 m NW of Mount Percy (#Location: Fimiston Superpit 30° 46' 39"S, 121° 30' 10"E).
Gold was first discovered in what was to become the Kalgoorlie Gold Field in June 1983 by three Irish prospectors, Dan Shea, Tom Flanagan and Paddy Hannan. Hannan and Flanagan had originally come to the area during the Coolgardie gold rush in 1889. By 1893, the alluvial gold at Coolgardie was largely exhausted and only one significant reef was being exploited. News of a 'good discovery' at a place called Mount Yuille started a rush in June 1893, and Hannan, Flannagan and O'Shea joined in. En route they prospected what they regarded as prospective ground, and on June 10, 3 days after leaving Coolgardie they found 'colours of gold' and then encountered 'good gold' from the north end of Mount Charlotte to south of Maritana Hill. In a few days they collected 3 kg of gold at surface, and Hannan set out for Coolgardie and lodged a Reward Claim application on June 17 1893. This discovery sparked a new rush with initial prospecting and mining activity concentrated at the northern end of the Kalgoorlie field where gold occurred in quartz veins and as surficial alluvial/colluvial concentrations familiar to prospectors. Two years elapsed before the full significance of the relatively inconspicuous ferruginous, oxidised outcrops after sulphide and carbonate rich ores to the south was recognised. However, when it was, that part of the gold field, which became known as the Golden Mile, was rapidly developed (Clout et al., 1990). The gold field has been exploited continuously since Hannan's discovery in1893.
In 1896 Kalgoorlie was connected to Perth by rail. In 1903 the Goldfields Water Supply Scheme was completed with a pipeline to provide water from the Mundaring Weir 566 km to the SW, near Perth. By 1908, ~100 headframes studded the Golden Mile and beneath the ground there were >3000 km of workings. Production from the gold field reached a peak in 1903 with 38.124 t of gold extracted from ore at an average grade of 41.1 g/t Au, predominantly from gold-telluride lodes (Blainey, 1993). Subsequently, production declined due to a combination of factors, including fixing of the gold price, rising costs as lodes were followed underground and ores became more refractory. At the same time, many smaller mines were acquired and consolidated into the larger operations. By then the gold field had become well established and production continued from ten major shafts and numerous other smaller operations. Increasing inflation and lack of manpower during and following the First World War had a negative influence on mining activity, although a re-evaluation of gold in 1932-33 sparked a short-lived boom. Production declined again in the 1960s, leading to cessation of mining on the Golden Mile in 1975, although production continued from the Mt Charlotte mine due to the introduction of low-cost mechanised mining. The floating of the gold price in late 1973, and the marked rise in price from 1979 sparked a resurgence in mining, and the Golden Mile operations progressively reopened with new open-cut mines developed.
Throughout its history, the mines of the Kalgoorlie Gold Field had been progressively consolidated into fewer and larger operations until in 1989, when Bond International Gold (Bond) attempted to consolidate the final three main remaining companies, Gold Mines of Kalgoorlie Ltd (GMK), North Kalgurlie Mines Ltd (NKM) and Kalgoorlie Lake View Pty Ltd (KLV) into one operation. By 1989 Bond had gained control of GMK and KLV and a number of other joint ventures over much of the Golden Mile. In April 1989 Bond sold half of its interests to Homestake Gold of Australia Limited, who subsequently separately purchased all the shares of NKM. Bond and Homestake then each controlled 50% of three joint ventures, Mount Percy, Fimiston/Paringa and Kalgoorlie Mining Associates (KMA) that controlled a complex mosaic of mining leases, mining operations and treatment facilities that covered the Golden Mile and Mount Charlotte deposits. The Kalgoorlie Consolidated Gold Mines Joint Venture (KCGM) was formed in 1989 to amalgamate the various mining operations and treatment facilities by combining the mines of the Golden Mile into the single Fimiston Superpit and operating Mount Charlotte as a separate underground mine. In August 1989, the remainder of the Bond Kalgoorlie interests held through GMK were acquired by Normandy Australia. In December 2001, Canadian company Barrick Gold Corporation merged with Homestake and in February 2002, Normandy was acquired by Newmont Mining Corporation. Since then, Barrick and Newmont have been the equal joint venture owners of KCGM and the Golden Mile and Mount Charlotte mining leases. In November 2019, Barrick Gold Corporation agreed to dispose of its interest in KCGM through the sale of 100% of the shares in Barrick (Australia Pacific) Pty Limited to Saracen Mineral Holdings Limited. In December 2019, Newmont Goldcorp agreed to sell its 50% interest in KCGM to Northern Star Resources Limited. Northern Star Resources and Saracen Mineral Holdings merged in February 2021, to operate as Northern Star Resources.
Regional setting
The Kalgoorlie Gold Field, which occupies and area of ~10 x 2 km, lies within the southern half of the NNW-SSE aligned Neoarchaean, Kalgoorlie granite-greenstone Terrane. This terrane is the western-most tectonic element of the Eastern Goldfields Superterrane that forms the eastern half of the Yilgarn Craton. The Kalgoorlie Terrane forms a NNW-SSE trending strip that is exposed over a width of ~50 to 120 km and length of ~800 km. It is separated from the older Youanmi Terrane to the west by the major east dipping Mount Ida Fault and from the similar Kurnalpi Terrane to the east by the Ockerburry Shear Zone which also dips east. The Kalgoorlie and Kurnalpi terranes were deposited after ~2810 Ma in an extensional rift setting flanked by the Youanmi and Burtville terranes that represent the proto-Yilgarn craton. Granites occupy ~70% of the solid geology of the Eastern Goldfields Superterrane to a depth of at least 15 km, enclosing and underlying a supracrustal succession of bimodal ultramafic-mafic and felsic volcanic rocks in variable proportions, with interbedded volcaniclastic and clastic rocks. The granites and greenstone successions of the Kalgoorlie and other terranes across the Yilgarn Craton developed during three distinct thermal/magmatic events at ~3.1 to 2.9, ~2.8 and ~2.76 to 2.60 Ga.
For more detail see the Yilgarn Craton overview record.
Geology
The southern half of the Kalgoorlie Terrane, contains a Neoarchaean greenstone succession overlying and intruded by large volumes of granite, as follows, from the base (after Gauthier et al., 2005; Vielreicher et al., 2016; Phillips et al., 2017):
Kambalda Sequence which comprises a 1500 to 4000 m thick, 2715 to 2690 Ma mafic-ultramafic suite consisting of the:
• Kambalda Komatiite which consists of channelised and overbank spinifex and cumulate textured komatiitic volcanic rocks with individual flows that are up to several metres thick. It is geochemically primitive with minimal crustal contamination, and contains zircons dated at 2708±7 and 2702±7 Ma. It has been subjected to carbonate alteration in the deposit area that includes magnesite and dolomite. Only the upper part of the unit, the >700 m thick Hannan's Lake Serpentinite is is found at Kalgoorlie, where it comprises komatiite flows ranging from picrites to peridotites.
• Devon Consols Basalt, a 50 to 200 m thick sequence of siliceous, high-Mg basalts, interpreted to represent a crustally contaminated komatiite. It has a variolitic texture, with pillowed, flow breccias to massive flows. Where altered is, it has a carbonate assemblage of dolomite and ankerite.
• Kapai Slate, a 1 to 20 m thick, sulphidic, tuffaceous black shale to mudstone marker unit recognised over strike intervals of tens of kilometres. Dated at 2692±4 Ma (U-Pb zircon; Claoué-Long et al, 1988). Carbonate alteration in the deposit area includes calcite and ankerite.
• Williamstown Dolerite Sill, a 150 to 250 m thick sub-volcanic ultramafic gabbro sill, which is geochemically distinct from the tholeiitic sills in the district. It has a bimodal composition, with lower ultramafic and upper gabbroic layers, and has been dated at 2696±5 Ma (U-Pb zircon; Fletcher et al, 2001). It grades laterally into extrusive facies and in mineralised areas has been altered to dolomite and ankerite.
• Eureka Dolerite Sill, a 100 to 200 m thick, differentiated gabbroic subvolcanic sill that is co-magmatic with the high-iron tholeiitic Paringa basalt. It is characterised by a fine to medium grained ophitic texture with chlorite clots after pyroxene that are mantled by carbonates (Bateman et al., 2001). Thin granophyric layers with quartz crystals occur towards the top of the thicker part of the sill. It is intruded within, and is geochemically similar, to the high iron tholeiite unit of the Paringa Basalt (see below). The sill has a gradual normal fractionation trend, with the upper sections being more fractionated and relatively more enriched in incompatible elements such as Zr, Ti, V and P. It is also geochemically distinct from the Golden Mile Dolerite with higher contents of incompatible elements.
• Paringa Basalt, a 300 to 900 m thick pile of basaltic flows grading from a high magnesium basalt (>10 wt.% MgO) with ubiquitous variolitic and local spinifex textures at the base, to a gradually more fractionated tholeiitic basalt (3 to 10 wt.% MgO; Bateman et al., 2001). The uppermost section is characterised by pillow and flow breccia textures and a general increase in interflow sedimentary rocks, mainly finely bedded black shale. The upper section varies from 50 to 300 m in thickness from north to south within the gold field, and consists of a high-iron tholeiite, in sharp contact with the underlying tholeiitic basalt. The contact is usually occupied by a 1 to 5 m thick finely bedded black shale horizon. This high-iron tholeiite is characterised by high TiO2 of 1.5 to 1.8 wt.%, Fe2O3 of 14 to 16 wt.%; Zr of 100 to 130 ppm; P2O5 of 0.2 to 0.25 wt.%; and low MgO of 3 to 5 wt.%, and has a normal fractionation trend. The thicker section of high-iron tholeiite in the south and on the western limb of the Kalgoorlie Syncline is intruded by the ~100 m thick co-magmatic Eureka Dolerite sill. In the eastern section of the Golden Mile, the high-iron tholeiite below the Golden Mile Dolerite, hosts the bulk of the economic Fimiston style lodes (see Mineralisation section below) that occur within the Paringa Basalt (Travis et al., 1971). The basalt has been dated at 2690±5 Ma (U-Pb zircon; unpublished, quoted by Vielreicher et al., 2016). Where altered, it comprises an assemblage that includes ankerite, siderite calcite and dolomite.
The Kambalda Sequence has numerous interbedded interflow sedimentary rocks, and is overlain by the Kalgoorlie Sequence.
