Fishtie, Kashime |
|
Zambia |
Main commodities:
Cu
|
|
 |
|
 |
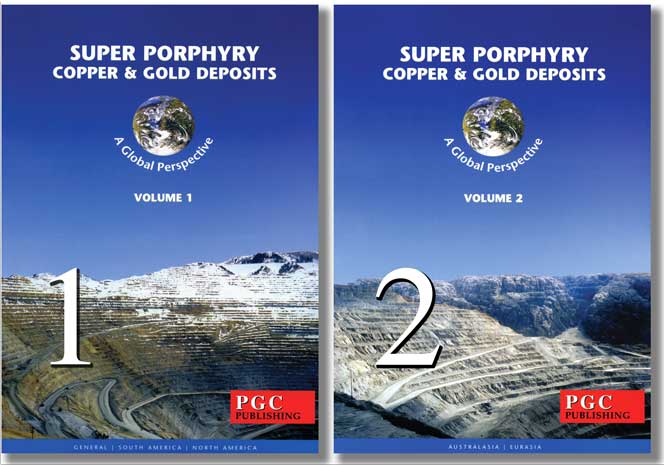 |
Super Porphyry Cu and Au

|
IOCG Deposits - 70 papers
|
All papers now Open Access.
Available as Full Text for direct download or on request. |
|
 |
The Fishtie (formerly Kashime) sedimentary hosted copper deposit, is located ~90 km SE of Ndola on the Zambian Copperbelt, ~30 km north of the village of Mkushi, Zambia and ~5 km south of the Congolese border (#Location: 13° 21' 21"S, 29° 24' 32"E).
Regional Setting
The Fishtie deposit is situated on the northern margin of the Lusale structural basin, a generally NE trending Katangan outlier enclosed by antiformal upfolded basement rocks composed of metavolcanic and metasedimentary rocks of the Irumide Belt. It is also located towards the eastern margin of the southern extension of the NW-trending Kafue Anticline that forms the core of the Zambian Copperbelt further to the north (Stillman, 1965).
The units hosting the deposit overlie the northeast-striking late Palaeoproterozoic to Mesoproterozoic Irumide Belt basement rocks that extend from central Zambia into northern Malawi (Dewaele et al., 2006). Regionally, these basement rocks are composed of a complexly deformed package of metamorphosed sedimentary, volcanic and intrusive lithologies. The Irumide sequence below the Lusale basin overlies a basement of 2.05 to 1.95 Ga Mkushi Gneiss Complex, characterised by banded biotite gneisses, augen gneisses and porphyritic granite gneisses (De Waele et al., 2006). These metamorphics, which are probable correlates of the Lufubu Metamorphic Complex to the north, are structurally and in places unconformably overlain by 1.85 to 1.65 Ga metasedimentary rocks of the lower section of the Muva Supergroup (Stillman 1965). Dating reported by De Waele (2004) and De Waele and Fitzsimons (2007) suggest the Irumide rocks were deposited in two episodes, the first, an extensive quartzite-metapelite succession with minor carbonate (Mporokoso, Kanona and Manshya River groups), deposited in the Palaeoproterozoic at ~1.8 Ga (based on interlayered 1815 Ma volcanic rocks), and a second, more restricted sequence which consists largely of very mature, fluvial sandstones (Kasama Formation) at ~1.4 Ga that are recycled sediments from the first episode rocks (De Waele, 2004). The more restricted Muva Group of the Zambian Copperbelt are probably equivalents of this younger sequence, and assumed to be ~1.3 to 1.05 Ga (Selley et al., 2005), but may be older. Peak metamorphism in the Irumide sequence was at 1.05 to 1.02 Ga (Rainaud et al., 2005). Voluminous syn- to post-kinematic Irumide granitoids were emplaced between 1.05 and 0.95 Ga.
Locally, the Muva Supergroup rocks dominantly belong to the Mafingi or Manshya River groups, and largely comprise muscovite-kyanite-sillimanite, quartz-muscovite, biotite and garnet-biotite schists and quartzites (Stillman 1965), representing quartz arenites and metapelitic rocks (Dewaele et al., 2006). Rocks of both the Mkusi Gneiss Complex and Muva Supergroup are intruded by 1.65 and 1.05 to 1.02 Ga granitoids. On the basis of aeromagnetic data, the Irumide metamorphic rocks below and adjacent to the Lusale basin are interpreted to have been intruded by two major (~10 km diameter) sub-circular igneous bodies of unknown age, that are 2.5 km NW and 15 km SW of Fishtie respectively (Hendrickson, 2013).
