Central African Copperbelt - Zambian Copper Belt |
|
Zambia |
Main commodities:
Cu Co
|
|
 |
|
 |
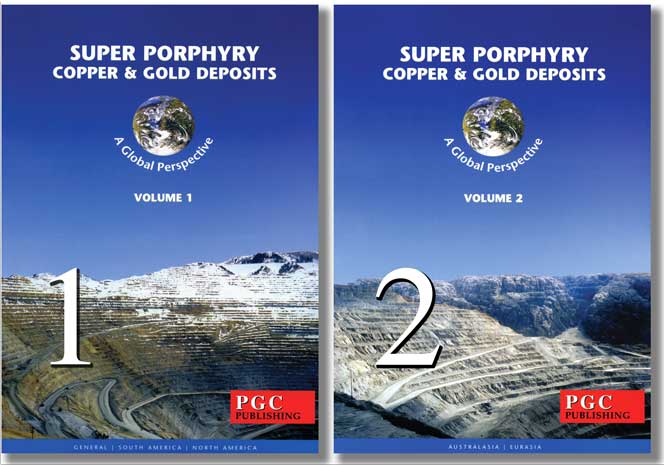 |
Super Porphyry Cu and Au

|
IOCG Deposits - 70 papers
|
All papers now Open Access.
Available as Full Text for direct download or on request. |
|
 |
Summary
The Zambian Copperbelt is part of the larger Central African Copperbelt, and extends north into the neighbouring Democratic Republic of Congo (DRC), to continue as the Congolese or Katangan Copperbelt. It closely coincides with the southern half of a complex, arcuate structural zone, the late Neoproterozoic to early Cambrian Lufilian Arc. Although part of the same curvilinear trend, the Zambian Copperbelt deposits, are hosted by non-evaporitic, rift facies siliciclastic rocks that are not temporally or lithologically equivalent to the mainly carbonate hosts of the Congolese Copperbelt. In addition, the structural architecture in Zambia is characterised by thrusted Palaeo- to Mesoproterozoic metamorphic basement inliers and folded Neoproterozoic sedimentary host rocks, in contrast to the thin skinned deformation of the hosts in the DRC, without involvement of basement.
Mineralisation within the Central African Copperbelt is hosted within the Neoproterozoic Katanga Supergroup, an intracontinental rift basin sequence, comprising the Roan, Nguba and Kundelungu groups. A period of extension commenced after ~900 Ma, and in Zambia, the sedimentary sequence began after 880 Ma, with the Lower Roan Subgroup oxidised rift facies clastic rocks, deposited in a series of restricted sub-basins controlled by extensional normal faults, including the thin reduced argillites of the Copperbelt Orebody Member near the middle of the unit. The succeeding sag phase Upper Roan Subgroup, is dominantly made up platformal mixed carbonate and clastic rocks, but also included a <500 m thick evaporite/salt bed. To the north, in the DRC, the sag phase sequence transgresses beyond the main rift margin, to become the basal unit of the Roan Group, where the Upper Roan Subgroup equivalents are divided into three. The lowest of these, the R.A.T. Subgroup, comprises the red, silt- and sand-sized residue after dissolution of a thick (>500 m) evaporite/salt bed deposited in a restricted sub-basin, separated from the open sea by a stromatolitic reef. This is overlain by the Mines Subgroup dolostones and shales, including a number of reduced units near the base. The uppermost of the three Roan Group units in the DRC is the Dipeta Subgroup, comprising a lower regressive suite, including oxidised, evaporitic rocks, followed by transgressive carbonate rocks with some reduced intervals.
During deposition of the late Upper Roan and Dipeta subgroups, a period of 'renewed extension' commenced, reflected by a ~30 to 100 km wide by ~500 km long corridor of gabbroic and lesser felsic intrusions, and an associated volcanic belt of thin mafic lavas and tuffs emplaced from ~765 to 735 Ma. During the same event, an arcuate metamorphic core complex formed, embracing the gabbroic intrusive corridor, marked by a series of basement domes capped by mylonitic detachments. During this period, deposition transgressed further onto basement, and the Dipeta Group passed upwards into a sequence of carbonaceous argillites of the Mwashya Subgroup, the uppermost unit of the Roan Group in both Zambia and DRC. This was overlain by glacial diamictites and interbedded pyritic black argillites of the basal Nguba Group Grand Conglomérat. The remainder of the Nguba Group carbonate and siliciclastic rocks were overlain in turn by the Kundelungu Group basal diamictites and then carbonate rocks, passing up into siliciclastic rocks.
The main Katanga Supergroup deposition was terminated by the Lufilian orogenic event after ~600 Ma, with NE vergent D1 folding and thrusting and D2 strike-slip folding to produce the Lufilian Arc. Prior to this event, possibly during late 'renewed extension', uplift of the Domes core complex to the SW, coupled with deepening of the basin to the NE (in response to extension), and dissolution of the R.A.T. Subgroup salt beds (as the increasing temperature and pressure allowed fluids to penetrate the salt beds), led to northward mass gravity gliding of the Upper Roan/R.A.T., Mines and Dipeta subgroup successions towards the main basin centre. This gravity gliding, slumping, salt dissolution and diapirism, produced a regional scale megabreccia of large clasts up to several km in length of Mines Subgroup rock in a matrix of R.A.T. Subgroup and comminuted Roan Group rock fragments to rock flour.
During the late Lufilian event, a complex of anorogenic granites was emplaced in the inner Lufilian Arc to the SW from ~560 to 530 Ma, with minor coeval mafic to ultramafic intrusions. This was followed after ~530 Ma by orogenic relaxation that lasted until after 500 Ma.
Stratabound and vein ore emplaced after 880 Ma is found in rocks from the Palaeoproterozoic Lufubu Metamorphic Complex to the Kundelungu Group. However, large, stratabound sediment hosted Cu-Cu±U deposits are restricted to the stratigraphic interval from the Lower Roan Subgroup to the basal Nguba Group, specifically where it occurs in association with rocks that contain (or contained) in situ or mobile hydrocarbons at the following stratigraphic levels:
i). the Copperbelt Orebody Member (including secondary positions within 100 m above and below this member), where the great bulk of the Zambian Copperbelt deposits are hosted (e.g., Luanshya-Baluba, Mufulira, Nkana-Mindola, Nchanga-Chingola, Chambishi and Konkola-Lubambe);
ii). the base of the Mines Subgroup (including a subsidiary position within ~100 m higher in the sequence) in the Congolese Copperbelt, accounting for the bulk of ore in the DRC (e.g., Kolwezi Klippe, Tenke Fungurume, Kinsevere, Kambove, Etoile, Ruashi, Luiswishi, Luishia and Kakanda);
iii). the upper Dipeta Subgroup (e.g., Mutanda, Deziwa;
iv). Mwashya Subgroup (e.g., Frontier, Kansanshi); and
v). Grand Conglomérat of the Nguba Group (e.g., Kamoa, Fishtie).
However, almost invariably, ore is only found at the first reduced unit overlying an oxidised arenaceous (or otherwise permeable) sequence, separating it from basement, e.g., ore is only found within the Mwashia Subgroup or Grand Conglomérat where no reduced Roan Group unit exists in the underlying sequence (e.g., Frontier, Kamoa).
Regional scale alteration includes an early i). calcic-magnesian phase that redistributed carbonate and anhydrite from the evaporite beds to porous rocks throughout the basin; ii). intense potassic alteration that overlapped the Ca-Mg stage, and preceded and overlapped ore; and iii). a later, but multipulse sodic-calcic phase that pervasively overprinted early ore and predominantly affected Nguba and Kundelungu group rocks, but also locally Roan Group lithologies.
Sulphide mineralisation was emplaced in a number of generations, including: i). early pyrite, developed either during early diagenesis in association with bacterial sulphate reduction (BSR) in carbonaceous argillites, or late diagenesis after emplacement of oil or gas reservoirs. ii). early diagenetic pyrite was overprinted and partially replaced by weak regional copper mineralisation derived from diagenetic, saline K-Mg brines circulating and scavenging metal from the basin; iii). significant, late diagenetic and pre-folding, generally fine-grained, disseminations (mostly bedding controlled) and veins containing Cu-Co sulphides and pyrite, concentrated in fluid traps along basement growth faults. These sulphides usually have isotopic signatures suggesting low temperature BSR associated with brines at temperatures of from 80 to 150°C; iv). early tectonic syn-folding sulphides, occurring as coarser veins and lesser disseminations of Cu-Co sulphides associated with brines at temperatures of 150 to 250°C that are replacing earlier, and generating new, sulphides by thermochemical sulphate reduction (TSR); v). post-folding, coarser veins and lesser disseminations of copper sulphides, that formed at the end the main Lufilian Orogeny during orogenic extensional relaxation. This generation was associated with calcic-sodic brines at temperatures of 250 to 400°C, replacing existing sulphide and producing ones by TSR, concentrated in reduced rocks, including those with pre-existing mineralisation. These brines were also responsible for IOCG and hybrid IOCG-sediment hosted copper mineralisation.