Kalgoorlie Sequence, deposited between 2690 and 2660 Ma (Blewett et al., 2010) as follows:
• Oroya Shale which overlies the Paringa Basalt, and where present separates it from the Golden Mile Dolerite sill. Vielreicher et al. (2016) regard this unit as the uppermost of the Kambalda Sequence, whilst Phillips et al. (2017) suggest it is the lowermost member of the Kalgoorlie Sequence, part of the Black Flag Group. It comprises a carbonaceous greywacke that is similar to rocks found above the sill in the Black Flag Group. While many authurs suggest base of the Kalgoorlie Sequence is unconformable (e.g., Blewett et al., 2010), Gauthier et al. (2004) note that deep water carbonaceous clastic sedimentary rocks occur as interflow inter-beds within the upper sections of the Paringa Basalt and associated sub-volcanic sills. These interflow beds are increasingly abundant and thicker towards the top of the Paringa Basalt reflecting the waning stages of mafic volcanism. Consequently they suggest this contact between the Black Flag Group and the underlying Paringa Basalt, at the Golden Mile deposit, is transitional rather than an unconformity (e.g., Krapez et al., 2001). East of the Golden Mile deposit, where the Golden Mile Dolerite sill is absent, the basal mudstone unit of the Black Flag Group that lies above the Paringa Basalt is conformably overlain by a >200 m thick, fine to medium grained greywacke, possibly of volcaniclastic origin (Gauthier et al., 2005).
• Golden Mile Dolerite Sill, which is 600 to 750 m thick and has been dated at 2680±9 Ma (Zircon; Rasmussen et al., 2009) and 2673±5 Ma (Zircon; Claoué-Long et al., 1988). It is a stratabound intrusive unit predominantly found along the contact between the Paringa Basalt and the unconformably overlying Black Flag Group, and is interpreted to have been emplaced at a depth of >5 km below surface. While some authors suggest it is a volcanic unit of ponded lavas (e.g., Golding, 1985), Hayman et al. (2019) present evidence that it is intrusive. It is a fractionated sill that is divided into 10 lithologically distinct units with gradational boundaries that may be traced for >10 km along strike in the immediate deposit area and for ~25 km overall. The sill has fine grained, chilled margins that are 10 to 20 m thick and have a tholeiitic basalt composition, representing units 1 and 10 respectively. Between these margins there is a pattern of crystallisation progressing from the floor through unit 2 to 7, and from Unit 9 in the roof, towards the more fractionated granophyric Unit 8 occurring towards the centre of the intrusion. It represents a pattern of iron and incompatible element enrichment, including silica, as the magma becomes progressively more fractionated, as follows:
- Units 2 and 3 are orthopyroxene-clinopyroxene-plagioclase cumulates characterised by high MgO, Ni (50 to 150 ppm) and Cr (200 to 400 ppm). Unit 2 contains olivine, whilst Unit 3 is dominantly pyroxene bearing, although the two units have gradational boundaries. Together they are 70 to 100 m thick.
- Unit 4 is ~90 m thick and is a pyroxene-phyric band with distinctive aggregates of ilmenite, and is dominated by pyroxenes and plagioclase. It is characterised by flat geochemical profiles with low TiO2, zirconium and vanadium contents. Chrome and nickel become more enriched towards the base of the unit. There is a gradational upper boundary between units 4 and 5.
- Units 5 and 6 both of which are <100 m thick and are sub-ophitic, composed of a plagioclase-orthopyroxene-clinopyroxene assemblage with the appearance of minor primary quartz. However, unit 6 marks the onset of magnetite crystallisation, characterised by 10 to 15% fine-grained magnetite, and has a sharp contact with Unit 5. While Unit 6 is fine grained and sub-ophitic, it grades upward to be weakly granophyric in texture. It is also characterised by a very high V (600 to 1200 ppm) and enriched Cu (200 to 300 ppm compared to 20 to 50 ppm in unit 5) contents. Ni and Cr typically have a small peak at the base of this unit but decrease to very low levels towards the top of the unit, each grading from 50 to 5 ppm. Conversely, Ti and Titanium are gradually enriched towards the top of Unit 6. These geochemical levels are independent of proximity to mineralisation.
- Unit 7 is 100 to 200 m thick, and like units 5 and 6, is characterised by a sub-ophitic plagioclase-orthopyroxene-clinopyroxene assemblage with minor free quartz, although it contains coarse pegmatitic bands, generally a few metres in thickness, that are compositionally similar to unit 8. It is characterised by abundant magnetite, a decreasing V trend and increasing Zr and Ti values towards the top, reflected by the presence of abundant fine grained ilmenite. Ni, Cr and Cu are very low.
- Unit 8 is 100 to 200 m thick, and composed of a granophyric textured clinopyroxene-plagioclase-quartz-ilmenite-magnetite assemblage. It contains 10 to 15 wt.% magnetite, and is characterised by a high content of incompatible elements such as Zr, P and Ti, with low, V, Cr, Ni and Cu. It has been subdivided into two further sub-units, with Unit 8a at the top, which is more areally restricted and is more siliceous, with lesser Fe and Ti than than the underlying U8b. Unit 8a is found sporadically along the western limb of the Kalgoorlie syncline and is the host of the Mount Charlotte quartz-vein stockwork mineralisation. It also hosts several other smaller quartz-stockwork style occurrences along a roughly 12 km strike length of that limb, but has not been mapped on the eastern limb (Travis et al., 1971).
- Unit 9 is 100 to 350 m thick, and comprises a plagioclase-orthopyroxene-clinopyroxene-quartz assemblage with abundant fine grained magnetite and medium to coarse grained ilmenite. It is medium to coarse grained, and locally granophyric where it grades into U8, but above that is mostly sub-ophitic in texture. There is a gradual enrichment in Zr, Ti and V downwards toward Unit 8. It has relatively elevated Cr and Ni at the top towards the upper chilled margin, and a gradual depletion towards the base of the unit 9. It has consistently higher V, Zr and Ti contents compared to units 4 and 5, with a increase in V towards the Unit 8 contact.
The Aberdare Dolerite sill mapped at the northern, eastern nargin and southern end of the Gold Field, has been shown to be equivalent to the Golden Mile Dolerite, with a gradual attenuation of the fractionation and resulting magmatic layering of the latter into the former. The two 'facies' are juxtaposed across the late Adelaide Fault to the south, but have a gradational transition to the north (Gauthier et al., 2005).
• Black Flag Group, a >3000 m thick, 2690 and 2660 Ma succession that comprises cycles of volcanic, volcaniclastic and sedimentary rocks and mostly represents rapidly reworked pyroclastic debris. In the Golden Mile deposit area, the base of the Black Flag Group comprises black
mudstone inter-bedded with siltstone and sandstone beds, similar to that of the Oroya Shale described above (Gauthier et al., 2005).
The Black Flag Group is divided into the Early Black Flag Group, which consists of massive graded to moderately stratified feldspar-rich sandstone, siltstone, felsic cobble-conglomerate, volcanic rocks and associated polymict volcaniclastic and epiclastic rocks, and rare mudstone. The volcanic rocks are mostly tonalite-trondhjemite-dacite with subordinate rhyolite and andesite. Rapidly reworked feldspar-rich pyroclastic debris predominates towards the base and intermediate to felsic volcanic and volcaniclastic rocks in the upper sections. The Late Black Flag Group unconformably overlies the Early Black Flag Group and the Golden Mile Dolerite sill. It consists of coarse mafic conglomerate facies, quartzo-feldspathic sandstone, with interbedded volcanic rocks as well as mudstone-siltstone (Oxenburgh et al., 2017; Squire et al., 2010), and includes clasts sourced from the sill in its basal sections. Two additional, similar cycles are mapped, which are variously attributed to the Black Flag Group (e.g., Vielreicher et al., 2016) or the overlying lower and late Merougil Group (e.g., Squire et al., 2010).
• Intrusions - the Kambalda and Kalgoorlie sequences are intruded by a series of dykes and sills related to the Kalgoorlie Sequence volcanism (after Gauthier et al., 2005; Vielreicher et al., 2016):
- Syn-volcanic, tholeiitic dykes - which are fine grained, chloritic and commonly contain vesicular chilled margins. They occur within the lower black shale portion of the Black Flag Group and commonly have very irregular and peperitic margins. They are commonly strongly sericite + ankerite altered
within the Golden Mile deposit and are cross cut by the feldspar porphyry dykes.
- Quartz-albite porphyry dykes and sills - A suite of syn-volcanic, structurally early, pre-gold, calc-alkaline plagioclase-phyric rhyolite and dacite sills and dykes dated at between 2673±3 and 2669±17 Ma, regarded as feeder dykes to volcanic units in the Kalgoorlie Sequence.
- Hornblende and feldspar porphyry dykes - occurring as local NNE striking and ~74° SE dipping, syn-deformational dyke swarms. These intrusions are a variably metamorphosed, ~2.65 Ga fractionated suite of sub-alkaline to alkaline hornblende-phyric andesite to feldspar-phyric porphyry dykes with Mg-monzodiorite-diorite to granodiorite-tonalite compositions respectively. These are both cut, and are cut by, Fimiston-style lodes and are cut by Charlotte-style lodes (see Mineralisation section below).
The feldspar porphyry dykes contain 10 to 40%, 2 to 10 mm plagioclase phenocrysts and 1 to 10% small rounded quartz phenocrysts within a fine grained quartzo-feldspathic groundmass. They have been dated at 2650±6 Ma (zircon; U-Pb SHRIMP; Vielreicher et al., 2010), although the same author summarises the feldspar-phyric porphyries as being emplace between 2.67 to 2.66 Ga. These dykes also contain trace to 10% hornblende phenocrysts. Feldspar porphyry dykes within the Golden Mile deposit are pervasively sericite + carbonate altered and variably hematitic. Two main large feldspar porphyry dykes occur within the Eastern part of the Golden Mile deposit where they are continuous along strike for at least 2 km, down dip for >1.5 km, and vary in thickness from 5 to 20 m (Gauthier et al., 2005).
A 100 to 400 m thick, composite, steeply west dipping feldspar porphyry dyke intrudes along the trace of the Kalgoorlie syncline to the south of the Golden Mile deposit and cuts the folded mafic volcanic rocks, Golden Mile Dolerite and overlying Black Flag Group sequence. It has wide zones of intrusive breccias, mainly along its margins, and contains several textures interpreted to indicate shallow intrusion (Morris and Witt, 1997; Ong, 1994).
The hornblende porphyry dykes are generally 1 to 5 m thick and are less abundant than feldspar porphyry dykes (Stillwell, 1929). They generally contain 10 to 40%, 2 to 10 mm hornblende phenocrysts aligned along the NW penetrative foliation planes. These dykes also contain 5 to10% plagioclase and 1 to 10% rounded quartz phenocrysts. Within the Golden Mile deposit, the are ubiquitously altered to an assemblage of carbonate and sericite. They have an alkaline affinity, as indicated by their enrichment in incompatible elements such as P2O5, Zr and Y and are interpreted to have been formed by a different magmatic event than the earlier calk-alkaline feldspar porphyry dykes (Gauthier et al., 2005). They have been dated at 2646±13 Ma (zircon; U-Pb SHRIMP; Vielreicher et al., 2010) who also quotes these dykes as being emplaced between 2.66 Ga and 2.65 Ga based on additional evidence..
- Intrusive breccia, which typically occur on the margin of large feldspar porphyry dykes, or as small individual breccias commonly found close to a larger coherent feldspar porphyry dyke. They cut all the host rocks present at the Golden Mile and typically grade from angular jig saw fit of feldspar porphyry clasts within the host rock matrix to more heterolithic and matrix supported breccias. Some exotic fragments within breccias have travelled up to 600 m away from their source. These breccias both cut and are cut by feldspar porphyry dykes, and are regarded to be intrusive, rather than tectonic.