The Roan Group rocks of the Zambia Copperbelt, deposited as a rift-sag succession between ~880 and ~750 Ma, are absent from the Lusale basin. The basement metamorphic complex is directly overlain by the basal unit of the Nguba Group, the Grand Conglomérat Formation, interpreted to be a correlative of the ~740 Ma (Xu et al., 2009) Sturtian glacial diamictites that are recognised around the world and throughout the Central African Copperbelt. The Nguba Group, which was regionally accompanied by mafic volcanism and intrusion, was deposited in response to a period of renewed extension, and spreading of the Katangan sequence onto the previous basin margins. At Fishtie, the Grand Conglomérat Formation comprises <150 m of very weakly carbonaceous diamictites that likely represent debris flows, together with lesser of siltstone and lenses of magnetite-ankerite-quartz that locally have the appearance of a banded iron formation. This basal unit is overlain by carbonate rocks and carbonate-bearing to carbonate-poor siltstones and sandstones of the Kakontwe Limestone Formation (Cailteux et al., 2007). The sequence of Nguba Group dolomitic sandstones and siltstones that normally overlie the Kakontwe Formation elsewhere (Batumike et al., 2006, 2007), are absent at Fishtie (Hendrickson et al., 2015), as is the succeeding Kundelungu Group.
This condensed Katangan succession was subjected to Pan-African inversion and deformation during the ~590 to 500 Ma Lufilian orogeny (Cahen et al., 1984; Kampunzu and Cailteux, 1999; Rainaud et al., 2005). Regionally, the Lufilian metamorphism and deformation was heterogeneous, with biotite/phlogopite-bearing assemblages of greenschist to lower amphibolite facies in the main Zambian Copperbelt to the north (Ramsay and Ridgeway 1977), while at Fishtie, the host sequence only contains a mineral assemblage compatible with greenschist facies, and no pronounced metamorphic fabrics (Hendrickson et al., 2015).
For more detail on the regional setting see the separate Zambian Copperbelt record.
Deposit geology
The geology of the deposit and surrounding district may be summarised as follows:
Basement
At Fishtie, the immediate basement, which has only been drilled to a maximum of 40 m below the Katangan hosts, principally comprises interlayered schist and quartzite of the Muva Supergroup. The schists are mostly composed of muscovite and quartz with subordinate chlorite, biotite and dolomite and exhibit a prominent foliation at a low angle to bedding. Muscovite is present as euhedral laths up to several millimetres in length, defining a distinct foliation, with fine-grained white mica aggregates commonly rimming larger muscovite flakes. Subhedral grains of quartz, up to 2 mm in diameter, give the schist an inequigranular, interlobate texture, with minor, subhedral, up to 200 µm diameter potassium feldspar grains. The latter is typically altered to muscovite or fine-grained white mica. Plagioclase is absent within basement rocks (Stillman,1965; Hendrickson et al., 2015). Biotite is found sporadically as up to 100 µm diameter anhedral crystals intergrown with muscovite, whilst dolomite occurs as irregular patches, overgrown with, or embayed by muscovite, fine-grained white mica, chlorite and biotite. Dolomite grains are rimmed, and locally replaced by large (>750 µm) fans of chlorite, which also occurs as small- to moderate-sized crystals intergrown with, and replacing, fine-grained white mica and locally biotite. Quartzite layers are composed of 10 µm to 2 mm diameter, sub- to anhedral, undulose quartz grains with an intragranular seriate texture, exhibiting sub-grain rotation and grain boundary migration. Intergrown muscovite, biotite and chlorite are interstitial to quartz grains, whilst minor green, pleochroic, tourmaline occurs as <15 µm euhedral crystals (Hendrickson et al., 2015).
Angular unconformity
Nguba Group
• Grand Conglomérat Formation - which at Fishtie ranges from ~20 to 150 m in thickness, and is dominantly composed of diamictite, intercalated with subsidiary siltstone and magnetite-ankerite-apatite lenses. It appears to be thickest adjacent to inferred high-angle normal faults suggesting these structures represent syn-depositional growth faults. Rapid thickness changes within this unit are also recorded elsewhere along the eastern edge of the Zambian Copperbelt (e.g., in the Itawa area to the north; Binda and Van Eden, 1972). Individual diamictite beds range from 5 to 30 m in thickness, and are massive and matrix supported, with unsorted and unorientated clasts comprising 15 to 30 % of the rock. Clasts are typically subangular to subrounded, and range from <1 mm to >2 m in diameter. The lowest beds are generally more clast-rich compared to those near the top. Clasts are commonly of basement lithologies, including quartzite, muscovite-quartz schist and vein quartz, and less frequently dolostone, limestone and albitised and/or chloritised mafic igneous rocks similar to the intrusions within the Upper Roan Subgroup to the north (Hendrickson et al., 2015).