Most significant sediment hosted Cu-Co deposits in the Central African Copperbelt can be shown to contain early pyrite, overprinting pre-folding, early to late diagenetic Cu-Co sulphides in disseminations and veins, and syn- to post-folding coarser veins and lesser disseminations. From the evidence available it can be inferred that significant Cu-Co mineralisation was emplaced by a major influx of metalliferous brines scavenged from both the basement and Katanga basin during: i). the renewed extension coeval with or soon after deposition of the Grand Conglomérat of the Nguba Group, at ~765 Ma; ii). during the early stages of the Lufilian compression from ~585 to 575 Ma; and iii). during orogenic relaxation from ~530 to ~500 Ma. Not all deposits have experience all three generations (e.g., Kansanshi), although most of the large high grade deposits are the product of at least two.
The Central African Copperbelt is the largest and highest grade sediment-hosted stratabound copper province known in the world. Mineralisation is hosted in a variety of rocks and fluid traps, and emplaced by a number of events spread over a period of ~200 Ma. The key however, is the large amount of Cu-Co, necessarily scavenged from a very large volume of rock with lithologic and structural permeability. The structural preparation of basement permeability, and the generation and circulation of brines required both a large scale source of salts/evaporites, and significant pulses of energy to promote basement faulting (both listric and compressional detachments) and to elevate thermal gradients. These energy pulses were provided by the 'renewed extensional' event, particularly the metamorphic core complex and gabbroic magmatism, and the Lufilian compression and related anatectic magmatism. Without one or both of these two events, the result may have been a regionally distributed low grade to anomalous copper shale.
Regional Setting
The >500 km long Lufilian Arc is a structural element imposed upon the rocks of the Katanga fold belt in western Zambia and southern DRC during the ~590 to 510 Ma Lufilian Orogeny. It substantially reactivated and reflects earlier syn-depositional structures, and extends from the edge of the Archaean Congo/Kasai craton in the NW, to the major trans-continental WSW-ENE trending, sinistral, Mwembeshi Shear Zone to the SE, separating it from the Zambezi Belt to the south. The Zambezi Belt trends east-west, normal to the Lufilian Arc, and is a zone of shearing and metamorphism that rims the northeastern margins of the Kalahari craton to the south. Porada and Berhorst (2000) suggest the Mwembeshi Shear Zone marks a suture between the predecessors of the Kalahari and Angolan/Congo cratons that were welded during the Ubendian-Eburnian cycle in the Palaeoproterozoic, and has focussed subsequent tectonic activity from the Palaeo- to late Neoproterozoic. The Mwembeshi Shear Zone, which comprises multiple strands over a width of kilometres, has been interpreted to continue as the Matchless Belt in the Damaran in Namibia to the SW (e.g., Hitzman et al., 2012).
The Kalahari and Congo cratons are composites of earlier Archaean nuclei (e.g., the Kaapvaal and Zimbabwe cratons within the former) and Palaeo- to Mesoproterozoic elements that were amalgamated and cratonised by ~1.05 Ga as part of the Rodinia supercontinent.
Magnetic data and deep drilling below cover, suggest the structural trend of the Lufilian Arc curves to the SW and south from its NW margin along the Congo Craton to the vicinity of the Mwembeshi Shear Zone in the SW in NW Botswana (Hitzman et al., 2012; De Wit, 2009).
The oldest orogenic basement rocks between the cratons are exposed as inliers within, and marginal to the Lufilian Arc, particularly in the core of the Zambian Copperbelt and the Bangweulu Block to the NE. They dominantly comprise a Palaeoproterozoic, Eburnian-Ubendian (~2.05 to 1.82 Ga) calc-alkaline magmatic arc sequence of metasedimentary, metavolcanic and intrusive granitoid rocks of the Lufubu Metamorphic Complex (Rainaud et al., 2005; Selley et al., 2005). These rocks are interpreted to be part of a broader, regionally extensive, ENE-WSW Palaeoproterozoic magmatic arc terrane, paralleling the Mwembeshi Shear Zone, stretching from northern Namibia to northern Zambia, between the Kalahari and Angola/Congo craton, then swinging to the NNE-SSW to NNW-SSE, in northern Zambia and the DRC, where it separates the Congo and Tanzanian cratons (Rainaud et al., 2005; Porada and Berhorst, 2000; Petters, 1986). The Neoproterozoic Lufilian Arc straddles the confluence of these two structural trends. In the east and below the Katanga sequence, this basement includes a reworked Neoarchaean component (2.73 Ga; in the Bangweulu Block only), a Palaeoproterozoic granitic phase (2.05 to 1.93 Ga) and a Palaeoproterozoic plutono-volcanic magmatic arc complex (1.87 to 1.82 Ga; De Waele and Fitzsimons, 2007 and sources cited therein). This orogenic terrane is inferred to have been related to the Ubendian-Eburnian collision mentioned previously, and was accreted onto the southern and eastern margins of the Congo Craton during the ~1.4 to 1.0 Kibaran orogeny, represented by a broad SW-NE trending string of 'Eburnian basement inliers' (Porada and Berhorst, 2000).
To the south of the Zambezi belt, a partially coeval sequence of 2.16 to 2.10 Ga continental to shelf clastic and lesser carbonate sedimentary rocks, with a mafic volcanic component, the Magondi Supergroup, was deposited over the margins of the Archaean Zimbabwe craton (Master,1991). This sequence hosts the Magondi Copperbelt deposits in Zimbabwe, ~400 km south of the Zambian Copperbelt (see the separate Magondi Copperbelt record).
The Archaean to early Palaeoproterozoic basement rocks of the Congo and Kalahari craton are separated from the Neoproterozoic sequence of the Katanga Supergroup by the Mesoproterozoic Kibaran and Palaeo- to Mesoproterozoic Irumide successions respectively. These rocks represent the extensive, generally northeast-trending, Kibaran and Irumide mobile belts, which were precursor rift basins to the Neoproterozoic Katangan sequence, bifurcated and separated by the Bangweulu Block and Tanzanian Craton.
The ENE-WSW elongated Irumide belt stretches over a distance of approximately 900 km from central Zambia to the Zambia-Tanzania border and northern Malawi, bounded to the NW by the Archaean to Palaeoproterozoic basement lithologies of the Bangweulu block, and truncated to the NE by Mesoproterozoic and Neoproterozoic transcurrent shear zones within reactivated parts of the Palaeoproterozoic Ubendian belt. To the SE and south, Irumide rocks were reworked within the Neoproterozoic Lufilian and Zambezi belts, and to the east by the Mozambique Belt.
The Irumide sequence comprises a thick (>8 km) sequence of variously metamorphosed conglomerates, sandstones and shales with lesser dolerite flows, and thin overlying graphitic shales, evaporites, stromatolitic limestones and dolostones. It occurs as a condensed succession over the Bangweulu Block, passing into a thick marine sequence to the south. Metamorphic grades range from greenschist facies in the northwestern foreland overlying the Bangweulu Block, to upper amphibolite facies in the southeast, with local granulites (De Waele, 2004). Dating reported by De Waele (2004) and De Waele and Fitzsimons (2007) suggest the Irumide rocks were deposited in two episodes, the first, an extensive quartzite-metapelite succession with minor carbonate (Mporokoso, Kanona and Manshya River groups), deposited in the Palaeoproterozoic at ~1.8 Ga (from interlayered 1815 Ma volcanic rocks), and a second, more restricted sequence which consists largely of very mature, fluvial sandstones (Kasama Formation) at ~1.4 Ga that recycled sediments from the first episode rocks (De Waele, 2004). Peak metamorphism in the Irumide sequence was at 1.05 to 1.02 Ga (Rainaud et al., 2005). Voluminous syn- to post-kinematic Irumide granitoids were emplaced between 1.05 and 0.95 Ga. In addition, a minor suite of 1.36 to 1.33 Ga anorogenic plutons (nepheline syenite and biotite granite) have been identified in the far northeastern Irumide belt. Geochemical data indicate uniform crust-dominated patterns and the significant participation of older crust in the generation of all of these magmatic suites (De Waele, 2004).
Within the Zambian Copperbelt, Lufubu Metamorphic Complex rocks are unconformably overlain by a supracrustal metasedimentary sequence of conglomerates, orthoquartzites and metapelites, the Muva Group that have been correlated with the Irumide sequence, but assumed to be ~1.3 to 1.05 Ga (Selley et al., 2005), but may be older.