- Late deformational mica lamprophyre and kersantite dykes dated at 2642±6 Ma (zircon; U-Pb SHRIMP; McNaughton et al., 2005) and 2637±20 Ma (monazite; U-Pb SHRIMP; McNaughton et al., 2005), and are both cut and are cut by Green Leader-style lodes and are cut by Charlotte-style lodes, but are not affected by Fimiston-stage alteration (see Mineralisation section below).
Late Clastic Basins - the Kambalda and Kalgoorlie sequences are unconformably overlain by the 2658 to 2655 Ma Kurrawang Formation sequence of polymictic conglomerate, grading upward into fine-grained sandstone and siltstone in extensional fault controlled clastic basins.
Structure
Deposition of the Kambalda and Kalgoorlie sequences took place during 2720 to 2670 Ma D1 extension. This was followed by inversion during the D2 ENE-WSW directed compressive event at ~2665 Ma producing NNW upright folding and reverse faulting, responsible for tilting of the host sequence, formation of the Kalgoorlie Syncline and Anticline, regional Boulder-Lefroy and deposit scale Golden Mile faults and a penetrative, subvertical foliation. This was followed by renewed extension during the 2665 to 2655 Ma D3 event, resulting in the development of a series of metamorphic core complexes exposing the main intrusive phase of high-Ca granites that were emplaced from 2740, continuing to 2650 Ma, and accounting for >60% of the granites in the terrane. The volumetrically lesser Low-Ca granitoids, which are reworked earlier High-Ca granites, were all formed between 2655 and 2630 Ma, subsequent to the main greenstone belt magmatism and the clastic basin deposition. Later deformation comprised D4a ENE-WSW contraction, resulting in upright folding and reverse faulting at ~2655 Ma; D4b WNW-ESE contraction, producing sinistral strike-slip shearing and thrusting from 2655 to 2650 Ma; D5 NE-SW contraction and dextral strike-slip transtension from 2650 to 2635 Ma; and D6 Low-strain vertical shortening and horizontal extension after 2630 Ma. The deformation stages D1 to D6 listed herein are as defined by Blewett and Czarnota (2007) in GeoScience Australia Record 2007/15, and resulted from the exhaustive Module 3 structural study of the pmd*CRC and AMIRA Y1-P763 Project that concluded in November 2005. A plethora of stages have been defined by other authors, including Mueller et al. (1988), Swager (1997), Nguyen (1997) and Miller (2006) and are used in current literature, such as Vielreicher et al. (2016) - see Blewett et al. (2010) for a comparison of the different stages defined.
Folding - The Kalgoorlie Gold Field follows the NNW to north-south trending, fault dislocated, upright, early D2 Kalgoorlie Anticline-Syncline pair. The Golden Mile deposit is located where the trend of the axial trace of the structural pair changes from NNW in the south, to north-south in the north. These structures have folded a core of Kambalda Sequence rocks that are intruded by the Golden Mile Dolerite sill and are surrounded by overlying and fault juxtaposed Black Flag Group rocks of the Kalgoorlie Sequence. This syncline-anticline pair dominates the structural architecture in the Golden Mile deposit area (Woodall 1965, Travis et al., 1971).
The Kalgoorlie Syncline is an asymmetric fold with an overturned western limb dipping at 80°W, whilst the eastern limb dips ~30°W in the Golden Mile deposit area. To the south of the deposit, where its strike rotates to trend NNW, the same limb changes dip to ~75°E. The axis of the Kalgoorlie Anticline, immediately to the east, is doubly plunging. In the Golden Mile deposit area, where the axial plane dips at ~80°W, the axis plunges at ~20°S, resulting in progressively older units being exposed along its axial trace towards the NNW. To the north of the deposit, the fold plunges to the north. In the immediate Golden Mile deposit area and northward, the hinge of the Kalgoorlie syncline is offset by the Golden Mile fault (Woodall, 1965), but is preserved ~2 km south of the deposit (Travis et al., 1971; Gauthier et al., 2005).
Smaller scale parasitic folds are developed on the western limb of the Kalgoorlie Anticline, spatially associated with the bend in the axial trace of the first order Kalgoorlie Syncline-Anticline fold pair and on the basis of structural fabric can be inferred to be coeval with formation of the main Kalgoorlie Syncline-Anticline.
A fault coincides with the axial plane of the Kalgoorlie Anticline and with the eastern edge of the Golden Mile Dolerite. This structure is interpreted to be a reactivated normal growth fault active during the emplacement of the intrusion. This conclusion is supporter by the absence of that intrusion on the eastern limb of the Kalgoorlie Anticline, and the distribution and thickness of its constituent units towards the axial plane.
The regional scale Boomerang Anticline is ~10 km NNW of the Golden Mile deposit, and refolds both the Kalgoorlie Syncline-Anticline pair and the Golden Mile Fault. Its axial trace is parallel to the steeply west dipping penetrative northwest foliation which overprints the earlier folds at the Golden Mile.
Early Faulting - Most of the folds described above are variously displaced by the crustal-scale, NNW trending, D2, sinistral Boulder-Lefroy Fault which has an offset of ~12 km and occurs along the western margin of the gold field. Displacement on this and relate D2 faults is also in part due to reactivation during subsequent compressional events. The Golden Mile deposit is located at a major change in the strike of this fault, corresponding to the similar bend in the axes of the Kalgoorlie Anticline-Syncline pair as described above. The regional scale sub-vertical Boulder-Lefroy Fault is oriented NNW south of the deposit and NW to its north, before returning to a NNW strike further to the north again. The parallel subsidiary D2 Golden Mile Shear/Fault passess through the deposits as do related lode bearing D2 shears and splays. These structures are dislocated by D3 extensional faults and D4 and D5 dextral shears, which combine to form a complex pattern of NNW, NNE north-south and NE faults (Vielreicher et al., 2016).
In the Golden Mile deposit area and further to the NNW, the D2 Golden Mile Fault dips steeply to the west, and has a substantial normal displacement, offsetting the hinge of the Kalgoorlie Syncline in the immediate Golden Mile deposit area (Woodall 1965). In the deposit area, it forms the eastern contact between a sliver of overturned, 85°W dipping, finely bedded, mudstones and sandstones of the Black Flag Group, and the Golden Mile Dolerite. The former is on the western limb of the Kalgoorlie Anticline, the latter dips at 30°W and is on the juxtaposed western limb of the Kalgoorlie Anticline. To the west, further Golden Mile Dolerite concordantly underlies the Black Flag Group sliver. This sliver of Back Flag Group sedimentary rocks represents the remnant core of the Kalgoorlie Syncline. It is a weak lithological layer, wedged between two competent blocks of Golden Mile Dolerite, and has been the focus of multiple pulses of displacement and shearing associated with this structure (Clout, 1989). This sedimentary sliver is also discordantly intruded by numerous steeply west dipping feldspar porphyry dykes (as described above) sub-parallel to the trace of the Golden Mile Fault (Stillwell, 1929). These dykes have a similar orientation to the feldspar porphyry dykes that cut other lithological units throughout the deposit (Gauthier et al., 2005).
Several sets of steeply east and west dipping reverse faults occur within the Black Flag sediment wedge and extend into underlying basalts and dolerites. These include an axial planar fault in the core of the Kalgoorlie Anticline. One such late fault within this set of structures (described as an example) extends over a strike length of ~2 km near the centre of the Black Flag Group wedge within the Golden Mile deposit. It is a 0.3 to 1 m thick zone of graphitic fault gouge with fabrics that demonstrates a dextral sense of movement. The fault strikes at 320° and dips 85°W, with stretching lineations that are consistently sub-horizontal. It contains completely dislocated and boudinaged quartz-carbonate veins, suggesting a complex history of repeated movement and later reactivation (Robert and Poulsen, 2001).
In the NE of the Golden Mile deposit, a set of steeply east-dipping reverse faults with offsets of several hundred metres merge into the Golden Mile Fault. Both these reverse faults and the Golden Mile Fault systematically truncate the Fimiston lodes with a dextral sense of displacement. Drilling and detailed mapping show that the trace of the Kalgoorlie Anticline axial plane is not parallel to the Golden Mile Fault, with dips of 80°W and 85°W respectively, whilst the anticline axis plunges at 20°S. As the two structures diverge to the north and south of the deposit, the closure of the Kalgoorlie Syncline reappears. In addition, detailed field observations in the Golden Mile demonstrate that the Golden Mile Fault offsets the upright Kalgoorlie Syncline and that it postdates upright folding and tilting of the host sequence (e.g., Gauthier et al., 2005). These conclusions are not consistent with previous interpretations that faulting predates tilting and that the Kalgoorlie anticline represents an overturned thrust ramp (Clout, 1990).
Shearing - A network of NW to NNW trending shear zones, predominantly steeply NE dipping and NE block up irrespective of dip, offset the stratigraphy, porphyry dykes and Fimiston lodes. Displacement is predominantly reverse, with only a minor, late, dextral strike-slip component. Most result in similar offset on the stratigraphy, lodes and dykes, although those closer to the Kalgoorlie Fault display a significantly larger component of displacement of the stratigraphy than the lodes and dykes. These shears are concentrated in the NW section of the Eastern Lode Domain, and ubiquitously cross cut Fimiston lodes. Most are narrow zones characterised by several cm-scale shear planes over a 1 to 2 m width. There are at least 5 main shear zones, which are part of complex array that includes many smaller structures with lesser displacement. Those shear zones closest to the Golden Mile Fault have the most extensive displacement, with the two main structures (the 'A' and 'C' shears) together accounting for 425 m of reverse movement and 175 m of dextral offset. They are sub-vertical to steeply west dipping at their NW end, whilst those to the NE, further from the Golden Mile Fault, have lesser displacement and are steeply NE dipping. The latter include the Kalgoorlie Shear, one of the most extensive, with a 175 m reverse offset on the stratigraphy, feldspar porphyry dykes and Fimiston lodes and <10 m of dextral strike-slip. The similar Australia East shear zone also distal to the Golden Mile Fault has a a reverse displacement of ~120 m and a 10 to 20 m sinistral offset (Gauthier et al., 2005).
These steep shears are overprinted by a younger conjugate network of shallow dipping, reverse shear zones that are ubiquitous throughout
the Golden Mile. They generally dip at 30 to 45° to either the east or west, the latter set being dominant (Ridley and Mengler, 2000). The reverse displacement on these shears is generally around a few metres at most. They commonly contain quartz veins and quartz breccias where cross cutting competent units (Ridley and Mengler, 2000). At Mt. Charlotte, the late steep north-south to NNE dextral strike slip faults cross cut the set of shallow dipping reverse shears (Ridley and Mengler, 2000), although the latter share mutually cross cutting relationship with the Mt. Charlotte quartz-carbonate vein stockwork (Clout et al., 1990, Ridley and Mengler, 2000).