The dark blue-grey to grey-green coloured diamictite matrix is fine-grained (2.5 to 300 µm), and where distal to iron oxide or sulphide mineralisation, is composed of quartz, albite, K feldspar, white mica, calcite and dolomite, with lesser chlorite, biotite and heavy minerals which include tourmaline, apatite and rutile. Small anhedral grains of pyrite, and more rarely pyrrhotite, are found sporadically throughout the matrix as is typical within the Grand Conglomérat throughout the Central African Copperbelt. Approximately ~20% of the matrix comprises subrounded to angular, 10 to 300 µm, apparently detrital grains of quartz, potassium feldspar and muscovite, with quartz being the most common. Many of the quartz grains have an irregular shape, partly the result of dissolution and etching of authigenic quartz overgrowths. Quartz grains, including those with authigenic quartz rims, are encapsulated by a thin coating of potassium feldspar and/or muscovite (Hendrickson et al., 2015).
Detrital calcic plagioclase has not been observed at Fishtie, although small subangular to subrounded detrital grains, possibly after calcic plagioclase, are now composed of albite. Albite grains are, in turn, locally altered to assemblages of muscovite, biotite and chlorite. K feldspar grains are small and subrounded to subangular, and may be both detrital, and alteration products after detrital plagioclase. Detrital muscovite grains up to 500 µm in length are occasionally recognised, while possibly detrital carbonate grains are also found within the matrix (Hendrickson et al., 2015). The finest-grained fraction of the matrix is composed of quartz, white mica (the most common phyllosilicate), biotite, chlorite, potassium feldspar and dolomite-ankerite, with lesser rutile and apatite. White mica is preferentially developed adjacent to potassium feldspar grains, whilst biotite is usually intergrown with chlorite. Very minor rutile is present within the matrix as <50 µm subhedral crystals (Hendrickson et al., 2015).
A weakly developed, steeply dipping foliation is occasionally observed within the matrix of the diamictites at Fishtie, particularly near the inferred east-west striking normal faults, and is most pronounced adjacent to lithic clasts. It is defined by the vertical alignment of muscovite, chlorite, biotite and sometimes anhedral dolomite (Hendrickson et al., 2015) and may be the result of fluid dewatering texture formed during diagenesis and hydrothermal alteration as interpreted for a similar foliation at Kamoa by Schmandt et al., 2013).
Subsidiary, 0.1 to 20 m thick laminated and very thin-bedded siltstone layers are intercalated within the Grand Conglomérat Formation at Fishtie, interpreted to represent turbidity current deposits. Individual beds within these intercalations are as much as 5 cm in thickness, commonly with normal grading, and rare slumps and intraformational folds. These siltstones are fine-grained, dominantly composed of white mica with subsidiary biotite, chlorite, quartz and K feldspar, and rare rounded to angular, 10 to 30 µm grains of apatite. Subhedral crystals or aggregates of fine white mica crystals are found interstitial to quartz, potassium feldspar and carbonate minerals, whilst small subhedral grains of chlorite and biotite are commonly found together. Coarser grained siltstone interbeds contain abundant subrounded, 50 to 200 µm diameter grains and grain aggregates of detrital quartz and feldspar (completely altered to K feldspar) which are commonly cemented by 20 to 60 µm diameter anhedral calcite, dolomite and/or ankerite. Traces of 10 to 20 µm, sub- to anhedral rutile grains and relatively larger, up to 500 µm, sub-spherical crystals of pyrite that form aggregates are also present. The sub-spherical shape of the pyrite grains suggests they were originally diagenetic framboids. Clasts that are up to several centimeters in diameter are locally found within siltstone beds, indenting underlying beds, but draped over by beds above, suggesting they are dropstones, which implies a glaciogenic origin (e.g., Cahen 1978; Hendrickson et al., 2015).
Hendrickson et al. (2015) suggest the textural and structural features of the Grand Conglomérat Formation diamictites at Fishtie imply deposition by high-energy mass-flows/debris flows, and are similar in character to this unit where described elsewhere in the Central Africa Copperbelt (e.g., Cahen 1978; Master and Wendorff 2011).
• Kakontwe Limestone Formation - (after Cailteux et al., 2007) which has a maximum thickness of 120 m at Fishtie, conformably overlies the Grand Conglomérat Formation, and comprises massive limestone, finely bedded to massive or algally laminated dolostone, and rhythmically bedded dolomitic siltstone. Limestone beds have locally been encountered near the base of the formation. However, the basal section is generally composed of massive grey dolostone with poorly preserved planar to domal stromatolitic laminations. This suite is overlain by a distinctive massive dolostone unit containing layers of pink coloured dolostone and thin argillaceous bands, capped by variably dolomitised, algally laminated limestones with thin argillaceous bands, a pinstriped dolostone layer, and dolomitic siltstones. The pinstriped dolostone layer comprises ~5 mm thick (average) intercalated white and pink to grey and green laminae. The finely laminated dolomitic siltstone beds in the upper portions of the sequence at Fishtie appear to be laterally equivalent to variably dolomitised massive limestones found elsewhere (Hendrickson, 2013; Hendrickson et al., 2015).