To the NW of the Katangan rocks, the >10 km thick, 1.4 to 1.05 Ga Kibaran sequence which is broadly coeval with the second Irumide pulse. It can be subdivided into four lithostratigraphic units (Laghmouch et al., 2012), from the base: i). Kiaora Group - dominantly phyllites and schists with quartzite horizons, and rhyolites at the top and intracontinental, rift related bimodal intrusions dates at between 1380 and 1370 Ma (Tack et al, 2010); ii). Nzilo group - predominantly quartzite, with local (quartzo-)phyllitic interbeds; iii). Mount Hakansson Group - dark slates and quartzites, with basal conglomerates; iv). Lubudi Group - dark arkoses with conglomerate lenses, black graphitic shale, some sandstone, and an upper limestones and dolostone unit, often with silicified stromatolites.
Two main styles of granitoid intrusion are recognised in the Kibaran Belt, syn- and post-orogenic phases at ~1.3 to 1.25 and 1.2 to 0.95 Ga respectively. The syn-orogenic gneissic to un-metamorphosed granitoids accompanied compression and basin inversion, while those of post-orogenic origin are alkaline and include the Kibaran tin granites (Petters, 1986 and sources quoted therein).
The Neoproterozoic Katanga Supergroup of the Katanga fold belt separates, and laps onto, both the Kalahari and Congo Mesoproterozoic cratons of southern and central Africa respectively (which by now incorporated the Kibaran and Irumide belts). It is one of a number of similar Neoproterozoic Pan-African fold belt sequences (including the Damaran, Kaoko and West Congo) that fringe and/or separate these two cratons, and which may be interconnected below intervening Phanerozoic cover.
In most of the Pan-African mobile belts of southern and central Africa, a ~200 m.y. period of tectonic quiescence followed the assembly of Rodinia, before extension and Neoproterozoic sedimentation heralded the commencement of its breakup. The exception may be the southern margin of the Damaran fold belt, where the uplift along the Rehoboth and Irumide zones, associated with the latter stages of the Kibaran event, resulted in mafic volcanism and coarse cratonic sedimentation of the 1100 to 950 Ma Dordabis (or Tsumis) Group, which hosts mineralisation in the Kalahari Copperbelt (see the Kalahari Copperbelt in Namibia and Botswana records).
In the Zambian section of the Lufilian Arc, the resultant 4 to 10 km thick, Katanga Supergroup (~880 to ~530 Ma) comprises an initial sequence of coarse grained, fluvial conglomerates and sandstones, dominantly siliciclastics, devoid of volcanic activity, deposited within relatively restricted, fault controlled, NW-SE elongated intracontinental rift basins, occurring as horsts and half grabens. These rift basins were the result of NE-SW directed extension, parallel to the major regional Mwembeshi Shear Zone, and flanked a NW-SE trending basement high, now reflected by the younger Kafue Anticline.
The Katanga Supergroup commences with the Mindola Clastics Formation, a variable sequence from 0 to 1300 m thick, most commonly up to ~150 to 240 m, composed of an oxidised suite of interbedded conglomerates, arenites, quartzites and lesser argillites. At the main deposits of the Zambian Copperbelt, it represents the footwall sequence, and contains basal conglomerate that varies from thin developments a few tens of metres, up to ~240 to 300 m thick, to near 1200 m on the northern margin of the Kafue Anticline, east of Konkola and Nchanga (although the latter may in part be due to structural repetition). This conglomerate is overlain by an arenite/quartzite unit, with rare argillites, and some conglomerate interbeds, particularly near the upper transitional contact, that similarly varies from a few tens of metres to as much as 300 m in thickness. These are followed by another conglomerate that is a few to a few tens of metres thick, then a further arenite/quartzite of similar thickness, and a final footwall conglomerate that is only a few metres thick. Locally at Nchanga, the upper two conglomerates and intervening arenite are absent. On the eastern margin of the Kafue Anticline, the Mindola Clastics Formation equivalent primarily comprises a 0 to 150 m thick sequence, commencing with a thin basal conglomerate, overlain by a succession of quartzite and grits.
These depositional centres were subsequently linked along master faults, marking a transgression, reflected by the deposition of local finer facies of the Copperbelt Orebody Member, the basal unit of the Kitwe Formation. The Copperbelt Orebody Member (or "Ore Shale") represents the culmination of early rift-stage extension and the first marine incursion within the Katanga basin in the Zambian Copperbelt. It comprises coarse and fine siliciclastic sedimentary rocks, representing fluctuating emergent and subaqueous conditions. The unit varies from 0 to as much as 60 m, more commonly 15 to 35 m thick, and frequently commences with a basal band of dolomitic schist, representing a décollement. It comprises carbonaceous and/or dolomitic siltstones, argillites, thin dolostones and fine sandstones, and hosts the bulk of the ore in the main large deposits of the Zambian Copperbelt (e.g., Luanshya, Nkana, Mindola, Chambishi and Konkola), although a number of smaller deposits (e.g., Chibuluma, Fitula and Mimbula) are hosted by the Mindola Clastics Formation. To the east of the Kafue anticline (e.g., at Mufulira), the probable stratigraphically equivalent unit of the Copperbelt Orebody Member is the 1 to 2 m thick dolomitic bed known as the "Mudseam" (Binda, 1994).
The remainder of the Kitwe Formation varies from <100 to >300 m in thickness, and at most localities commences with a quartzite to arkose unit, which may be locally absent (e.g., Nkana). It is followed by a finer unit that varies from argillite to dolomitic argillite or sandstone, to dolostone and dolomitic schist, that varies from a few to 160 m thick, culminating in a generally 10 to 30 m dolostone band. These are succeeded by a thick (up to 160 m) of feldspathic and dolomitic sandstones, interbedded with coarse grained calcareous sandstones to grit. At Nchanga, the ore extends upward into the sequence above the Copperbelt Orebody Member, with much of the ore within feldspathic quartzite of the "hanging wall", capped by a shale interbed.
The Mindola Clastics and Kitwe Formations, together, comprise the Lower Roan Subgroup. The upper two units of the Kitwe Formation (dolostone and calcareous sandstones to grit) were included into the Upper Roan Subgroup by earlier authors (e.g., Mendelsohn, 1961), although the Lower to Upper Roan Subgroup boundary has been shifted to the base of the Upper Roan Dolomite unit in more recent papers (e.g., Binda and Mulgrew, 1974).
Significant authigenic anhydrite is found in the Lower Roan Subgroup, in particular within the upper Mindola Clastics and lower Kitwe formations. Narrow evaporite units are also indicated by local breccias and porous units in both formations (e.g., within the Basal Quartzite at Nkana, and the Hanging wall Aquifer at Kirila Bombwe/Konkola).
See the separate Luanshya, Mufulira, Nkana-Mindola, Chambishi, Nchanga-Chingola and Konkola-Lubambe records for the detailed stratigraphy through the Zambian Copperbelt.
The succeeding post-rift, thermal sag phase, produced the laterally more extensive platformal sequence of the Upper Roan Subgroup, comprising laterally extensive mixed shallow marine to lagoonal carbonate rocks and generally finer grained siliciclastic rocks with abundant evaporitic textures and mainly stratabound chaotic breccia (interpreted to represent the dissolution of evaporites). The sequence varies considerably in thickness, from <30 to 800 m, and is characterised by metre-scale, laterally extensive cycles of upward-fining, sandstone, siltstone, dolomite, algal dolomite and local anhydrite. Thickness variations can be abrupt and commonly are associated with breccias. The breccias are usually composed of rounded to angular, mm to metre sized polylithic intraformational clasts, set in a crystalline matrix of carbonate, albite, quartz, anhydrite and/or magnesian chlorite. The breccias may be single cm to metre thick bands, to stacked complexes, up to several hundred metres thick. In general they are stratabound, but on a larger scale, step down through the sequence to the south and west (Selley et al., 2005).
The Upper Roan Subgroup is alternatively known as the 'Bancroft' or 'Bancroft Kanwangungu' Formation by some authors (e.g., Cailteux et al., 2007; Zientek et al., 2014), whilst an alternative amalgamation of the Upper Roan Subgroup to also include the carbonate and clastic units of the upper Kitwe Formation (i.e., the shales with grit of the Antelope Clastics, also known as the Kibalongo Formation by Cailteux et al., 2007), has been referred to as the Kirilabombwe Subgroup (e.g., Cailteux et al., 2007; Zientek et al., 2014). Cailteux et al. (1994) equates the Upper Roan Subgroup with the Dipeta Subgroup in the DRC.