Late Strike-Slip Faulting, which trend north-south to NNE and are sub-vertical, forming a regionally significant set. They represent the latest set on the Golden Mile, post-dating the Fimiston lodes and crosscut the early faulting. One of these, the Hannans Star Fault, dextrally offsets the Morrisson lode, part of the Western Lode System, by ~40 m. The Hannans Star Fault also offsets the steeply east dipping reverse Adelaide Fault, a late regional-scale, NNW trending, steeply west dipping dextral fault which is reflected by ~1 m of sericitic and carbonate-rich fault gauge. The Australia East Fault, a steeply east
dipping reverse fault which offsets the Fimiston Lodes, is itself cross cut and offset by the Adelaide Fault, establishing the latter as a late structure. Displacement on this structure varies from an ~300 m normal, to a dominantly dextral offset in the SE. The late NNW, ~60 to 70°W dipping Golden Pike Fault dextrally offsets the Boulder-Lefroy and Golden Mile faults by ~500 m, but the stratigraphy by ~2 km (Gauthier et al., 2005). These late faults are interpreted to have influenced the structural fabric that controlled the deposition of mineralisation. The dextral D5 Adelaide/Hannans Star and Golden Pike faults which are obliquely displace the main structural trend of the Golden Mile deposit were accompanied by reactivation of the earlier major structures that define that trend during gold mineralisation (Vielreicher et al., 2016; Groves et al., 2018). Similarly, as detailed below, the D5 dextral, obliquely cross cutting Charlotte and Maritana faults straddle the Mount Charlotte deposit with a similar reactivation of the Golden Mile and related D2 structures.
Mineralisation
Gold mineralisation within the Kalgoorlie Gold Field is hosted in all rock types within the gold field. However, 70 to 80% of production comes from mineralisation hosted by the 2680±9 Ma Golden Mile Dolerite.
The Kalgoorlie Gold Field is characterised by two dominant ore types, the Fimiston- and Mount Charlotte-styles, largely corresponding to the two mining areas (Vielreicher et al., 2016).
Fimiston-style lodes
Fimiston-style lodes within the Golden Mile deposit can be subdivided into the Eastern Lode Domain, a swarm of smaller lodes segmented by numerous steep reverse faults, and the Western Lode Domain which is less complex, with good lateral and vertical continuity, and are better defined. These domains are separated by the Golden Mile Fault and band of intensely deformed Black Flag Group rocks and basically coincide with the eastern and western limbs of the Kalgoorlie Syncline (Gauthier et al., 2005).
Fimiston-style lodes are predominantly zones of replacement characterised by brecciation and fracture fill, and comprises a complex array of ductile to brittle stringer-, vein- and breccia-lodes that evolved broadly during the syn- to late-formation of the regional NW-trending foliation. They are developed in four principal directions in both the Eastern and Western Lode Domains (Finucane, 1948) with strike and dip orientations as follows: i). Main 135 to 140°/90 to 85°SW, parallel to the main NW Golden Mile Fault; ii). Caunter at 100 to 120°/55 to 80°SSW; iii). Cross Lodes at 40 to 50°/65 to 80°SE; and iv). Easterly at 160°/70 to 90°ENE. The Main and Caunter lodes are the dominant sets in both domains. Individual lodes are generally narrow, <2 m thick, but are vertically and laterally extensive, up to 2 km long by 1.3 km in vertical extent. The vertical continuity of mineralisation has been traced to a depth of at least 1.8 km. Together, the lodes in both domains form a downward tapering array, which is sub-vertical in the Western and steeply west dipping in the Eastern lode domains (Gauthier et al., 2005).
The approximately planar, steeply dipping Fimiston-style lodes are characterised by pyrite veinlets and disseminations, fine-grained quartz-sericite-sulphide-telluride bearing quartz-carbonate veinlets, crackle- and cockade-breccias, banded chalcedonic quartz-carbonate veins, and stringer zones that grade into lenses of microbreccia and cataclasite (Vielreicher et al., 2016; after Clout, 1989; Bateman et al., 2001; Gauthier et al., 2004). Lodes are characterised by variably foliated quartz - sericite - pyrite - ankerite/dolomite/siderite/calcite - Fe/Ti-oxides (locally as boxworks after Ti-magnetite), ±Fe-chlorite, ±tourmaline alteration. This sericite, carbonate and pyrite-dominated alteration forms i). an inner proximal gold-bearing, pyrite-sericite zone with ankerite ±siderite, surrounded by ii). an ankerite and siderite zone that extends for over 100 m outwards, and is enveloped by iii). an outer 1 km wide chlorite-calcite ±ankerite assemblage. The distribution of this alteration is strongly influenced by both structure and lithology (Gauthier et al., 2005). There is a strong lithological control on alteration (Nixon et al., 2014), and as a consequence, the intensity and mineralogy of alteration is strongly controlled by the interplay of structure and lithology (Phillips, 1986; White et al., 2003).
The wide distal carbonate ±chlorite alteration zone appears to be the result of fluid flow along multiple thinner conduits in the inner pyrite-rich zones that were each accompanied by their own discrete alteration selvedges. As fluid flow continued and additional fractures opened, the selvedges expanded and coalesced to form a broad, composite, virtually continuous alteration halo (White et al., 2003).
The mineralogy of this alteration assemblage would be consistent with influx of an aqueous-CO2 dominated fluid reacting with the metamafic host rocks to cause breakdown of actinolite to chlorite and carbonate (e.g., Vielreicher et al., 2016).
Sericite in the proximal alteration zones is regarded to be the result of potassium addition from the fluid (White et al., 2003), and pyrite is the result of sulphidation of Fe in the host rocks (Neall, 1987). Mineral equilibria studies show the alteration assemblages are consistent with equilibrium with a single fluid of composition XCO2 = CO2/(CO2 + H2O) = 0.1 to 0.25 (outer zones) and 0.25 (inner zones) at temperatures of 320 to 315°C (White et al., 2003). These temperatures are within the range of from 390 to 305°C defined by chlorite and arsenopyrite geothermometry of alteration assemblages collected from the Oroya, Lake View and Great Boulder Main Lodes on the Golden Mile (Vielreicher et al., 2016). Sericite geobarometry on the same samples suggest pressures of 110 to 290 MPa (Vielreicher et al., 2016). In addition, Shackleton et al. (2003) interpreted data to suggest gold + calaverite + petzite and minor late hessite in the Golden Mile were deposited by a fluid cooling from 300 to below 170°C.
Gold within the Fimiston lodes is present in either inclusion-poor auriferous pyrite, or as micron-scale inclusions in <100 µm diameter inclusion-rich pyrite. As such, the gold is typically refractory, with gold grades directly related to the occurrence of fine-grained pyrite.
High grade zones, typically carrying >50 g/t, but locally up to 1% Au, contain native gold, tellurium in a range of telluride minerals (Shackleton et al., 2003), as well as closely associated chalcopyrite, arsenopyrite, tennantite-tetrahedrite, sphalerite and galena. These zones are characterised by the addition of CO2, K, Rb, S as well as Au (-B), Te, V and Ba (Phillips, 1986). Locally, bonanza grade shoots, known as 'Green-Leader' (Larcombe, 1912) or Oroya-style lodes with grades of up to 10% Au are spatially associated with intersections of the lodes with carbonaceous metasedimentary units. Gold in has been observed in syn-sedimentary to diagenetic zoned pyrite nodules within these graphitic units (Steadman et al., 2015). The Oroya-style lodes are characterised by abundant free gold with associated native tellurium and rarely, mercury (Weller et al., 1998), along with disseminated pyrite and numerous Au-Ag, Ni, Hg, Pb, Sb-bearing telluride minerals (Stillwell, 1929; Scantlebury, 1983; Clout et al., 1990). They occur within roscoelite-bearing (green vanadium-bearing muscovite) alteration zones with ankerite, calcite, siderite, sericite, V-Ti bearing Fe-oxides, pyrite, chalcopyrite, arsenopyrite, tennantite-tetrahedrite, sphalerite and galena (Stillwell, 1929; Scantlebury, 1983). The principal example is the 1500 m long Oroya shoot, controlled in large part by the 50°W dipping, reverse Oroya shear zone system. This style of ore occurs in the upper extremities and brecciated cores of steeply dipping Fimiston lodes, and may represent a late-stage of the D4b transpressional regime that generated the Fimiston lodes (Mueller et al., 1988) and a separate mineralisation sub-stage (Bateman et al., 2001) as described below.
Gold within the Fimiston lodes is present in either inclusion-poor auriferous pyrite, or as micron-scale inclusions in <100 µm diameter inclusion-rich pyrite. As such, the gold is typically refractory, with gold grades directly related to the occurrence of fine-grained pyrite.
Zoning is evident within the Golden Mile, with an overall increase in Sb/Au in the tennantite-tetrahedrite group minerals, as well as an increase in the Au:Ag ratio in the free gold with depth (Golding, 1978). This zonation is also associated with variations in the telluride mineralogy, with increasing montbrayite ((AuSb)2Te) laterally, and telluroantimony (Sb2Te3) at depth (Shackleton et al., 2003).
In the Western Lode Domain, Fimiston lodes form a sub-vertical network 1.6 km in strike length and up to 1.1 km in vertical extent. They mostly occur in the steeply west dipping Unit 9 of the Golden Mile Dolerite, close to, but bounded by the Black Flag Group to the east and by Unit 8 to the west. The core of the lode system in this domain is spatially associated with a bend in the Golden Mile Fault, from a strike of 135° in the north to 120° in the south and from sub-vertical near surface, to 80°W dipping at depth. This bend, or jog, corresponds to the similar bends in the axes of the Kalgoorlie Syncline-Anticline pair and in the Lefroy-Boulder Fault as described previously. The lodes within this domain are vertically zoned, e.g., the Lode #4, where the gold decreases markedly from the bonanza grades over the upper 400 m to subeconomic values below the 1100 m level (Larcombe, 1912; Clout, 1989).
The lodes of the Eastern Lode Domain form a steeply west dipping network that extends over a strike length of ~3 km associated with a corridor of alteration and gold mineralisation that persists for a further 10 km to the NNW where it encompasses several smaller gold deposits, including Mt Percy. The lode system within this domain has a pronounced narrowing downwards, which is controlled by the shallow west dip of the Golden Mile Dolerite. It thins from widths of 500 to 600 m within the Golden Mile Dolerite, to 200 to 300 m wide in the underlying Paringa Basalt. The lodes are steeply west dipping, with economic grades generally restricted to the Golden Mile Dolerite, rarely persisting for more than 50 to 100 m below in the Paringa Basalt (Woodall, 1965). Unlike in the Western Lode Domain, the Eastern Domain lodes are strongly discordant to the shallow dipping stratigraphy and stratigraphic contacts have a strong influence on the location of high grade ore shoots within lodes (Gauthier et al., 2005).
Examples from within selected Fimiston Lodes at Kalgoorlie - Note the different orientations and generations of veining in the upper image; The second image pair is of breccia lode, showing ~20 cm of drill core from a broader >6 g/t Au interval, and an enlarged central section of that interval (note the disseminated sulphides); The lower image pair is of sheared lode and an enlargement of section of the same (again, note the disseminated and fracture controlled sulphides). Images of KCGM drill core by Mike Porter., 2019.