The massive and pinstriped dolostones are composed of 10 to 100 µm diameter grains of anhedral dolomite, which replaced similarly sized anhedral calcite grains. These dolostones also contain small amounts of disseminated subrounded quartz grains, rare white mica, and minor cubic to subhedral pyrite grains up to 150 µm in diameter. The dolomitic siltstone interval comprises alternating dolomitic, siliciclastic and carbonaceous beds, with individual layers varying from 1 to 30 mm in thickness. Siliciclastic interbeds contain quartz, dolomite and K feldspar, as well as minor white mica, and are generally mildly carbonaceous, with the carbonaceous material forming irregular masses interstitial to dolomite, quartz and K feldspar. Carbonaceous laminae with minor white mica, dolomite, quartz and K feldspar are up to 3 mm thick (Hendrickson, 2013; Hendrickson et al., 2015).
Structure
Regional aeromagnetic data show that the deposit sits on a NNE-trending magnetic high, bounded to the north by a magnetic low that corresponds to an area with thicker Katangan metasedimentary rocks. This has been interpreted to indicate the deposit is underlain by a buried basement horst, but may equally reflect the presence of the magnetite-rich layers within the deposit and its hosts (Hendrickson, 2013; Hendrickson et al., 2015).
A series of sub-vertical normal faults are interpreted to cut the deposit area. Both thickness and facies variations within the Grand Conglomérat and Kakontwe Limestone formations appear to controlled by these structures, which are inferred to offset the contact between the Grand Conglomérat and both the basement below and the Kakontwe Limestone formation above. However, none of the generally vertical drill holes intersect any of these interpreted faults (Hendrickson, 2013; Hendrickson et al., 2015).
These inferred steep structures strike sub-parallel to the Lusale Basin margin, from ENE in the west to ESE in the east, forming an arcuate or boomerang shaped pattern, concave to the south, with down-throw primarily to the south. Drilling suggests these structures have lengths of up to 1.5 km although lateral extensions of many are not well constrained by available data, whilst maximum offsets are of the order of <100 m. Their geometry and the regularity of displacement gradients along individual faults has been interpreted to suggest they may form a series of segmented fault arrays in which displacement was transferred between structures by ramp-relay zones (Walsh and Watterson 1989).
The deposit area has been divided into a series of structural domains over a strike length of 3.75 km, based on the distribution and geometry of these inferred faults. In the western structural domain, there are three NNE striking, sub-vertical to steeply SE dipping faults with normal offsets of 15 to 65 m. Within this domain, the Grand Conglomérat thickens significantly towards the southernmost fault, which has an ~50 m displacement. In this part of the deposit, the Kakontwe Limestone Formation is only found to the south of this southernmost structure, having been either eroded to the north, or is represented in saprolite weathering profile only (Hendrickson, 2013; Hendrickson et al., 2015).
The central structural domain contains the thickest and highest-grade portion of the Fishtie deposit, and is characterised by normal faults that generally trend east-west. It is apparently bounded to the north by one such fault that dips steeply to the north, with ~50 m of north-down displacement, but also contains two similarly striking normal faults with downward displacements to the south. The Grand Conglomérat Formation thickens significantly towards the southernmost of these faults, although no similar thickening is evident along the fault immediately to the north, which has an apparent normal offset of 25 m. Correlations in this domain suggest either that the Kakontwe Limestone is laterally equivalent to the uppermost part of the Grand Conglomérat, or there are additional, currently unrecognised normal faults between these two structures. In both the western and central domains, the longitudinal structures are offset by a series of north-south striking dip-slip faults. In the central structural domain, the longitudinal normal faults are offset by two NE-trending dextral strike-slip faults. Similarly trending normal faults separate the central and eastern structural domains. These steeply dipping faults had normal movement with a combined down-throw to the west of ~60 m (Hendrickson, 2013; Hendrickson et al., 2015).
In the eastern domain, there are at least two longitudinal structures, both of which strike WNW and dip to the SSW. The southernmost and northernmost of these have normal offsets of ~25 and 35 m respectively. Within this domain, the Grand Conglomérat Formation progressively thins to the east, although there is insufficient data to determine whether the normal faulting influenced thickness variations in the stratigraphy (Hendrickson, 2013; Hendrickson et al., 2015).
A fourth, or satellite domain, which is 400 to 500 m long on the eastern margin of the deposit, is separated from the eastern structural domain by two NNE-trending subvertical normal faults that have a combined normal down throw of >100 m. It comprises an area of thicker preserved Kakontwe Limestone Formation, and contains both east-west and north-south striking normal faults. It likely forms a graben bounded by the NNE trending faults to the west, described above, and a similar less well-constrained set to the east, with an offset of only ~15 m. There is insufficient drill data to determine whether the Grand Conglomérat thickness changes relative to the ESE-trending normal faults, although there appears to be no change in the thickness of the same unit relative to the NNE-trending normal structures. However, in contrast, the overlying Kakontwe Limestone Formation, displays thickness and facies changes relative to both fault sets, and contains more dolomitic siltstone to the north and east (Hendrickson, 2013; Hendrickson et al., 2015).