The Upper Roan Subgroup is interpreted to pass from mixed shallow marine and lagoonal evaporitic facies adjacent to the 'Kafue Anticline' and in the Congolese Copperbelt, to a deeper marine shelf environment to the west and SW (Porada and Berhorst, 2000), but still includes shallower, evaporitic lagoonal sections in the western Domes Region (Gregory et al., 2012).
The Upper Roan Subgroup in Zambia is overlain across either a conformable transition, or an evaporite-facilitated breccia-detachment, by the 150 to 650 m thick Mwashya (or Mwashia) Subgroup, emplaced within a deepening marine setting. This sequence commences with a lower suite of reefal to intertidal clastic carbonate rocks, mainly arenitic dolostone with lesser argillaceous dolostone containing pseudomorphs after anhydrite nodules. The lower Mwashya is only poorly developed in Zambia, but in the DRC, the thicker equivalent, the Kansuki Formation, is included as the uppermost member of the Dipeta Subgroup, (Cailteux et al., 2007). The Dipeta Subgroup is equivalent to the upper sections of the Upper Roan Subgroup in Zambia (Cailteux et al., 2005; 2007; Hitzman et al., 2012). The first of a series of intrusive rocks and thin (up to 50 m) mafic lavas and tuffs with interbedded ironstone lenses are encountered in the Kansuki Formation in the DRC. Similar iron formations, clasts of which are found in overlying Nguba Group conglomerates, occur within the uppermost Upper Roan Group at Kasumbalesa (north of Konkola) in Zambia.
The bulk of the Mwashya Subgroup is composed carbonaceous siltstones and mudstone and lesser clastic carbonate rocks, which has a transitional boundary with the underlying carbonate rocks.
Mafic lava flows underlying Nguba Group rocks on the western margin of the Katanga basin in NW Zambia are dated at ~765 Ma, and are equated with the Mwashya Subgroup by Key et al. (2001).
A second period of rift extension (or renewed extension) is reflected by deepening and lateral enlargement of the Katanga basin onto basement margins and to the north into the Kundelungu Gulf Foreland, and by a period of bimodal magmatism. This magmatism, which commenced with the mafic volcanic and intrusive rocks of the late Upper Roan and Mwashya subgroups, as described above, and continued into the overlying lower Nguba Group, is principally evident as a ~30 to 100 km wide by ~500 km long corridor of intrusion that stretches across northern Zambia from the Kafue Anticline in the east, to the Angolan border. These rocks vary from mafic to ultramafic in composition, mainly amphibolites, ophitic gabbros and olivine gabbros, but also basaltic lavas, norites, picrites, peridotites, Iherzolites, troctolites and a few eclogites and serpentinites. They are often lensoid and discontinuous, with structural margins accompanied by brecciation (Porada and Berhorst, 2000), although large masses up to 10 km across have also been mapped. Based on limited dating, and stratigraphic relationships, these intrusions are interpreted to have been emplaced over the period ~765 to ~735 Ma, and are coeval with the mafic to intermediate volcanic rocks in the Dipeta and Mwashia subgroups and lower Nguba group in the DRC. In Zambia they frequently occur as gabbroic sills or sill swarms within the Upper Roan Subgroup dolostones, although they also intrude rocks from the basement Lufubu Metamorphic Complex to the lower Nguba Group.
The geochemical evolution of these intrusive mafic rocks and their interpreted volcanic equivalents, ranges from earliest continental tholeiite to alkaline and tholeiitic magmas, and finally in the DRC, to lavas with E-MORB affinities, suggesting a progression from continental to a possible embryonic oceanic rift (Kampunza et al., 2000).
Lesser coeval felsic intrusions are also encountered. A number of small 735±5 Ma (SHRIMP II U-Pb age; Key et al., 2001) granitoids (possibly A-type) and syenites have been dated in the western Domes Region in Zambia in the same area as the gabbroic intrusions, while Lobo-Guerrero describes ~765 Ma (U/Pb SHRIMP II) granitic dykes cutting Lower Roan Subgroup rocks below ore in the Nchanga pit. Similar aged mafic and felsic intrusions are also recorded further south in the Katanga Core (Lobo-Guerrero, 2005).
Stratigraphic relationships at the Kansanshi deposit suggest some of these intrusions may be Lufilian, <600 Ma in age, whilst scattered outcrops of gabbroic rocks further south in the Katanga Core, both tholeiitic and alkaline, give crystallisation ages from 570 to 520 Ma (Milani et al., 2015). Consequently, it is likely not all of the mafic intrusions in this corridor are of the same age.
The base of the overlying Nguba Group (previously Lower Kundelungu) is defined by the 10 to 1300 m thick Grand Conglomérat, a regional sequence of debris flows, glacial diamictites, some mafic flows, correlated with the world wide ~740 Ma Sturtian glacial event. In the Zambian Copperbelt, the Grand Conglomérat occurs as a regionally extensive sequence of debris flows and diamictites, with minor thin intercalations of siltstone and sandstone, and varies from ~10 to 100 m in thickness. It is essentially a pebbly mudstone which shows erosional basal contacts, slump structures, fabric and correlatable pebble-size variations, indicative of a mode of deposition by mudflows. The pebbly mudstone is interbedded with, and passes laterally into finely-bedded argillites. Clasts include rare rounded basement clasts but is dominantly subangular fragments of rocks of the Mwashia and Upper Roan subgroups, indicating widespread glacial erosion of the middle part of the Katangan Supergroup.
Within Zambia, the Grand Conglomérat is overlain by a 400- to 500-m-thick, upward-deepening sequence of carbonates, dolomitic sandstones and siltstones and siltstones-mudstones, becoming progressively carbonate-poor and coarser to the north (Cailteux et al., 2007; Batumike et al., 2006, 2007). The lowest member is the massive carbonate rocks of the Kakontwe Limestone represented by 350 to 500 m of massive dolostones and limestones in Zambia, and by a thinner sequence of carbonate-bearing to carbonate-poor siltstones and sandstones northward into the DRC, and appear to represent shallow marine to fluvial sediments. The Kakontwe Limestone is best developed on the northern margin and over the domes of the Domes Region.
To the north, mostly in the DRC, this succession is overlain by the Kundelungu Group, the base of which is defined by the Petit Conglomérat, a glacial diamictite, believed to be the time-equivalent to the global ~635 Ma Marinoan glacial event (Hoffmann et al., 2004; Master and Wendorff, 2011). The Petit Conglomérat is overlain by a weakly metamorphosed and deformed sequence that passes upwards from limestones and dolomitic sandstones (the 'Calcaire Rose', capping the diamictite), through dolomitic siltstones and argillites, to sandstones with lesser carbonate rocks. This sequence is, in turn, overlain by the extensive, flat lying, continental clastic molasse succession of the Plateaux Subgroup, composed of argillaceous and arkosic sandstones, and conglomerates (Cailteux et al., 2005; Batumike et al., 2006, 2007).
The inner core of the Lufilian Arc, in the SW, contains the syn- to post-tectonic Hook Granite batholith which is exposed over an area of >12 000 km2 (Naydenov et al., 2013). This composite magmatic complex has been uplifted and exposed along the regional Mwembeshi Shear Zone which forms its southeastern margin, but is indicated in magnetic data to continue at depth to the north over a much larger area. Aeromagnetic interpretation suggest the batholith contains multiple younger anorogenic granitoid ring complexes which overprint an earlier generation of intrusion (Nisbet et al., 2000) and has a strong WSW-ENE and north-south to NNW-SSE overprinting structural fabric (Naydenov et al., 2013; Lobo-Guerrero, 2005). Some of the structural trends reflect zones of high-strain deformation up to several km wide with augen-gneiss textures in their central cores (Naydenov et al., 2013).
The exposed Hook Granite batholith is mainly composed of fine- to medium-grained and coarse megacrystic granites and leucogranites, rhyolites and syenites with some associated migmatites (Hanson et al., 1993; Naydenov et al., 2013; Milani et al., 2015). Phases of this complex have been dated at 559±18 and 566±5 Ma (syntectonic; Hanson et al., 1993), 549±2 Ma, 541±3 Ma and 533±3 Ma (Naydenov et al., 2013), while Milani et al. (2015) state that recent new U/Pb age data constrain most of the felsic magmatism to between 550 and 540 Ma. Scattered outcrops of gabbroic rocks, both tholeiitic and alkaline, which are generally coeval with the granitoids, give crystallisation ages from 570 to 520 Ma (Milani et al., 2015). This bimodal magmatism (mafic to predominantly felsic) is characterised by both an alkali-calcic and an alkalic suite, with typical A-type, metaluminous, high Fe/Mg and K/Na geochemical signature, while occasional sodic granitoids have also been documented (Milani et al., 2015).