Paragenesis. The Fimiston lodes and their associated alteration reflect a complex evolving system of paragenetic stages with close spatial and temporal associations that tend to overlap and form complex mutually cross-cutting relationships (Clout, 1989). The main paragenetic stages comprise:
• Stage 1 - Early iron-carbonate +magnetite +hematite +quartz veins and breccias which form widespread carbonate-rich breccias and veins without significant gold grades, and pre-dates the main gold bearing stages. Carbonate breccias and veins are best developed within the more competent granophyric Unit 8 and Unit 7 of the Golden Mile Dolerite and have a clear spatial association with the later stage mineralised Fimiston lodes which they clearly pre-date. The breccia, that exhibit a jigsaw pattern, have a matrix that ranges from iron-carbonate in wide zones of hydrothermal brecciation to narrower zones of ankerite +quartz +magnetite +hematite breccias and veins with locally well-developed infill textures. Magnetite varies widely, with local massive bands up to a few cm thick, and can grade locally into black hematite-rich breccias with the same textural characteristics and temporal relationships. Magnetite and quartz +magnetite veins and associated magmatic breccias have mutually cross cutting relationships with feldspar porphyry, dykes suggesting contemporaneous development.
• Stage 2 - Finely disseminated pyrite +quartz +sericite +carbonate +tourmaline. Pyrite generally occurs as broad zones of 10 to 20 wt.%, but locally up to 50 wt.% disseminations and/or narrow veinlet stockworks. Pyrite is also commonly concentrated in pillow rims and flow breccia matrix within the Paringa Basalt. Pyrite disseminations dominate within the Paringa Basalt and to a lesser extent within Unit 8 of the Golden Mile Dolerite where they form relatively broad zones in association with Fimiston lodes. They typically contain from 1 to 10 g/t Au, although local high gold grades are associated with pyrite +tourmaline veinlets. This mineralisation is characterised by a high Ag:Au ratios (commonly >1)in contrasts to the last following paragenetic stages, which have low Ag:Au ratios. Tourmaline is commonly associated with the pyrite-rich veins and dissemination zones. Pyrite dissemination zones generally grade laterally into sericite +iron carbonate alteration with diminished levels of pyrite (1 to 5 wt.%), and the appearance of magnetite. Although the margins of these pyrite zones have a similar mineralogy to the selvages of later stage veining they can be demonstrated to be due to separate events (Gauthier et al., 2005).
• Stage 2a - Green leader style disseminated pyrite +gold +tellurides within roscoelite-rich alteration zones. Tourmaline also commonly accompanies this sub-stage. It represents a sub-set of stage 2 (Clout, 1989) and forms bonanza ore shoots e.g., the Oroya lode which yielded >65 tonnes of gold at an average grade of 31 g/t Au; and the Duck Pond ore shoot that averaged 1245 g/t Au. These high grade shoots form within the upper extremities of the typical Fimiston type lodes (Clout, 1989). Both the Oroya and Duck Pond shoots occur as flat lying cigar-shaped lenses, controlled by the intersection between a steeply west dipping Fimiston lode and shallow dipping lithological contacts. Duck Pond lies within the large Lake View lode, and is associated with an infold of black shales of the Black Flag Beds in the Lake View syncline, although not formed directly in contact with the black shales (Clout, 1989; Gauthier et al., 2005). The gently-plunging pipe-like Oroya Shoot lies within the Paringa Basalt and follows a graphitic sedimentary unit, the Oroya Shale, at the contact with the Golden Mile Dolerite (Bateman et al., 2001).
• Stage 3 - Quartz ±carbonate veinlets and breccias, which comprises arrays of generally 5 to 20 mm thick quartz veinlet stockworks within Fimiston lodes. The veinlets locally grade into thicker breccias, commonly containing mineralised wall rock clasts. The veinlets are rimmed by narrow pyrite +sericite +iron carbonate alteration selvedges grading outward into sericite +iron carbonate. These veinlets and breccias host high grad gold with associated tellurides, and generally represent the high grade portion of the lodes. The quartz veinlets contain well-developed comb textures and quartz breccias display a range of infill textures. Whilst the veinlets are dominated by quartz, they also contain carbonate as discreet bands within the veins and breccias. Anhydrite is also a locally significant infill (Clout, 1989). The quartz veinlets clearly cross cut pyrite-rich zones of stages 2 and 2a.
• Stage 4 - Banded quartz-carbonate veins, which range from 2 to 10 cm in thickness, and are predominantly aligned at angles of 238°/65°NW and 22°/59°SE, close to the Cross Lode and Caunter trends (108°/77°NW). These veins cross cut both Stage 2 and 3 veins and are composed of finely alternating bands of quartz and carbonates with pyrite locally concentrated along individual bands. They have variably developed selvedges of pyrite +sericite +carbonates. There is a correlation between gold grades and the pyrite content of the veins. Gold grades are highly variable, with these veins commonly being barren at the periphery of the deposit.
• Post Fimiston hydrothermal events, which postdate the NW foliation and cross cut the Fimiston lodes. These include:
- Sub-vertical barren coarse-grained carbonate veins that are ubiquitous throughout the Golden Mile deposit area and are also a widely distributed regional feature. They veins are associated with the steep reverse faults and cut Fimiston Lodes.
- Mount Charlotte-style lodes which are described below. In the Golden Mile deposit these veins are mostly within the most competent Unit 8 of the Golden Mile Dolerite and also within feldspar porphyry dykes. Two concentrations of these veins form stockworks that are dominantly oriented north-south, and dip steeply west and sub-horizontal to shallow west. They occur along the late Golden Pike fault within Unit 8 in the Western Lode Domain, where they form the Golden Pike and Drysdale stockworks (Clout et al., 1990). See details of the Golden Pike fault above in the Structure section.
Age of Mineralisation - Fimiston-style gold mineralisation is constrained to ~2.64 Ga, during (late D4b? to) D5. It post-dates the 2.69 Ga Paringa Basalt, 2.68 Ga Golden Mile Dolerite and deposition of the 2.69 to 2.66 Ga Black Flag Group. A 20 m thick pre-mineral feldspar porphyry dyke that is clearly cross cut by the Australia East Lode of the Golden Mile deposit has been dated at 2676 ±3 Ma (zircon; U-Pb TIMS; Gauthier et al., 2005). Other similar dykes have yielded comparable ages and relationships to mineralisation. Alkaline hornblende porphyry dyke, dated at 2663 ±11 Ma (zircon; U-Pb TIMS; Gauthier et al., 2005) clearly cross cuts a Fimiston stage banded quartz-carbonate vein of the Golden Mile deposit. However, these dykes, which occupy similar structural sites to the gold mineralisation, are ubiquitously altered to an assemblage of carbonate and sericite within the Golden Mile deposit, implying they may be intra-mineral. An unaltered lamprophyre dyke cross cutting the Oroya lode was dated at 2638 ±6 Ma and dissects the Oroya lode (McNaughton et al. 2001). This dyke is described as unaltered except for a thin crust along its margin and contains no significant K2O (K2O = 0.15 wt.%). This is significant, as the Oroya lode is characterised by intense roscoelite alteration and substantial associated K2O enrichment (Clout, 1989).
Petrographic studies (Mikucki and Roberts, 2003) indicate gold mineralisation and wall rock alteration were just post-peak regional greenschist-facies metamorphism, which is considered to have occurred prior to ~2.65 Ga (Vielreicher et al., 2016).
Mineralisation-related, hydrothermal monazite and xenotime in ore samples from the Mt Charlotte deposit, which is interpreted to be structurally late, gave ages of 2655±13 Ma (Rasmussen et al., 2009) and 2644±11 Ma (Vielreicher et al., 2010). The most precise data from the structurally older Fimiston-style ore samples yielded an age of 2632±12 Ma (Vielreicher et al., 2010), with a minimum age of 2636 Ma for Oroya-mineralization (McNaughton et al., 2005). Published 40Ar/39Ar dates for hydrothermal, gold-related sericite from the Golden Mile (recalculated from Kent and McDougall, 1995 by Vielreicher et al., 2010) of 2641±13 Ma and 2643±13 Ma overlap all these data. From this, Vielreicher et al. (2016), concluded that as geological evidence indicates Mt Charlotte ore formed after the Fimiston ore, both ore types must have formed within 10 m.y. of each other at ~2.64 Ga.
Mount Charlotte-style lodes
Mt Charlotte type Quartz-carbonate veins are the dominant lode type in the Mount Charlotte mine, but also occur at Mount Percy and in the Golden Mile deposit (e.g. the 'Golden Pike and Drysdale stockwork lode') mostly within the most competent Unit 8 of the Golden Mile Dolerite and also within feldspar porphyry dykes (Gauthier et al., 2005).
Conversely, Fimiston-style lodes are also represented at Mount Charlotte where Mount Charlotte-style quartz vein stockworks are separated from Fimiston-Style mineralisation and alteration across barren D4b thrusts (Mueller, 2015). These lodes are more siliceous and silver-rich than those in the Golden Mile deposit and commonly contain white laminated quartz veins and grey silica-pyrite bands, and economic gold-pyrite ±telluride mineralisation is typically developed over 0.5 to 2 m widths. Similarly, Fimiston-style mineralisation is redeveloped at Hannan's North to the north of Mount Charlotte, where the lode lacks the late telluride stage of the Golden Mile. Sericite-ankerite alteration is discontinuous, and siliceous lodes are locally in direct contact with chloritic Golden Mile Dolerite (Haycraft, 1965).
Mount Charlotte-style lodes are mostly brittle, and slightly younger than the Fimiston lodes. They comprise elongate pipe-shaped networks of dilational vein-stockworks of sheeted north-dipping veins occupying extensional and shear fractures (Mueller, 2017).
The vein network that makes up the Charlotte orebody, which accounts for ~70% of the ore mined at Mount Charlotte, covers a plan area of 250 x 75 m, and vertical extent of >1000 m. The orebody strikes NW-SE, parallel to the Golden Mile Dolerite and D2 Golden Mile Fault, and is terminated and offset by the transtensional D5 dextral, strike-slip, north-south striking Charlotte, Reward and Maritana faults and related intervening structures (Mueller, 2017).
These two major structural elements control the location of Mt Charlotte-style gold mineralisation within the Mt Charlotte deposit, namely the 2625 Ma, dextral, strike-slip D5 faults, and reactivated west-dipping D2 reverse faulting. The deposit occurred where these structures were both active within competent igneous rocks that provided a lithological control (Bateman and Hagemann, 2004). These include, in particular, the most differentiated coarse-grained and competent granophyric clinopyroxene-plagioclase-quartz-ilmenite-magnetite Unit 8a of the Golden Mile Dolerite, but also within Units 7, lower 8 and 9, and porphyry dykes intruding the Hannans Lake Serpentinite. The Charlotte deposit area includes swarms of ~2.67 Ga feldspar-phyric porphyry, lesser ~2.66 to 2.65 Ga calc-alkaline hornblende-phyric and ~2.65 to 2.64 Ga lamprophyre dykes (Vielreicher et al., 2016).