Alteration and Mineralisation
The alteration and mineralisation at Fishtie can be grouped into the following:
Diagenesis
Diagenetic alteration of the Grand Conglomérat sequence is largely masked by later hydrothermal processes. Rare preserved quartz overgrowths observed on quartz grains in both diamictite and siltstone beds are most likely the result of early diagenesis. Other reactions, such as carbonate overgrowths, formation of chlorite from detrital clay, potassium feldspar overgrowths on detrital feldspar and quartz and albite pseudomorphs after detrital plagioclase could have also taken place during early burial to shallow depths (<2 km) and low temperatures (~100°C; e.g., Morad et al., 2000; Tucker 2001).
The abundant stromatolitic textures within the Kakontwe Limestone suggests portion of the unit may have been deposited as dolostone, and with burial, much of the original calcite or aragonite in the unit would probably have been recrystallised, precipitated as carbonate cements and converted to dolomite (Hendrickson, 2013; Hendrickson et al., 2015).
Magnetite-ankerite-quartz-apatite alteration
Iron-rich assemblages of magnetite, ankerite, quartz and apatite, with lesser chlorite and biotite, are restricted to siltstone bearing portions in the uppermost part of the Grand Conglomérat Formation, adjacent to normal faults, and reach a maximum thickness of ~10 m adjacent to the southernmost normal faults in the western and central structural domains. Similar developments have also been intersected on the northern edge of the central structural domain. These assemblages typically occur as alternating bands of magnetite, magnetite-quartz, magnetite-ankerite, ankerite, or apatite. The iron and phosphorous-rich layers are commonly interbedded with siltstone or thin diamictite beds that also contain significant disseminated magnetite and/or ankerite (Hendrickson, 2013; Hendrickson et al., 2015).
Magnetite occurs as up to 100 µm diameter subhedral to euhedral grains, and is most commonly intergrown with quartz. Locally, in the supergene environment, the magnetite has been replaced by hematite, that is, in turn, partially replaced by goethitic iron oxides. Ankerite is found as small to moderate sized (20 to 200 µm) subhedral to anhedral crystals. Apatite is anhedral to subhedral and is up to 200 µm in diameter, occurring as thin, nearly monomineralic bands and/or disseminated grains. Chlorite is common, occurring as 10 to 50 µm anhedral grains rimming or intergrown with magnetite. Some chlorite grains are rimmed by biotite (Hendrickson, 2013; Hendrickson et al., 2015).
Hendrickson (2013) showed that selected samples of magnetite-ankerite-quartz rocks contain up to 46 wt.% Fe2O3 and 32 to 58 wt.% SiO2. They may also have up to 4 wt.% P2O5, 240 ppm Ba, which probably occurs in apatite (e.g., Henderson et al., 2009), <1% Mn and 650 ppm Cu. Where present, chalcopyrite always overgrows magnetite, strongly suggesting the formation of magnetite pre-dates copper mineralisation.
Rocks that have undergone magnetite-ankerite-quartz alteration lack significant muscovite and K feldspar, and usually contain less biotite than typical Grand Conglomérat Formation siltstones, while distinct detrital quartz grains of the type normally found in these siltstones are absent. However, an increase in mineral grain sizes towards the base of iron-rich beds, mimicking graded bedding, is common (Hendrickson, 2013). Hendrickson et al. (2015) interpreted the mineralogy and lack of detrital quartz to suggest the magnetite-ankerite-quartz layers formed as a chemical precipitate. In addition, magnetite-ankerite-quartz clasts are completely absent from the diamictite, even where directly overlying such bands, and the apparent graded bedding observed in some of these iron-rich rocks would be difficult to produce from amorphous iron-rich gels or chemically deposited varves (e.g., Bekker et al., 2010). The same authors also note that siltstone and diamictite beds overlying the magnetite-ankerite-quartz layers commonly contain disseminated 10 to 100 µm diameter anhedral to subhedral magnetite, the distribution and texture of which suggest they grew within the diamictite after deposition. They therefore conclude, that these observations and the textural evidence, as well as the geometry of magnetite-ankerite-quartz lenses that thicken towards adjacent normal faults, suggests the magnetite-ankerite-quartz layers formed by precipitation and replacement within permeable siltstones of the Grand Conglomérat, caused by hydrothermal fluids, emanating from growth fault conduits, and percolating outward into the host beds.