To the west of the Kafue Anticline, in the western Domes Region (see Geological Setting map below), surrounding the Kabompo and Mwombezhi Domes (see the Domes Region maps below), the generalised metamorphosed Katangan succession commences with (after Gregory et al., 2012 and sources quoted therein):
• localised developments of a sequence that comprises a basal sandstone (50 to 100 m of medium to fine grained, variably schistose phlogopite-quartz rock); siltstone, shale and graphitic shale (100 to 200 m of micaceous, phyllitic shales and siltstones, with locally preserved bedding and recumbent folding); overlain by a further 50 to 100 m of conglomeratic siliciclastic rocks;
• a more extensive and continuous sequence commencing with 20 to 50 m of pebbly, quartz-rich sandstone; 200 to 400 m of massive quartzite with occasional clasts of quartz and feldspar, interbedded with variably evaporitic siltstones towards the top; 100 to 200 m of variably evaporitic, laminated to massive anhydrite-talc-sericite-silica bearing siltstone with variably developed hematite-siderite-albite alteration, including a <10 m thick graphitic shale with crackle breccia with kyanite-quartz infill and local albitisation. This sequence has been significantly altered and recrystallised during intense metasomatism;
• a carbonate rich sequence, commencing with a 10 to 20 m thick pebbly carbonate with a prominent dissolution texture (a fluidised dolomitic breccia); overlain by 10 to 20 m of equigranular to marbleised dolostone; 50 to 150 m of dolostone and calc-silicate rocks (after marls), which frequently contain kyanite porphyroblasts, intercalated with thin black shale and minor siliciclastic rocks (quartz-biotite schists);
• turbiditic and graphitic shale, comprising ~30 m of cm to metre scale carbonaceous (mainly graphite) shale beds, disrupted by pebbly sandstone with rip-up clasts, in possible fault contact with the underlying carbonate sequence;
• shaley diamictite, equated with the Grand Conglomérat, comprising >50 m of fine grained massive micaceous rock with rare coarse cobbles of quartz rich sedimentary rock. The fine matrix is dark and may be locally carbonaceous in places, although the majority of the dark material appears to be fine grained micritic biotite.
This sequence is lithologically very similar to the Lower and Upper Roan and Mwashya subgroups, and basal Nguba Group found in the main Zambian and Congolese copperbelts, although Gregory et al. (2012) suggest the Lower Roan Subgroup may not be represented in this sequence, and all rocks below the turbiditic graphitic shale belong to the Upper Roan Subgroup.
Basement is dominantly composed of medium to coarse grained quartz-biotite-muscovite±hornblende schists, with local development of pink-red garnet and/or blue-green kyanite porphyroblasts. A flat-lying, biotite-defined schistosity is ubiquitous, with common boudinaged layer-parallel quartz veins.
The Katangan and basement rocks are separated from the by a décollement zone represented by a strongly foliated quartz-phlogopite/biotite rock, that is essentially a transition zone between the two.
South of the main Zambian Copperbelt, where the Domes Region intersects the basement Irumide Belt, structural trends are ~ESE-WSW, parallel to the Mwembeshi Shear Zone and the main structural grain of the Irumide belt. In this area, surrounding and north of the Kabwe Zn-Pb deposit, the Katanga Supergroup unconformably overlies a Palaeo- to Mesoproterozoic basement complex largely made up of older Lufubu Metamorphic Complex rocks and younger Muva schist and quartzite, as well as mafic lavas of uncertain age. The lowest unit is a basal conglomerate, correlated with the Lower Roan Subgroup, overlain by the Kangomba Formation, a mixed unit of arkose, quartzite and conglomerate at the base, overlain by metasiltstone, schist and phyllite, capped by a dolostone (Moore, 1964; Cairney and Kerr, 1998). As discussed by Kamona and Friedrich (2007), the lower clastic and upper carbonate units of the Kangomba Formation have been correlated with the Lower and Upper Roan subgroups respectively. Kampunzu et al. (2009) regard the lower units as equivalent to the Lower Roan Kibalongo Formation (as defined above) and the remainder to the Upper Roan (Bancroft Formation). The Bancroft Formation comprises (Kortman, 1972): i). grey arenaceous dolomite; ii). light grey massive dolomite hosting the main Kabwe Zn-Pb-Ag orebodies; iii). grey slightly carbonaceous dolomite; iv). dark carbonaceous dolomite, which is also mineralised locally; and v). grey to pink argillaceous dolomite with talcose partings.
These are overlain by the Nyama Formation, a predominantly phyllite-dolomite unit with associated limestone-marble, which has been correlated with the Nguba Group (Moore, 1964; Intiomale, 1982), but it is also possibly equivalent to the Upper Roan Subgroup.
Taylor (1954), Kortman (1972), and Intiomale (1982) recognised an Nguba Group equivalent lithostratigraphy at Kabwe, from the base, of: i). a matrix-supported conglomerate (Grand Conglomérat or Mwale Formation); ii). a finely bedded dolostone alternating with carbonate-rich and carbonaceous shales (the "Schistose Dolomite"); iii). a grey to dark grey bedded dolomite including lenticular carbonaceous shales ("Carbonaceous Dolomite" - which with the "Schistose Dolomite" are regarded as equivalents of the Kaponda Formation of the DRC); iv). light grey massive dolostone with frequent zones of recrystallised dolomite, locally fractured at the base (equivalent of the Kakontwe Limestone; Kampunzu et al., 2009).
The equivalent Katanga Supergroup sequence in the Zambezi Belt, to the south of the Mwembeshi Shear Zone, where it hosts the Nampundwe Cu-pyrite deposit, includes a syn-rift, bimodal volcanic suite (the Nazingwe metabasalts and Kafue rhyolites) which yielded zircon ages of 879±19 Ma (Wardlaw, quoted in Hanson et al., 1994), and the associated Lusaka Granite (854±12 Ma; Barr et al., 1977). The Lusaka Granite is intrusive into basement, and tectonically overlain by Katanga Supergroup rocks. This magmatism is earlier than the commencement of sedimentation in the Lufilian Arc further north in Zambia where the broadly coeval ~880 Ma Nchanga Red Granite is separated by an erosional surface from the basal Katanga Supergroup. Within the Zambezi Belt, the Katangan Supergroup equivalents commences with the Chunga Formation, composed of feldspathic quartzites, calcareous schists and dolomitic limestone, with the bimodal volcanic rocks at the base. The overlying Cheta Formation comprises a thick basal limestone, followed by quartz-muscovite (-chlorite) schist and quartzite. This is followed, in turn, by the Lusaka Dolomite, which ranges from dolostone to limestone and has been regarded as an equivalent of the Nyama Formation and Nguba Group, although it could also be part of the Upper Roan Subgroup (Barr et al., 1978; Kampunzu et al., 2009). The Kawenta Formation marks the top of the sequence, and is argillaceous at the base overlain by a psammitic facies. All of these rocks have been subjected to medium to high grade metamorphism (Petters, 1986), occurring as paragneiss and high grade schist and amphibolites after the mafic volcanic rocks, which are the Makuti-Rushinga, Malaputese and Kishira groups in Zimbabwe.
The equivalents that lap onto the Archaean Zimbabwe craton to the south of the Zambezi Belt, are represented by limestone, dolomite and orthoquartzite of the Tengwe River Group, and the red grits, sandstones, shales and conglomerates of the Sijarira Group.
Tectonic, Tectono-stratigraphic and Structural Setting
The tectonic evolution of the Lufilian Arc culminated in the main (late-Katangan) Lufilian Orogenesis, which appears to span a period of >100 m.y. The oldest metamorphic ages are at ~590 Ma, whilst the main stage orogenesis was from 560 to 530 Ma (Tack et al., 2010), based on U-Pb zircon dating of syn- to post-tectonic granites and rhyolites in the Katanga core (Hanson et al., 1993). The syn- to post-tectonic composite Hook Granite, which cuts the Nguba Group, comprises an older, magnetically flat set of medium-grained biotite-hornblende granitoid intrusions, dated at 559±18 and 566±5 Ma in two samples. These are cut by numerous, younger microcline-biotite-hornblende megacrystic phases reflected by donut-shaped magnetic anomalies with diameters of from <10 to 50 km, interpreted to represent buried anorogenic ring complex intrusions dated at 533±3 Ma (Hanson et al., 1993; Nisbet et al., 2000; Lobo-Guerrero, 2010). Hypabyssal rhyolite, intruded during displacement along the Mwembeshi Shear Zone, has been dated at 551±19 Ma. Post-tectonic felsic magmatism in the same area occurred at about 538-533 Ma (Hanson et al., 1993). A peak metamorphic U-Pb monazite age of ~530 Ma was obtained from white schist facies rocks (talc-kyanite, a low-temperature high-pressure assemblage) which are restricted to the mylonitic detachment marking the structural transition from Palaeoproterozoic gneisses and schists in the cores of domes in the central and western parts of the Domes region, to the overlying and fringing greenschist facies Katanga Supergroup sequence (John et al., 2004). Widespread dates of 510 to 465 Ma possibly record post-orogenic cooling (Selley et al., 2005, and sources cited therein).