The D5 faults crosscut and offset the D2 faults, although later oblique reactivation of the latter is also evident. The D5 faults have a brittle-ductile component, reflected by syn-mineralisation foliation, slickensides and stepped veins on fault surfaces, overprinted by a brittle component of fault cataclasite that always crosscuts the veins. The reactivated D2 faults are brittle-ductile, characterised by foliation, tension gashes and mineral lineations, as well as stepped veins on fault surfaces. Both brittle-ductile fault sets crosscut quartz veins, and are crosscut by them, indicating both sets were active at the time of vein formation. The interaction of the two sets of faults produced a network of extension and shear fractures, infilled by veins that are solely dilatational, with no significant consistent vein parallel sense of displacement (Mueller, 2015). They were formed by the stepped dextral displacement on the D5 faults and translation and extension on the reactivated D2 structures in a competent host rock.
The Mount Charlotte-style lodes contain coarse-grained quartz, carbonate, albite and scheelite and are spatially associated with late steeply (~70°) and gently (~45°) dipping, northerly trending brittle dextral-reverse fault sets that offset the regional NW-trending foliation (e.g. Clark, 1980; Ridley and Mengler, 2000; Weinberg et al., 2005; Mueller, 2015). The veins are composed of an inner core of quartz ±scheelite and an outer shell of quartz-dolomite-albite-pyrite (Mueller, 2017). Individual veins vary from a few millimetres to 5 m in thickness and contain multiple generations of quartz growth (Clark, 1980; Bateman and Hagemann, 2004). Quartz, which is unstrained, accounts for ~90% of the vein and is coarsely crystalline with prismatic terminations (Clout et al., 1990). The veins themselves are largely barren of gold but characteristically carry scheelite within the quartz core, occurring as grains from <1 mm to up to 10 cm across. There is a very strong oscillatory zonation in rare earth elements (REE) within these veins on a scale of 1 to 200 µm (Brugger et al., 2000; Ghaderi et al., 1999). These veins form a mutually crosscutting network, and appear to be preferentially developed within rheologically competent units, as described above.
Gold in the Mt Charlotte-style lodes is located on the outer vein margin contacts and in the pyritic selvedges, where it occurs as 5 to 15 µm grains in fractures within pyrite, or at contacts between pyrite and gangue minerals. It is predominantly free, and is associated with pyrite, pyrrhotite, telluride minerals, ankerite, sericite and quartz. Native gold fineness is above 800 (Clout 1989). The well defined selvedges surrounding veins have associated enrichment in K, Rb, Cs, Li, Ba, Ca, Sr, Mg, Ni, V, Cr, W, Te and Au (Mueller, 2015).
The stockwork mineralisation has a distinct zonation of carbonate-sericite-albite-pyrite ±pyrrhotite dominant alteration assemblages surrounding the individual veins. These selvedges are zoned with:
i). a proximal, inner halo that persists for up to ~50 cm from vein margins and is composed of an assemblage of quartz-albite-muscovite-ankerite-ilmenite-rutile-pyrite/pyrrhotite carrying from 5 to 10 g/t Au. It grades outward through
ii). a distal and diffuse gradational zone that is 50 to 100 cm from the vein margins and contains less muscovite and more chlorite and ilmenite and outwardly decreasing gold grades, before passing into
iii). the least altered protolith, carrying 50 to 200 ppb Au, peripheral to the alteration selvedge, representing the background district scale chlorite-calcite alteration of the Golden Mile Dolerite.
The alteration selvedge flanking the veins represents chlorite destruction and growth of ferroan carbonate, sericite, pyrite and native gold. This outer zone of least altered protolith may be 0.5 to >10 m in width. The mineralogy of this protolith is the result of the breakdown of actinolite in the Golden Mile Dolerite to form chlorite and carbonate, as detailed in the Fimiston-style lodes section above. Ore (>2 g/t Au) is formed where the stockwork of veins is sufficiently densely spaced that the mineralised alteration selvedges coalesce to produce a bulk mineable grade. Where this occurs, the remaining least altered chloritic rock accounts for ~25 to 30% of the resource.
The alteration mineralogy enveloping individual veins changes systematically depending on where the vein is within the deposit, as follows (Clark, 1980; Mikucki and Heinrich, 1993; Bateman and Hagemann, 2004; Vielreicher et al., 2016):
• Type 1 - Pyrite-muscovite alteration, where ankerite, muscovite, pyrite, siderite and rutile in the proximal zone and albite, chlorite, magnetite and pyrite occur in the distal zone. This alteration is characterised by the inner proximal halo becoming bleached and muscovite-rich and the dominant sulphide being pyrite. This alteration occurs in the upper and outer levels of the mine, i.e., from the surface to 450 m depth and contains high gold-grades, but at depth, mostly forms an outer shell at the periphery, outside of the bulk mineable orebody. As such it forms a bell shaped cap to the deposit
• Type 2 - Pyrite-pyrrhotite alteration results in an assemblage of quartz, ankerite, muscovite, pyrite, siderite, rutile (pyrrhotite) in the proximal zone, and albite, chlorite, magnetite and pyrrhotite in the distal zone at depths of 600 to 800 m. As such it is similar to the Type 1 alteration, but with the addition of pyrrhotite in the distal diffuse contact zone (i.e. up to 100 cm from the vein margins), and an increase of albite at the expense of muscovite in the inner bleached zones. This is the predominant alteration type associated with economic mineralisation in the uppermost sections of the deposit. It is also common towards the periphery of the orebodies at intermediate levels, and is associated with isolated, distal veins in the deeper part of the deposit, i.e., Charlotte Deeps.
• Type 3 - Pyrrhotite-albite alteration, which consists of assemblages of ankerite, albite, sericite, pyrrhotite, siderite, rutile (pyrite) in the proximal zone, and albite, chlorite, magnetite and pyrrhotite in the distal zone and occurs at depths of >800 m, where pyrrhotite is found in both the inner and outer parts of the alteration envelopes, with a marked increase in the content of albite in the inner zone. This downward expanding zone is the dominant alteration type in the mid to upper core and deeper sections of the Mount Charlotte deposit.
These three types, or alteration assemblages, mark a series of vertical changes, particularly the decrease in pyrite with depth, the reduction of muscovite and corresponding increase in albite. In the inner proximal zone (up to 50 cm from the vein margin), albite increases with depth antithetically to sericite and becomes dominant below the D4b Flanagan thrust, a shallow 330 to 335° and 50 to 55°SW dipping structure offsetting the NW-SE striking deposit near its centre. In the distal zone (50 to 100 cm from the vein margin), sericite and ankerite decline and the protolithic chlorite begins to dominate. Pyrite is the only disseminated sulphide above 450 m depth, but decreases sharply beyond the replacement front of the proximal selvedge. Between 450 and 700 m below surface, pyrite is still the only sulphide in the inner zone, but gives way to pyrrhotite in outer chloritic domains. Below the Flanagan thrust, pyrrhotite dominates over pyrite in the inner selvedge, also.
These changes in the zoned vein selvedges where gold is hosted are reflected in the grade of bulk mineable gold in the Charlotte deposit, which declines from 5.0 g/t at ~125 m below surface to ~3.0 g/t Au at a depth of ~900 m (Mueller, 2015). Fluid inclusion data and chlorite thermometry indicate that the down-plunge zonation is due to the cooling of the hydrothermal fluid from 440 to 410°C at the lower levels to 360 to 350°C at the top of the Charlotte orebody, while the greenstone terrane remained at a 250°C ambient temperature and at 300 MPa lithostatic pressure (Mueller, 2015). In the bulk ore, this decrease in temperature is also reflected in a decreasing Au/Ag ratio and increasing gold, arsenic and mercury contents in the upper sections of the deposit (Mueller, 2015; Bateman and Hagemann, 2004).
The extension and shear fracture network opened by interactions between the dextral D5 and reactivated D2 structures, as described above, was infiltrated by high-pressure H2S rich fluid at 2655 ±13 Ma (xenotime U-Pb; Rasmussen et al., 2009) and 2644 ±11 Ma (xenotime; Vielreicher et al., 2010). Gold was deposited during wall rock sulphidation in overlapping vein selvages at a temperatures gradient as detailed in the previous paragraph. The open fractures filled with barren quartz and scheelite during retrograde cooling of the hydrothermal event, through ~300°C. During the sealing of these fractures, fluid flux was periodically restricted at the lower D4b thrusts. Cycles of high and low up-flow, represented by juvenile H2O-CO2 and evolved H2O-CO2-CH4 fluid, respectively, are recorded by the REE and Sr isotope compositions of scheelite oscillatory zones (Mueller, 2015).
Mueller (2015) suggest the temperature gradient measured in the vein stockwork points to a hot (>600°C) fluid source 2 to 4 km below the mine workings, and several kilometres above the supracrustal rocks. Geochemical characteristics of the ore/alteration related elements are consistent with the local high-Mg monzodiorite/hornblende-phyric porphyry suite but not with the Golden Mile Dolerite host rock. Similarly, 87Sr/86Sr ratios of the vein scheelite are higher than the mantle ratio of the Golden Mile Dolerite and overlap those of high-Mg monzodiorite/hornblende-phyric porphyry intrusions emplaced along the Golden Mile Fault at 2662 ±6 to 2658 ±3 Ma (Mueller, 2015).
A final chlorite-stable event dated at 2603±18 Ma (xenotime U-Pb; Vielreicher et al., 2010) overprints both the Golden Mile and Mount Charlotte deposits. This age is coeval with published 40Ar/39Ar white mica data from the Golden Mile, and overlaps an ~2.61 to 2.60 Ga event recorded at most
deposits in the Kalgoorlie Terrane (Vielreicher et al., 2015). It most probably coincides with the final terrane uplift, cooling and stabilization (Witt et al., 1996).
Formation of the Kalgoorlie Gold Field
According to Vielreicher et al. (2016) both the Golden Mile and Mount Charlotte deposits comprise i). shear-zone related gold-bearing pyrite-telluride disseminations, veinlets and breccias (predominantly at Fimiston) that are structurally overprinted by ii). quartz vein stockworks with gold-bearing sericite-albite-pyrite/pyrrhotite selvedges (dominantly at Mt Charlotte) that carry a significantly lower proportion of telluride minerals. Studies of the mineralogical characteristics, fluid inclusion and isotopic studies all show this mineralisation is consistent with formation from a dominantly low-salinity, 18O enriched, potassic H2O-CO2(+CH4) fluid (or fluids). These studies all indicate that these fluids formed mineralisation at similar temperature (>400 to 300°) and pressure (110 to 300 MPa) ranges. The fluids were transmitted through openings in fractured rheologically favourable competent rocks to deposit gold by reacting with chemically favourable wall rocks, in conjunction with decreasing solubility of gold complexes during declining temperature and rapid pressure release (Vielreicher et al., 2016).