Post-iron oxide alteration
Both the Grand Conglomérat and Kakontwe Limestone formations have undergone pervasive hydrothermal alteration that is generally coeval with sulphide mineralisation, and subsequent to the magnetite-ankerite-quartz-apatite phase. This alteration is distinguished by subtle colour changes, compared to the dull grey unaltered diamictite, and comprises the following (after Hendrickson et al., 2015):
• Pale grey, muscovite-rich matrix diamictite is the most widespread. Muscovite occurs as crystal aggregates overgrowing detrital and diagenetic quartz and albite, disseminated magnetite and apatite, and also commonly replaces K feldspar. Muscovite-altered diamictites may contain minor ankerite, but generally lack calcite and dolomite. Very strongly muscovite altered diamictites are spatially associated with areas of copper mineralisation.
• Brown-colored, biotite-rich matrix diamictite, which preferentially occurs in the uppermost section of the Grand Conglomérat Formation. Aggregates of biotite are concentrated around clasts, and are locally intergrown with and replace K feldspar. Biotite may contain 10 to 50 µm subhedral to anhedral muscovite inclusions and albite as well-rounded apatite grains that are rimmed or partially replaced by calcite or dolomite/ankerite.
• Greenish chlorite-rich diamictite is most abundant adjacent to the inferred normal faults, but is present throughout the Grand Conglomérat as well as in copper-sulphide mineralised sections of the Kakontwe Limestone. The chlorite is not oriented, and occurs as 20 to 200 µm anhedral grains replacing both muscovite and biotite. Where replacing biotite, it also forms 100 to 200 µm rims on diamictite clasts. Chlorite rich diamictite commonly carries 20 to 50 µm anhedral crystals of ankerite. Copper sulphide minerals are commonly intergrown with chlorite, implying chloritisation was temporally related to the dominant period of sulphide mineralisation.
Biotite and chlorite are commonly found rimming diamictite clasts at Fishtie, although muscovite rims are uncommon. These rims may also contain K feldspar, ankerite, quartz and copper sulphides, and are thickest and most abundant within and proximal to sulphide-rich zones. They are sometimes developed at opposite ends of individual clasts, with the appearance of pressure shadows, similar to those described at the Kamoa deposit by Schmandt et al. (2013).
Sulphide mineralisation
The Nguba Group rocks in the Fishtie district contain variable pyrite, pyrrhotite and copper sulphides. Within the immediate vicinity of the ore deposit, the Grand Conglomérat Formation contains minor, always <1%, fine-grained (<50 µm) pyrite, with a subrounded morphology, suggestive of a framboidal habit. Minor, similarly shaped pyrite occurs within dolomitic siltstones of the Kakontwe Limestone. Beyond the deposit, the Grand Conglomérat contains minor disseminated pyrrhotite, typical of the unit throughout the Central African Copperbelt, probably due to modification of primary pyrite during Lufilian metamorphism (Hendrickson et al., 2015).
The Fishtie deposit comprises a basic suite of copper sulphide minerals, predominantly chalcopyrite, accompanied by bornite in higher-grade zones. Bornite is only locally the dominant sulphide. Late stage, coarse-grained, generally subhedral pyrite is found on the fringes of the chalcopyrite zone. The mineralised system does not contain significant Ag, Pb, or Zn, although locally there is anomalous cobalt, with carrollite only observed within the Kakontwe Limestone (Hendrickson et al., 2015).
Copper sulphides occur throughout the section, from the basement metamorphic rocks to the highest preserved levels of the Kakontwe Limestone Formation (Hendrickson et al., 2015).
Only sparse sulphide minerals are found in basement rocks, where fine to coarse grains of pyrite and lesser pyrrhotite are intergrown with coarse-grained muscovite. Copper sulphides, dominantly chalcopyrite, are primarily present as coarse-grained disseminations in quartz-muscovite schist and in quartz-sulphide veins cutting schist and quartzite. Within the schist, the copper sulphides are generally intergrown with coarse-grained, unfoliated aggregates of either muscovite and/or chlorite (Hendrickson et al., 2015).
The distribution and amount of copper sulphides is controlled by both proximity to normal growth faults, as is the earlier magnetite mineralisation detailed above, and by distinct stratigraphic units.
Within Grand Conglomérat diamictites, hypogene copper sulphides are most abundant in the central and the satellite structural domains, immediately adjacent to inferred normal faults, occurring near the contact with the overlying Kakontwe Limestone, and within dolomitic siltstone layers of the latter. Sulphide mineralisation within the Grand Conglomérat in the central domain is focused along the northern of the two normal faults, restricted to within 50 m on either side of the inferred fault plane. Less intense mineralisation also occurs along the southern normal fault that controlled the location of earlier magnetite-ankerite-quartz alteration. Sulphide mineralisation in the Kakontwe Limestone is also focused along and adjacent to these same inferred normal faults. In the satellite structural zone, sulphide mineralisation occurs primarily at the base of and within the Kakontwe Limestone rather than in the Grand Conglomérat. Sulphides in this zone persist for at least 100 m laterally from the inferred normal fault, although the distribution of copper sulphide mineralised zones suggests a normal fault north of the main drilled area may have been the primary conduit for hydrothermal fluids (Hendrickson et al., 2015).