This orogenesis was the result of oblique compressive basin inversion, which occurred throughout the Katangan and Damaran systems from southern DRC to the Atlantic Ocean and occurred to east-west directed collision in the Mozambique Belt to the east, during accretion of the short-lived supercontinent Pannotia.
The Lufilian Arc comprises four distinct, north-convex, arcuate tectonic subdivisions, the:
i). External Fold and Thrust Belt to the northeast, mainly in the DRC (where it hosts the Congolese Copperbelt), characterised by thin-skinned thrust/nappe-dominated deformation, absence of exposed basement, low-grade metamorphism and structural repetition of the Katangan stratigraphy;
ii). Domes Region, which includes the Zambian Copperbelt in its outer margins, comprises a series of exposed basement domes, characterised by upper-greenschist facies metamorphism in the east, increasing in intensity to upper-amphibolite in the west.
The belt is also characterised by a corridor of gabbroic intrusions, cutting both the basement in the domes, Upper Roan Subgroup and Nguba Group country rocks.
The individual domes west of the Kafue Anticline, coincide with magnetic highs within a well defined arcuate belt of greater magnetic relief than the neighbouring Synclinorial Belt.
Unconformable relationships between Katanga Supergroup rocks and older basement are preserved on the flanks of the Kafue Anticline, where upright to inclined, high-amplitude folds dominate. However, tectonic decoupling has been described at the same contact with basement domes further west, which are cored by recumbent folds and nappes, and juxtaposed thrust sheets of contrasting metamorphic grade (Cosi et al., 1992; Binda and Porada, 1995; Key et al., 2001).
Daly et al. (1984) interpret the basement inliers in the Domes region to represent antiformal stacks above mid- to lower crustal ramps.
These domes resemble metamorphic core complexes, capped by domal, radially outward-dipping detachments/décollements. The rocks above the décollements are usually metamorphosed to greenschist facies and comprise Katanga Supergroup lithologies, while those below are strongly metamorphosed Palaeo- and Mesoproterozoic gneisses and schists, with interpreted interleaved metamorphosed Neoproterozoic slices, and include quartz-feldspar-biotite ±magnetite gneisses, garnet-bearing granite gneisses, etc., with a strong mylonite texture in the upper parts of the dome (cf., at Lumwana in the Mwonbezhi Dome). Talc-kyanite white-schists occur within the décollements between the basement and the Katanga Supergroup sedimentary sequence in the Domes (Hitzman et al., 2012), dated at ~530 Ma (John et al., 2004). Talc-kyanite white schists may be taken to represent ultra-high pressure, but low to moderate temperature metamorphism (e.g., John 2004), associated with subduction, collision and overriding of the Congo and Kalahari Craton. However, Hitzman et al. note that their occurrence in basement and immediately overlying Katanga Supergroup rocks within hundreds of metres of overlying greenschist facies Katanga rocks, suggests structural complexity and/or mobility of aluminium and unusual mineral growth during high-salinity, Mg-rich metasomatism. In addition, the white schists are localised, restricted to the margins of the domes and do not persist into the structural footwall, which would be expected in the John (2004) interpretation. Elsewhere, white schists are interpreted to have formed from granitic or sedimentary (sometimes evaporitic) protoliths by syn-metamorphic oxidation and Mg-metasomatism (e.g., Mautia Hill, Tanzania; Jons and Schenk, 2004).
While the domes may represent extensional metamorphic core complexes (or the exposed crests of a larger undulose complex or complexes) formed during the renewed extension phase, they have been substantially modified, deformed and metamorphosed during the later compressive Lufilian Orogeny. The variations in metamorphic grade, shape and size, imply that, if they are metamorphic core complexes, they have been more deeply exhumed, and/or represent more deeply derived and uplifted core rocks to the west.
The arcuate chain of domes described above, obliquely overlap, overprint and coalesce with the southern half of the Kafue Anticline on the southeastern margin of this region. The Kafue Anticline was a basement high during deposition of the Katanga Supergroup, although it also shares some of the extensional and subsequent compressional features seen in the domes to the west, and is locally fringed by décollement structures (e.g., in the Nchanga-Mindola district). The easternmost Kabuche Dome, appears to updome the Lower and Upper Roan subgroups, rather than act as a basement high against which they taper, as is the case with the Kafue Anticline high. This is consistent with the arcuate belt of domes postdating the Kafue Anticline high and Roan Group deposition;
iii). Synclinorial Belt, where sedimentary rocks were subjected to large scale folding during at least two deformation events, and low grade metamorphism and is reflected by a more subdued pattern in magnetic data, contrasting with its neighbouring regions. It has been suggested this sequence, reflects a change from a marginal shelf in the north in what is now the External Fold and Thrust Belt, to a deeper basin SW of the Domes region (Cosi et al., 1992; Porada and Berhorst, 2000). However, Selley et al. (2005) suggested that they could find no evidence for a deeper marine sequence in the Synclinorial Belt; and
iv). Katanga Core (or Katanga High) to the southwest, in which only the upper parts of the Katanga Supergroup and almost all of the outcropping granitic intrusions of the Lufilian Arc are exposed (Petters, 1986; Selley et al., 2005). It is reflected by a more complex and variable pattern in aeromagnetic data, with a well defined margin.
The Katanga High was first proposed by De Swardt and Drysdall (1964), who considered that it represented a central core of uplift (for unknown reasons), which induced gravitational sliding of the Katangan cover sequence to both the north and the south. To the NE, Roan (Nguba) and Kundelungu group rocks were folded, imbricated and thrust northwards to form the Lufilian Arc. As a mirror image, the Zambezi Belt developed from similar processes to the SW, with late-stage transcurrent movements on the Mwembeshi Shear Zone juxtaposing low-grade rocks of the Lufilian Belt and high-grade rocks of the Zambezi Belt. Porada (1989) and Porada and Berhorst (2000) argued against this interpretation, and suggested instead, the Katanga High was composed of late Katangan rocks over shallow basement, over-thrust to the NE onto the Synclinorial Belt during the Lufilian orogeny.
The exact nature of the Katanga High remains unclear. Hitzman et al. (2012) do not differentiate it from the core of the broader Katangan basin. It may well occupy the central core of a wide, NE-SW elongated, shallow basin of marine-shelf facies rocks that extended from the Kasai/Congo craton in the NW, to south of the Mwembeshi Shear Zone to the Kalahari Craton to the SE, and from the Foreland and Bangweulu Block to the NE, into Angola, Namibia and Botswana to the SW. The current geophysical expression of the Katanga High may be no more than a reflection of the superimposed late-Lufilian magmatic event intrusions represented by the syn- to post-tectonic composite Hook Granite complex.
The northeastern margin of the Lufilian Arc is represented by exposures of the relatively undeformed upper units of the Upper Kundelungu Group within the Kundelungu Gulf Foreland. The eastern margin of the arc, to the east of the Zambian Copperbelt, passes into a similar prong of Nguba Group overlying Irumide rocks, separated from the Kundelungu Gulf by the triangular Bangweulu Block. This embayment represents transgression onto Irumide basement during the renewed extension that accompanied the onset of Nguba group deposition. The southern limit is defined by the major, WSW-ENE trending, sinistral Mwembeshi Shear Zone which separates it from the Zambezi Belt.
Following the two extensional events and deposition of the majority of the Katanga Supergroup, Late Neoproterozoic to Lower Cambrian basin inversion was initiated. Kinematics suggest NW to NE directed thrusting during this orogenesis, with displacement vectors radiating perpendicular to the arcuate trend of the fold belt (Selley et al., 2005). However, Porada and Berhorst (2000) and Kampunzu and Cailteux (1999) suggest the arcuate shape is the result of oblique compression during this event, between the northern margin of the Kalahari craton and the southwestern edge of the Congo craton. The stress field was complicated by the east-west compression that formed the major Mozambique Belt collision zone to the east, and resultant sinistral movement on the Mwembeshi Shear Zone, to produce 'oroclinal bending' and form the Lufilian Arc.
See the Congolese/Katangan Copperbelt record for a more detailed summary of the deformation stages that formed the Lufilian Arc.
In Zambia, these phases of deformation are expressed as follows:
• Early Extension, which followed an ~100 to 200 Ma period of quiescence after the amalgamation of the Rodinia Supercontinent, and marks the early onset of its break-up.