Vielreicher et al. (2016) note that the presence of methane in the ore fluid is attributed to either mixing with an externally derived fluid, or to post-entrapment fluid modification (e.g. Hagemann and Brown, 1996; Mernagh et al., 2004). Alternatively, it could be the result of fluid-rock interaction and reduction of juvenile carbon dioxide (Mikucki and Heinrich, 1993; Polito et al., 2001), generated during desulphidation of the ore fluid, which also caused destabilisation of gold thiosulphide complexes and precipitation of pyrite and gold.
Precise geochronology indicates the formation of the Fimiston and Mount Charlotte lode-styles was temporally close, within ~10 m.y. of each other, at ~2.64 Ga, during D5, after peak metamorphism at ~2.65 Ga, and ~20 to 30 m.y. after the peak of intrusion of the feldspar-phyric porphyry dykes at ~2.67 Ga, and followed deposition of the Black Flag volcaniclastic rocks at ~2.69 to 2.655 Ga; (Krapez et al., 2000). While the gold mineralisation appears to have been a single protracted event, a paragenetic evolution can be recognised, as detailed above (e.g., Groves et al., 2018).
Both the Golden Mile and Mount Charlotte deposits are each largely confined between a pair late stage dextral faults oblique to the main structural trend that have accompanied reactivation of earlier structures. These fault pairs are the Adelaide and Golden Pike faults on the Golden Mile and the Charlotte and Maritana faults at Mount Charlotte (Vielreicher et al., 2016; Groves et al., 2018).
Kalgoorlie sits near the centre of an ~180 km diameter block of preserved greenstones/supracrustals, the largest in the Eastern Gold Fields Superterrane.
The mineralised system at Kalgoorlie was formed on a kilometre scale (at least), adjacent to the active, crustal-scale, Boulder-Lefroy fault system that allowed rapid uplift and erosion of several kilometres of both the Kambalda and Kalgoorlie sequences and deposition of volcanic and clastic sedimentary basinal rocks of the Late Clastic Basin sequences at 2.658 to 2.655 Ga (Vielreicher et al., 2016). The faults of this system are also considered to have provided efficient plumbing system for magmas, including deep lithosphere derived lamprophyres found within the Gold Field. Hydrothermal fluids may have also traversed the same structures from the deep crust/lithosphere to reach the interconnected mesh of upper crustal structures in an uplifted block (inlier) of ultramafic to mafic greenstone rocks within the Kalgoorlie Gold Field. The Boulder-Lefroy fault system is interpreted to be spatially associated with a series of significant gold deposits, extending from Noresman in the south, past Kambalda-Saint Ives, New Celebration, Kalgoorlie, Kanowna and Paddington, which collectively account for ~50% of the gold produced from the Yilgarn Craton (Vielreicher et al., 2016).
Kalgoorlie sits within the core of a complex antiformal structure formed by the interaction of D2 to D5 folding and thrusting (Vielreicher et al., 2016). This antiform isolated a relatively brittle mafic sequence, including the Golden Mile Dolerite, within a relatively incompetent and impermeable envelope of Black Flag volcanosedimentary and sedimentary rocks forming a trap and depositional site for mineralised fluids (Vielreicher et al., 2016). The Kalgoorlie Gold Field is located adjacent to a NW-trending dilational jog in the regional NNW-trending Boulder-Lefroy Fault. This jog results in the trend of the latter being deflected from NNW south of the deposit, to NW to its north. This bend is also reflected in a change in the strike of the Golden Mile Fault and the axial planes of the Kalgoorlie Syncline-Anticline pair, which are also buckled to produce changes in dip (Blewett et al., 2010; Vielreicher et al., 2016).
Data from deep seismic surveys (Drummond et al., 2000), and magnetotelluric (MT) surveys (Henson and Blewett, 2006) show broad anomalies below Kalgoorlie which may reflect alteration and the passage of large volumes of fluids (e.g., Blewett et al., 2010). In the MT data, both the upper crust and upper mantle are anomalously conductive. A domed upper-mantle conductivity (MT) anomaly beneath Kalgoorlie is coincident with the edge of a deep seismic tomographic velocity anomaly. The latter may reflect a favourable deep architecture which acted as a fluid pathway (Blewett et al., 2010). The lower crust beneath Kalgoorlie is also anomalously devoid of reflections in the deep seismic data, which may also represent widespread alteration (Blewett et al., 2010; Drummond et al., 1993; Goleby et al., 1993). These data and interpretations all suggest large scale lithospheric to crustal scale fluid circulation and transport below the Kalgoorlie Gold Field, within thinned, rifted crust of the Kalgoorlie Terrane, just to the east of the older, thicker Youanmi Terrane basement margin.
Production, Reserves and Resources
The bulk of the gold from the Kalgoorlie Gold Field is mined from two operations, i). the KCGM Superpit at Fimiston that is ~3.5 x 1.5 km in area, scheduled to reach 700 m deep, and exploits the remains of >1000 named ore lodes and intervening mineralisation, and ii). the Mount Charlotte deposit, which is 3 km to the NW, made up of four orebodies Reward, Charlotte, Maritana and Charlotte Deeps, consisting of predominantly brittle-style vein stockworks that extend for ~1000 m along strike x 1100 m vertically, and up to 90 m in width.
Total production from the Kalgoorlie Gold Field, dominantly from the Golden Mile and Mount Charlotte mines between 1893 and 2005 was ~1475 t Au (47.5 Moz), with a further ~110 t from 2005 to 2010. By 2017 the gold field had yielded ~1866 t of gold with a remaining endowment of ~363 t of gold in 2018.
Production from the northern part of the field, including the Mount Percy mine totalled ~10 t of gold from three open pits between 1985 and 1992. The Hannan's North mine, also to the north, had been worked since 1893, but was taken over and expanded by Broken Hill Proprietary from 1931. It operated until 1968, producing 0.838 Mt of ore averaging 11.1 g/t Au for 9.286 t of gold.
The total open pit and underground reserves plus resources at December 31, 2010 were (Barrick, Newmont, 2011):
Proved + probable reserves - 153 Mt @ 1.71 g/t Au, for 260 t Au; (reserves are additional to resources)
Measured + indicated resources - 95.6 Mt @ 0.76 g/t Au, for 72 t Au;
Inferred resource - 2.24 Mt @ 4.47 g/t Au, for 10 t Au.
The total open pit and underground reserves plus resources at December 31, 2018 were (Barrick, Newmont, 2018):
Proved + probable reserves - 192.776 Mt @ 1.18 g/t Au, for 227.5 t Au; (reserves are additional to resources)
Measured resources - 10.686 Mt @ 1.42 g/t Au, for 15.17 t Au;
Indicated resources - 50.910 Mt @ 1.51 g/t Au, for 76.87 t Au;
Inferred resource - 18.804 Mt @ 2.33 g/t Au, for 43.81 t Au.
TOTAL Mineral Resource - 80.40 Mt @ 1.69 g/t Au, for 187.33 t Au.
TOTAL Ore Reserve + Mineral Resource - 273.17 Mt @ 1.33 g/t Au, for 363 t Au.
Production in 2010 totalled approximately 24.5 t of recovered Au (Barrick and Newmont, 2011) and in 2018 ~22 t per annum (KCGM website).
Remaining Ore Reserves and Mineral Resources as at 31 March, 2022 (Northern Star Reserves and Resources Report to ASX, 2022) were:
Mineral Resources
Measured Resources - stockpile - 122.976 Mt @ 0.7 g/t Au;
Indicated Resources - open pit - 219.505 Mt @ 1.8 g/t Au; Underground - 49.440 Mt @ 2.2 g/t Au; TOTAL - 268.945 @ 1.8 g/t Au;
Inferred Resources - open pit - 99.288 Mt @ 1.3 g/t Au; Underground - 54.758 Mt @ 2.4 g/t Au; TOTAL - 154.046 @ 1.7 g/t Au;
TOTAL Resources - 545.967 Mt @ 1.6 g/t Au for 850 t of contained gold.
Ore Reserves (included in Mineral Resources)
Proved Reserves - stockpile - 122.976 Mt @ 0.7 g/t Au;
Proved Reserves - open pit - Nil; Underground - Nil;
Probable Reserves - open pit - 140.035 Mt @ 1.7 g/t Au; Underground - 17.839 Mt @ 2.0 g/t Au; TOTAL - 157.874 @ 1.8 g/t Au;
TOTAL Reserves - 280.850 Mt @ 1.3 g/t Au for 371 t of contained gold.
The most recent source geological information used to prepare this decription was dated: 2012.
This description is a summary from published sources, the chief of which are listed below. © Copyright Porter GeoConsultancy Pty Ltd. Unauthorised copying, reproduction, storage or dissemination prohibited.
Kalgoorlie, KCGM Super Pit
|
|
Anonymous 1998 - Super Pit: in Register of Australian Mining 1997/98 p 167
|
Anonymous 1998 - Normandy Mining Limited: in Extracts from the Normandy Mining Limited 1997 Annual Report pp 12-14, 17, 26-28.
|
Anonymous 1998 - Normandy Mining Limited (Extract): in Extracts from the Normandy Mining Limited 1997 Annual Report pp 12-14, 26-28
|
Anonymous 1998 - Mount Charlotte: in Register of Australian Mining 1997/98 p 135
|
Bateman R and Bierlein F P, 2007 - On Kalgoorlie (Australia), Timmins-Porcupine (Canada), and factors in intense gold mineralisation: in Ore Geology Reviews v32 pp 187-206
|
Bateman, R. and Hagemann, S., 2004 - Gold mineralisation throughout about 45 Ma of Archaean orogenesis: protracted flux of gold in the Golden Mile, Yilgarn craton, Western Australia: in Mineralium Deposita v.39, pp. 536-559.
|
Blewett, R.S., Henson, P.A., Roy, I.G., Champion, D.C. and Cassidy, K.F., 2010 - Scale-integrated architecture of a world-class gold mineral system: The Archaean eastern Yilgarn Craton, Western Australia: in Precambrian Research v.183, pp. 230-250.
|
Boulter C A, Fotios M G, Phillips G N 1987 - The golden mile, Kalgoorlie: a giant Gold deposit localized in ductile shear zones by structurally induced infiltration of an auriferous metamorphic fluid: in Econ. Geol. v82 pp 1661-1678
|
Clout J M F, Cleghorn J H, Eaton P C 1990 - Geology of the Kalgoorlie Gold Field: in Hughes FE (Ed.), 1990 Geology of the Mineral Deposits of Australia & Papua New Guinea The AusIMM, Melbourne Mono 14, v1 pp 411-431
|
Dunga, J., Sully, D., Hagemann, S.G., Duuring, P. and Danyushevsky, L., 2021 - Structural setting, wall rock alteration and gold mineralisation of the Mt. Percy gold deposit, Kalgoorlie, Western Australia: in Mineralium Deposita v.56, pp. 1449-1470.
|
Evans, K.A, Phillips, G.N. and Powell, R., 2006 - Rock-Buffering of Auriferous Fluids in Altered Rocks Associated with the Golden Mile- Style Mineralization, Kalgoorlie Gold Field, Western Australia: in Econ. Geol. v.101, pp. 805-818.