Within the Grand Conglomérat, sulphides are more abundant in diamictites than within siltstone interbeds, occurring as <50 µm, anhedral grains, and as coarse-grained crystals on clast margins. Copper sulphides overgrow magnetite in both the magnetite-ankerite-quartz zones and within the Grand Conglomérat matrix, commonly with textures indicating co-precipitation, although some bornite is seen to replace chalcopyrite, and some chalcopyrite apparently replaces pyrite. Rarely, quartz-carbonate-(sulphide) veins up to 15 cm wide are observed (Hendrickson et al., 2015). There is a mineralogical zonation of the copper sulphides within the Grand Conglomérat. A bornite-rich assemblage at the base of the Grand Conglomérat unit in the central structural domain, occurs adjacent to the normal fault, grading stratigraphically upwards to a more chalcopyrite-rich assemblage. Bornite reappears within the Grand Conglomérat unit, below the contact with the overlying Kakontwe Limestone. There is also a poorly defined lateral zonation, from a more bornite-rich assemblage adjacent to the northernmost normal fault to chalcopyrite- and, then a distal, pyrite-rich assemblage both to the north and south of the fault (Hendrickson et al., 2015).
Within the Kakontwe Limestone, copper sulphides are preferentially found in highly carbonaceous dolomitic siltstone beds, occurring as disseminated grains along bedding planes. Bedding parallel and subvertical quartz-dolomite-sulphide veins, dominantly chalcopyrite with lesser bornite, pyrite and locally carrollite, are also found in the Kakontwe Limestone in the satellite structural domain. There is a similar vertical and lateral zonation of sulphide minerals in the satellite structural domain of the Kakontwe Limestone to that found in the Grand Conglomérat (Hendrickson et al., 2015).
Two main pulses of sulphide mineralisation are interpreted at Fishtie. The first is an early diagenetic pyrite that is found in the siltstone units, and to a lesser extent, in the diamictite matrix of the Grand Conglomérat. This was followed by the iron-oxide mineralisation and alteration, which, in turn, was overprinted by copper sulphide and ore stage pyrite mineralisation, which affected both the Grand Conglomérat and Kakontwe Limestone. The copper sulphides are most abundant in association with chlorite-rich alteration, and less so in biotite- and muscovite-rich assemblages, implying that the bulk of the copper sulphide mineralisation was coeval with chloritisation (Hendrickson et al., 2015).
The resultant ore deposits do not form concordant sheets, but are transgressive and linear, straddling the main normal growth faults. Each has a 'Christmas-tree' like cross section, widest at the base, immediately above the basement contact, where mineralisation extends up to 50 m from the fault, tapering upwards, before widening again towards the top of the Grand Conglomérat and into the Kakontwe Limestones where mineralisation may extend 100 m from the fault. The ore zone encloses a similar 'Christmas-tree' shaped core of bornite-rich mineralisation.
The isotopic values of diagenetic and hydrothermal sulphides at Fishtie are spread over a relatively wide range, which Hendrickson (2013) interprets to indicate there was no significant isotopic homogenisation in the Fishtie deposit during the ~590 to 500 Ma Lufilian metamorphic event.
Fine-grained, disseminated diagenetic pyrite within Grand Conglomérat siltstones, both within and distal to the ore zone, yield δ34S values clustered around +11‰ (Hendrickson, 2013), overlapping normal Neoproterozoic sedimentary pyrite (Strauss 1997). In contrast, δ34S values for diagenetic pyrite from the Zambian Copperbelt to the north, is commonly lighter than 0‰ (McGowan et al.2003), whilst framboidal diagenetic pyrite from Grand Conglomérat siltstone layers at the Kamoa deposit in the DRC, has values of -11 to +3‰ (Schmandt et al., 2013). Hendrickson (2013) interpret the relatively heavy values at Fishtie to possibly reflect ore stage pyrite replacement of the diagenetic precursor. The ore-stage pyrite and chalcopyrite is coarse-grained and has relatively heavy sulphur isotopic values. In the central structural domain, both coarse-grained clast rimming, and fine-grained disseminated chalcopyrite have δ34S values ranging from from 7 to 13‰, averaging 9‰. Similar chalcopyrite in the satellite structural domain displays values ranging from 10 to 11‰, whilst bornite and chalcopyrite in quartz-carbonate veins hosted by the Grand Conglomérat have values that range from 3 to 6‰, averaging 4‰ (Hendrickson, 2013).