Within Zambia, this extension was directed NE-SW, parallel to the Mwembeshi Shear Zone, forming a series of fault controlled, NW-SE elongated basins. These basins were developed towards the SW limit of the thicker sequences within the precursor Kibaran and Irumide rift basins, which were thickest and widest to the NE, and thinned towards the region in which the Katanga Supergroup was subsequently deposited.
This extension was heralded by bimodal magmatism south of the Mwembeshi Shear Zone (and the Lufilian Arc), in the Zambezi Belt, commencing with basal bimodal rift volcanic rocks (Nazingwe metabasalts and Kafue rhyolites) which yielded zircon ages of 879 ±19 Ma (Wardlaw, quoted in Hanson et al., 1994) and the limited Lusaka Granite (854±12 Ma; Barr et al., 1978) which is intrusive into basement, and tectonically overlain by Katanga Supergroup rocks. These volcanic units are interbedded with sedimentary sequences in the Zambezi Belt. Just to the north of the Mwembeshi Shear Zone, in the Kabwe area, mafic lavas of uncertain age which overlie the Irumide sequence may be related to this phase. Within the main Lufilian Arc, the coeval Nchanga Red Granite (877±11 Ma; U-Pb SHRIMP zircon; Rainaud et al., 2000) and similar granites in the basement at Nkana-Mindola are suspected to be of this age also (Croaker, 2011). Unlike the equivalent magmatism in the Zambesi Belt, no volcanic component is present or preserved, and the granites have been uplifted, exposed and partially eroded prior to the first Katangan deposition. This suggests that during early extension, intrusion of the granites at Nchanga and Nkana-Mindola accompanied uplift of the structural high that occupied what was to become the core of the Kafue Anticline (see below). Similar granites have been dated in basement near Lumwana (Barrick, 2014) in the western Domes Region.
To the north of the Lufilian Arc, the 813±30 Ma Gagwe Amygdaloidal Lavas, are deposited on the western flanks of the Tanzanian Craton, overlying Kibaran basement.
The initial deposition of the Katanga Supergroup within the Lufilian Arc, was in narrow grabens, represented by the Lower Roan Subgroup Mindola Clastics Formation, which became interconnected and with further subsidence, and grew into the larger rift basin that hosts the overlying Kitwe Formation and then the sag phase Upper Roan Subgroup.
During this phase, the NNW-SSE trending structural high that was to become the core of the Kafue Anticline, influenced the distribution and thickness of facies. This structure also included the basement exposed in the Konkola and Luina domes, now separated by D1 and D3 synforms. It was partially emergent during deposition of the Mindola Clastics Formation, which on the rims of, and in remnant structural basins (e.g., Luanshya basin) in the core of the "anticline", contain basal conglomerates, channel sediments incised into basement, and aeolian sandstones.
The same basement high was partially submerged during the deposition of the overlying Kitwe Formation, which grades from arenites over the margins of the basement high, to a well defined shale corridor, before passing into deeper water turbiditic sedimentary rocks in the Synclinorial Zone to the west. The structure was subsequently largely submerged, overlain by the shelf/carbonate-rich to lagoonal Upper Roan subgroup sequence (Porada and Berhorst, 2000);
Breccias, interpreted to be after mobilised evaporitic units, are found within the Upper Roan subgroup over and adjacent to the Kafue Anticline. These breccias and interpreted evaporitic layers are much less developed than in the similar lithologies and halokinetic megabreccias of the mineralised sequence in the DRC.
• Renewed Extension, from ~765 to 735 Ma (Hitzman et al., 2012), commencing during the late Upper Roan Subgroup and accompanying deposition of the Mwashya subgroup and Nguba Group. During this period, the main centre of deposition migrated north from the region principally to the west of the "Kafue Anticline" basement ridge in Zambia, to be located in Katanga in the DRC, between the Domes Region and the Kundelungu Gulf Foreland (see the Congolese/Katangan Copperbelt record for more detail).
It is suggested here, that during this event, NE-SW extension resulted in the development of an elongate metamorphic core complex (or string of complexes), the undualting crest of which is exposed as the individual domes of the Domes Region, including the Kabompo Mwonbezhi, Solwezi, Luswishi Dome and Kabuche domes, but not the "Kafue Anticline" (and subsidiary Luina and Konkola domes). The "Kafue Anticline" basement high and subsidiary domes had been uplifted as a faulted basement high during the initial stages of the 'Early extension', accompanying and succeeding intrusion of the Nchanga Red Granite, which was exposed by uplift and erosion prior to deposition of the basal Katanga Supergroup. The arcuate core complex(es) of the Domes Region intersects the earlier basement high obliquely from Nchanga in the north, to south of Luanshya in the south.
It is also suggested that uplift associated with this metamorphic core complex, and the coeval deepening of the rift basin to the north, initiated northward verging gravity gliding of the evaporite-rich Upper Roan Subgroup over a décollement, which followed the interpreted salt/evaporite bed of the R.A.T./Upper Roan Subgroup. This produced a modified 'intraformational' package, comprising a folded and imbricated stack of north-vergent folded thrust sheets and finally a zone of diapiric thickening in the mid External Fold and Thrust Belt (see the schematic section in the separate Congolese/Katangan Copperbelt record). This package, largely composed of megabreccia in the NE, represents extension and thinning of this unit in the Domes Region of northern Zambia, and shortening and thickening in the External Fold and Thrust Belt of the DRC. The upper limit of this deformed package would appear to be a breccia zone within the upper Dipeta Subgroup in DRC, below the comparitively little deformed Mwashya Subgroup.
Both the Mwashya subgroup and Nguba Group are found over and flanking the Kafue Anticline and associated Konkola and Luina domes, the Solwezi dome and the External Fold and Thrust Belt. The dominantly shale facies of the Mwashya subgroup are best developed in the core of the rift basin in the External Fold and Thrust Belt in the DRC, grading into sandy facies on the margin with the Kundelungu Gulf Foreland to the NE, and to the SW towards the Domes Region (Cailteux et al., 2007). At the same time, deposition extended to the NW and SE over the marginal Kibaran and Irumide basement respectively, and into the Kundelungu Gulf Foreland. The Mwashya subgroup is absent or only present as oxidised conglomerate-sandstone wedges in these transgressive sequences, and is not mapped around the westernmost domes or to the SW of the domes, in the Synclinorial Belt or the Katanga Core, although they may have not been differentiated in these areas. The Nguba Group however, is well developed over most of these same intervals, although it appears to thin over the domes to the west of the Kafue Anticline, while the best developments of the Nguba Group Kakontwe Limestone flank these domes, suggesting they were emergent or shallow during Nguba Group deposition.
A ~30 to 100 km wide by ~500 km long, arcuate ESE-WNW trending corridor of gabbroic to dioritic intrusions follows the Domes region from the Luanshya Structural Basin through the Chambishi Structural Basin, and the Kabuche, Luswishi, Solwezi, Mwombezhi and Kabompo domes. These rocks are described above and are interpreted to be coeval with the thin (up to 50 m) mafic to intermediate volcanic rocks in the Dipeta and Mwashia subgroups and lower Nguba group in the DRC which form a regional volcanic belt that follows the Congolese Copperbelt.
• Main Stage Lufilian Orogenesis, commencing at ~590 Ma, but according to Armstrong et al. (2005), was spread over the interval from 600 to 512 Ma, corresponding to the D1 Kolwezian tectonic event of François (1974) and Kampunzu and Cailteux (1999). The earliest greenschist facies metamorphism has been dated at 592±22 Ma (U-Pb monazite) and 585±0.8 Ma (Ar-Ar biotite; Rainaud et al., 2002), with peak (?) metamorphism at ~530 Ma for talc-kyanite white schist metamorphism (John et al., 2004), which is not regional, but localised in the thrust mylonites that cap the domes of the western Domes region.
This, generally NE vergent compressional stage, corresponds to the structural inversion of the Katangan rift basin, and is characterised by complex polyphase deformation and superimposed thrust terranes, curved folds and sinistral strike-slip shear/fault zones.
Décollements related to the earlier extensional phases appear to have been reactivated in the domes to the west of the Kafue Anticline, and rocks of the Mwashia and Nguba groups have been isoclinally, folded immediately to the NE of the domes, as interpreted at the Kansanshi deposit (see the separate Kansanshi record and the schematic section in the separate Congolese/Katangan Copperbelt record).