|
Gauthier, L., Hagemann, S. and Robert, F., 2004 - Architecture and timing of gold mineralization at the Golden Mile gold deposit, Kalgoorlie, Western Australia: in Neumayr, P., Walshe, J.L. and Hagemann, S., 2005 Final Report, Camp to Deposit-Scale Alteration Footprints in the Kalgoorlie-Kambalda Area, Project Y3, 2002-2004, Predicitive Mineral Discovery Cooperative Research Centre, Part 2, Report 3, 95p.
|
Godefroy-Rodriguez, M., Hagemann, S., Frenzel, M. and Evans, N.J., 2020 - Laser ablation ICP-MS trace element systematics of hydrothermal pyrite in gold deposits of the Kalgoorlie district, Western Australia: in Mineralium Deposita v.55, pp. 823-844.
|
Godefroy-Rodriguez, M., Hagemann, S., LaFlamme, C. and Fiorentini, M., 2020 - The multiple sulfur isotope architecture of the Golden Mile and Mount Charlotte deposits, Western Australia: in Mineralium Deposita v.55, pp. 797-822.
|
Groves, D.I., Santosh, M., Goldfarb, R.J. and Zhang, L., 2018 - Structural geometry of orogenic gold deposits: Implications for exploration of world-class and giant deposits: in Geoscience Frontiers v.9, pp. 1163-1177.
|
Johnston S F, Sauter P C C, Hyland S J, Bradley T 1990 - Mount Percy Gold deposits: in Hughes F E (Ed.), 1990 Geology of the Mineral Deposits of Australia & Papua New Guinea The AusIMM, Melbourne Mono 14, v1 pp 433-437
|
Kent A J R, Campbell I H, McCulloch M T 1995 - Sm-Nd systematics of hydrothermal Scheelite from the Mount Charlotte Mine, Kalgoorlie, Western Australia: an isotopic link between Gold mineralization and Komatiites: in Econ. Geol. v 90 pp 2329-2335
|
Kent A J R, McDougall I 1995 - 40Ar-39Ar and U-Pb age constraints on the timing of Gold mineralization in the Kalgoorlie Gold field, Western Australia: in Econ. Geol. v90 pp 845-859
|
McDivitt, J.A., Hagemann, S.G., Kemp, A.I.S., Thebaud, N., Fisher, C.M. and Rankenburg, K., 2022 - U-Pb and Sm-Nd Evidence for Episodic Orogenic Gold Mineralization in the Kalgoorlie Gold Camp, Yilgarn Craton, Western Australia: in Econ. Geol. v.117, pp. 747-775.
|
McDivitt, J.A., Hagemann, S.G., Thebaud, N., Martin, L.A.J. and Rankenburg, K., 2021 - Deformation, Magmatism, and Sulfide Mineralization in the Archean Golden Mile Fault Zone, Kalgoorlie Gold Camp, Western Australia : in Econ. Geol. v.116, pp. 1285-1308.
|
McDivitt, J.A., Hagemann, S.G., Thebaud, N., Martin, L.A.J. and Rankenburg, K., 2022 - Constraints on the structural setting, relative timing, and geochemistry of the Fimiston, Hidden Secret, and Oroya gold-telluride lode types, Kalgoorlie gold camp, Western Australia: in Mineralium Deposita v.57, pp. 1023-1046.
|
McNaughton N J, Mueller A J and Groves D I, 2005 - The Age of the Giant Golden Mile Deposit, Kalgoorlie, Western Australia: Ion-Microprobe Zircon and Monazite U-Pb Geochronology of a Synmineralization Lamprophyre Dike: in Econ. Geol. v100 pp 1427-1440
|
Morey, A.A., Weinberg, R.F., Bierlein, F.P. and Davidson, G.J., 2007 - Gold deposits of the Bardoc Tectonic Zone: a distinct style of orogenic gold in the Archaean Eastern Goldfields Province, Yilgarn Craton, Western Australia : in Australian J. of Earth Sciences v.54, pp. 783-800.
|
Mueller, A.G. and Muhling, J.R., 2020 - Early pyrite and late telluride mineralization in vanadium-rich gold ore from the Oroya Shoot, Paringa South mine, Golden Mile, Kalgoorlie: 3. Ore mineralogy, Pb-Te (Au-Ag) melt inclusions, and stable isotope constraints on fluid sources: in Mineralium Deposita v.55, pp. 733-766.
|
Mueller, A.G., 2022 - Archean intrusion-related Au-Te and Cu-Au deposits in the Boulder Lefroy-Golden Mile fault system, Western Australia: an overview,: in 16th SGA Biennial Meeting, Rotorua, New Zealand, 28-31 March 2022, Proceedings, v.1, pp. 271-274.
|
Mueller, A.G., 2015 - Structure, alteration, and geochemistry of the Charlotte quartz vein stockwork, Mt Charlotte gold mine, Kalgoorlie, Australia: time constraints, down-plunge zonation, and fluid source: in Mineralium Deposita v.50 pp. 221-244
|
Mueller, A.G., 2020 - Paragonite-chloritoid alteration in the Trafalgar Fault and Fimiston- and Oroya-style gold lodes in the Paringa South mine, Golden Mile, Kalgoorlie: 2. Muscovite-pyrite and silica-chlorite-telluride ore deposited by two superimposed hydrothermal syst: in Mineralium Deposita v.55, pp. 697-730.
|
Mueller, A.G., 2020 - Structural setting of Fimiston- and Oroya-style pyrite-telluride-gold lodes, Paringa South mine, Golden Mile, Kalgoorlie: 1. Shear zone systems, porphyry dykes and deposit-scale alteration zones: in Mineralium Deposita v.55, pp. 665-695.
|
Mueller, A.G., 2007 - Copper-gold endoskarns and high-Mg monzodiorite-tonalite intrusions at Mt. Shea, Kalgoorlie, Australia: implications for the origin of gold-pyrite-tennantite mineralization in the Golden Mile : in Mineralium Deposita v.42, pp. 737-769.
|
Mueller, A.G., 2017 - Mount Charlotte deposit, Kalgoorlie goldfield: in Phillips, G.N., (Ed.), 2017 Australian Ore Deposits, The AusIMM, Melbourne, Mono 32, pp. 195-198.
|
Mueller, A.G., Hagemann, S.G. and McNaughton, N.J., 2020 - Neoarchean orogenic, magmatic and hydrothermal events in the Kalgoorlie-Kambalda area, Western Australia: constraints on gold mineralization in the Boulder Lefroy-Golden Mile fault system: in Mineralium Deposita v.55, pp. 633-663.
|
Mueller, A.G., Hagemann, S.G., Brugger, J., Xing, Y. and Roberts, P.R., 2020 - Early Fimiston and late Oroya Au-Te ore, Paringa South mine, Golden Mile, Kalgoorlie: 4. Mineralogical and thermodynamic constraints on gold deposition by magmatic fluids at 420-300°C and 300 MPa: in Mineralium Deposita v.55, pp. 767-796.
|
Mueller, A.G., Harris, L.B. and Lungan, A., 1988 - Structural control of greenstone-hosted Gold mineralization by transcurrent shearing: A new interpretation of the Kalgoorlie mining district, Western Australia: in Ore Geology Reviews v.3, pp. 359-387.
|
Mueller, A.G., McNaughton, N.J. and Muhling, J.R., 2021 - Albite ± actinolite-altered porphyry dykes in Archean gold deposits of the Boulder Lefroy-Golden Mile fault system, Yilgarn Craton, Western Australia: Petrography, chronology and comparison to Canadian albitites.: in Minerals (MDPI) v.11, doi.org/10.3390/min11111288.
|
Phillips G N 1986 - Geology and alteration in the Golden Mile, Kalgoorlie: in Econ. Geol. v81 pp 779-808
|
Phillips, G.N., Groves, D.I. and Kerrich, R., 1996 - Factors in the formation of the giant Kalgoorlie gold deposit: in Ore Geology Reviews v.10, pp. 295-317.
|
Phillips, G.N., Groves, D.I., Neall, F.B., Donnelly, T.H. and Lambert, I.B., 1986 - Anomalous Sulfur isotope compositions in the Golden Mile, Kalgoorlie: in Econ. Geol. v.81, pp. 2008-2015.
|
Phillips, G.N., Hergt, J. and Powell, R., 2017 - Kalgoorlie goldfield - petrology, alteration and mineralisation of the Golden Mile Dolerite: in Phillips, G.N., (Ed.), 2017 Australian Ore Deposits, The AusIMM, Melbourne, Mono 32, pp. 185-194.
|
Ridley, J. and Mengler, F., 2000 - Lithological and structural controls on the form and setting of vein stockwork orebodies at the Mount Charlotte Gold deposit, Kalgoorlie: in Econ. Geol. v95 pp 85-98
|
Shackleton J M, Spry P G, Bateman R 2004 - Telluride mineralogy of the Golden Mile deposit, Kalgoorlie, Western Australia: in The Canadian Mineralogist v41 pp 1503-1524
|
Steadman, J.A., Large, R.R., Meffre, S., Olin, P.H., Danyushevsky, L.V., Gregory, D.D., Belousov, I., Lounejeva, E., Ireland, T.R. and Holden, P., 2015 - Synsedimentary to Early Diagenetic Gold in Black Shale-Hosted Pyrite Nodules at the Golden Mile Deposit, Kalgoorlie, Western Australia : in Econ. Geol. v.110 pp. 1157-1191
|
Vielreicher N M, Groves D I, Snee L W, Fletcher I R and McNaughton N J, 2010 - Broad Synchroneity of Three Gold Mineralization Styles in the Kalgoorlie Gold Field: SHRIMP, U-Pb, and 40Ar/39Ar Geochronological Evidence: in Econ. Geol. v105 pp 187-227
|
Vielreicher, N.M., Groves, D.I. and McNaughton, N.J., 2016 - The giant Kalgoorlie Gold Field revisited: in Geoscience Frontiers, v.7, pp. 359-374
|
Weinberg R F, Van der Borgh P, Bateman R J and Groves D I, 2005 - Kinematic History of the Boulder-Lefroy Shear Zone System and Controls on Associated Gold Mineralization, Yilgarn Craton, Western Australia : in Econ. Geol. v100 pp 1407-1426
|
Witt W K, Knight J T, Mikucki E J 1997 - A synmetamorphic lateral fluid model for Gold mineralization in the Archean southern Kalgoorlie and Norseman Terranes, Western Australia: in Econ. Geol. v92 pp 407-437
|
Porter GeoConsultancy Pty Ltd (PorterGeo) provides access to this database at no charge. It is largely based on scientific papers and reports in the public domain, and was current when the sources consulted were published. While PorterGeo endeavour to ensure the information was accurate at the time of compilation and subsequent updating, PorterGeo, its employees and servants: i). do not warrant, or make any representation regarding the use, or results of the use of the information contained herein as to its correctness, accuracy, currency, or otherwise; and ii). expressly disclaim all liability or responsibility to any person using the information or conclusions contained herein.
|
Top | Search Again | PGC Home | Terms & Conditions
|
|