Sulphides hosted by the Kakontwe Limestone have heavier δ34S values than those within the Grand Conglomérat. In the satellite structural domain, ore-stage cubic pyrite from dolomitic siltstones of the Kakontwe Limestone has δ34S values ranging from 15 to 26‰, averaging 20‰. In the same part of the deposit, chalcopyrite has values between 14 and 25‰, averaging 19‰, whilst chalcopyrite in quartz-carbonate veins has slightly lighter values that range from 13 to 19‰, averaging 16‰.
Similar trends indicating heavier sulphur isotopic values up section have been noted at a number of Zambian Copperbelt deposits to the north (Selley et al., 2005) and in the Kamoa deposit (Schmandt et al., 2013). Hendrickson et al. (2015) note that the reasons for this trend is unclear, but suggest it may reflect increased incorporation of sulphur derived from Neoproterozoic seawater sulphate upwards in the stratigraphic sequence.
At the onset of the Neoproterozoic Sturtian glaciation, the δ34S sulphur isotopic composition of seawater is estimated to have been between 15 and 25‰ difference from the Canyon Diablo Troilite (CDT; Claypool et al., 1980; Strauss et al., 2001). Throughout the Central African Copperbelt, anhydrite from the Roan Group has δ34S values averaging ~17‰ (Selley et al., 2005), whilst sulphate within the Mwashya Subgroup immediately below the Grand Conglomérat at Kamoa averages 20‰ (Schmandt et al., 2013). Hendrickson et al. (2015) conclude the isotopic values of copper sulphides and coeval pyrite at Fishtie suggests reduced sulphur was not derived from diagenetic sulphides as has been suggested for the majority of sulphides at Kamoa (Schmandt et al., 2013) but must have been derived by reduction of seawater sulphate.
Supergene mineralisation
The Fishtie deposit contains significant quantities of brown to reddish, fine-grained to almost conglomeratic saprolite composed of limonite-goethite, clay, copper oxide and carbonate minerals (malachite >> azurite) and chalcocite, at grades of that may exceed 4.5 % Cu over 1 m intervals. The bulk of the supergene mineralisation occur along the contact between the Grand Conglomérat and the Kakontwe Limestone formations within the central structural domain where it forms a 1 to 30 m thick zone that is ~450 m long (east-west) and 125 m wide (north-south). The mineralisation is predominantly in weathered dolostone that comprises a saprolite to residual collapse breccia containing relict clasts of saprolitised carbonaceous dolomitic siltstone with pseudomorphs after Fe and Cu sulphides. This oxidation extends down into the sulphide zone within the Grand Conglomérat along the normal faults. However, elsewhere, the top siiltstone of the Grand Conglomérat forms an impermeable footwall to development of the oxide mineralisation.
There is a lateral zonation across the oxide zone from north to south, from the northern or up dip extremity, where mixed refractory and acid soluble copper has a grade of <0.5% Cu. This passes south into a zone of 0.5 to 2% Cu as black neoticite copper in dolomite residuum. Then to high grade acid soluble copper, extending down into the main normal fault zone where it occurs as variable mixed oxide-sulphide ore to depth. South from this zone is 1 to 5% acid soluble copper in residual and collapse breccia carbonate sap-rocks, and on the southern margin a zone of high grade oxide ore at the transition to a dolomite karst surface, mainly containing malachite and chrysocolla with black oxide neoticite.
Estimated sulphide, mixed oxide-sulphide and oxide mineral resources (Hanssen 2008) were:
~55 Mt @ 1.04 % Cu.
This summary has largely been drawn from: "Hendrickson, M.D., 2013 - Geology of the Fishtie copper deposit, Central Province, Zambia; Unpublished M.S. thesis, Colorado School of Mines, Golden, CO., 148p." and Hendrickson et al., 2015 as cited below.
The most recent source geological information used to prepare this decription was dated: 2015.
This description is a summary from published sources, the chief of which are listed below. © Copyright Porter GeoConsultancy Pty Ltd. Unauthorised copying, reproduction, storage or dissemination prohibited.
Fishtie
|
|
Hendrickson, M.D., Hitzman, M.W., Wood, D., Humphrey, J.D. and Wendlandt, R.F., 2015 - Geology of the Fishtie deposit, Central Province, Zambia: iron oxide and copper mineralization in Nguba Group metasedimentary rocks: in Mineralium Deposita v.50 pp. 717-737
|
Porter GeoConsultancy Pty Ltd (PorterGeo) provides access to this database at no charge. It is largely based on scientific papers and reports in the public domain, and was current when the sources consulted were published. While PorterGeo endeavour to ensure the information was accurate at the time of compilation and subsequent updating, PorterGeo, its employees and servants: i). do not warrant, or make any representation regarding the use, or results of the use of the information contained herein as to its correctness, accuracy, currency, or otherwise; and ii). expressly disclaim all liability or responsibility to any person using the information or conclusions contained herein.
|
Top | Search Again | PGC Home | Terms & Conditions
|
|