The structure over and adjacent to the Kafue Anticline is characterised by thrusting within the basement, passing up to be expressed as folding within the overlying Roan Group rocks. This is evident across the anticline from Chingola-Nchanga eastward to Mufulira. In the west of this section, ENE verging thrusts follow the basement-Roan Group contact, passing upwards to emplace imbricated and interleaved wedges of basement gneiss and granite into Roan Group rocks. Further east, the basement thrust climbs into the Roan Group, and follows the Copperbelt Orebody Member of the basal Kitwe Formation as a décollement. This structure separates thrusted deformed basement, granite and overlying Mindola Clastics Formation siliciclastic rocks, from overlying, folded, Kitwe Formation lithologies. Similar décollements and decoupling are common at this stratigraphic position elsewhere, e.g., at the Luanshya, Nkana and Chambishi deposits, exploiting the more fissile lithologies of the Copperbelt Orebody Member. Eastward, towards the centre and eastern margin of the Kafue Anticline, WSW facing folds and east dipping reverse faulting, thrusting and banded mylonite zones are mapped. On the eastern margin of the Kafue Anticline, at Mufulira, ENE verging folds are evident although, these face downwards. Similar structures are seen elsewhere on the same margin (Daly et al., 1984).
Daly et al. (1984) interpret this structural pattern to reflect ENE vergent thrusting above a deep décollement within the basement of the Kafue Anticline, with associated branching subsidiary thrusts that climbed up through the sequence. The back thrusts to the east are interpreted to be compensatory structures, while the downward facing folds are taken to be equivalents of the structures on the western flank that have been subsequently tilted as the Kafue Anticline rose as a thrust culmination.
The Kafue Anticline is also characterised by a series of basinal and domal structures e.g., the Luanshya and Chambishi structural basins, the Konkola and Luina domes and the basin between the Luina, Konkola domes and northern Kafue Anticline. These structures appear to be the result of interference between an earlier NE-SW and a later NNE-SSW compressional event. It is suggested that during the three separate pulses recognised by Croaker (2011), the direction of compression may have rotated to produce this interference pattern. Croaker (2011) also suggests the pattern of folding may be partly influenced by the orientation of the original rift/graben depositional basins and inhomogeneity of facies distribution, consistent with Annels (1984) who proposed the interference pattern is due to the overprinting of initial ESE-WNW trending rift phase depositional basins and the NE-SW compression. These structures are also possibly related to the oblique merging of the early NNW-SSE 'Kafue Anticline' basement rise and the NW-SE trending Domes Region structures.
A D2 tectonic event is recognised in the northern sections of the Congolese Copperbelt (the Monwezian event of François, 1974, and Kampunzu and Cailteux, 1999), comprising a series of generally WSW-ENE to east-west sinistral, branching, strike slip faults displacing D1 Kolwezian structures. To the east and SE these structures curve to parallel the trend of the Lufilian Arc and trend SE. An equivalent structural phase is not mentioned in the literature perused for Zambia, other than the major WSW-ENE trending sinistral Mwembeshi Shear Zone to the south which truncates the Lufilian Arc and separates it from the Zambezi Belt. Possibly the set of WNW-ESE trending structures which offset the D1 folds of the Kafue Anticline area may be related to this event.
Kipata (2013) interpreted an oroclinal bending stage, transitional between D1 and D2, related to lateral mechanical constraints between the Kibara belt to the NW, and the Bangweulu block to the east. He suggested this led to dextral displacement along the NE-SW trending Kibaran margin to produce east-west to WSW-ESE trends in the NW. Sinistral displacement recorded along the Mwembeshi Shear Zone produced a comparable SSE-NNW trend in the southern section of the arc in Zambia, thus creating the overall arcuate structure.
• Late Orogenic Extensional Collapse, a brittle event, only recorded in the Lufilian Arc, which Kipata (2013) regards as probably post ~530 Ma. It caused NW-SE directed extension across the whole arc, and reactivation of earlier compressional and strike-slip structures. Selley et al. (2005) suggest the widespread 510 to 465 Ma 39Ar/40Ar biotite and a Rb/Sr muscovite plateau age of 483.6±1.1 Ma (Cosi et al., 1992; Torrealday et al. 2000; Rainaud et al., 2002; John et al., 2004), most likely record post-orogenic cooling. According to Zientek et al. (2013) low cooling of the Lufilian Orogen is indicated by apparent 39Ar/40Ar ages obtained from microcline in the Zambian Copperbelt at Musoshi that range from 467.0±2.7 to 405.8±3.8 Ma.
• Post-orogenic Transpressional Inversion, a NW-SE brittle transpressional inversion recorded in the Lufilian Arc also affects the Kundelungu Foreland and the fringing Irumide and Ubendian basement. It corresponds to the Lufilian D3 Chilatembo stage of Kampunzu and Cailteux (1999) and took place after the Lufilian extensional collapse. François (1993) suggested an age of ~305 Ma for this event, which Kipata (2013) similarly regards to be post-Lufilian, "most likely Palaeozoic in age". It produced large, gentle, open, upright folds with axes varying from NNE-SSW to ENE-WSW (e.g., the ENE-WSW oriented syncline that separates the Luina and Konkola domes from the main Kafue anticline), and north-south and east-west faults, low-angle thrusting of Nguba and Kundelungu group units, possibly accompanied by late salt diapirism in the DRC (Kampunzu and Cailteux, 1999; Schuh et al., 2012; Kipata, 2013). This phase is responsible for much of the interference folding seen in the DRC and northern Zambian sections of the Lufilian Arc.
• Uplift, Peneplanation and Cratonisation, followed by more recent extension related to the Cenozoic African Rift.
Distribution of Mineralisation - Zambian Copperbelt and Domes
Traditionally, the ore deposits of the Zambian Copperbelt have been interpreted to lie within the Neoproterozoic Lower Roan Subgroup, composed principally of coarse siliciclastics (conglomerate to arkose and siltstone, with lesser carbonate rocks). The main deposits define two NW-SE trending parallel lines of Cu mineralisation some 20 km apart, separated by the Palaeoproterozoic basement gneisses, granitoids and schists, and Mesoproterozoic conglomerates, quartzites and granitoids that make up the Kafue Anticline, in the eastern Domes Region of the Lufilian Arc. Each of these two belts is 5 to 20 km wide and up to 150 km long. Ore grade mineralisation, however, tends to occupy linear, often more structurally complex, semicontinuous bands, up to 2 to 3 km wide, and as much as 17 km long, particularly on the SW line, interrupted by narrow barren gaps and cross folded anticlinal basement ridges (e.g., the Nkana-Mindola, Nchanga and Konkola strings of deposits separated by barren gaps which coincide with basement hills and reef facies dolostones). The majority of the deposits are on the SW of the two lines of mineralisation, which coincides with a corridor of shale facies developed at the base of the Kitwe Formation, and lapping onto basement to the east.
Within these two belts of deposits, there are some 7 major and >25 minor stratabound deposits. The major deposits are Luanshya-Baluba, Mufulira, Frontier-Lufua, Nkana-Mindola, Nchanga-Chingola, Chambishi and Konkola-Lubambe-Musoshi. The larger orebodies had production + resources that range from 90 to 1000 Mt @ 2.4 to 3.6% Cu. Between 1930 and 1987 some 24 Mt of copper metal was produced from 1.07 Gt of ore averaging 2.71% Cu. The total mined ore plus reserves/resources has been calculated at 3.28 Gt @ 2.68% Cu containing ~88 Mt of Cu (Selley et al., 2005 and references cited therein).
The Lower Roan Subgroup is conformably overlain by Neoproterozoic carbonate rocks of the Upper Roan Subgroup which is equivalent to the R.A.T., Mines and Dipeta subgroups in the External Fold and Thrust Belt of the neighbouring Democratic Republic of Congo (DRC). Major deposits have not yet been discovered in these in the Zambian Copperbelt, but host most of the significant Cu-Co deposits of the Katangan section of the Central African Copperbelt in the DRC.
More recently, the Lumwana Cu-Co-U and Kansanshi Cu-Au operations, located in the central to western Domes Region to the west of the established Copperbelt, were brought into production and the Enterprise Ni-Cu and Sentinel Cu-Co deposits discovered in the same region. In 2013 Lumwana and Kansanshi were the biggest copper producers in Africa, each with large, lower grade resources of 800 to 1000 Mt @ 0.5 to 0.7% Cu. These deposits have differences in the apparent mode of occurrence compared to the main copperbelt deposits fringing the Kafue Anticline. See the two overlapping images below for a geological summary of the Domes Regions and distribution of units described above. For more details of the local geology and the mineralisation styles, see the records for the Lumwana (Chimiwungo and Malundwe), Kansanshi, Enterprise, and Sentinel deposits.
Click here for a composite of these two images of the full Zambian Domes Region, best viewed on a large screen.
Mineralisation
Mineralisation within the major deposits 'flanking' and overlying the Kafue Anticline has the following characteristics:
Fault Controls
The geometry, size and distribution of orebodies is closely linked to early fault-controlled sub-basin architecture during deposition of the Lower Roan Subgroup. Basement faulting affected facies distribution on basement margins, the formation of structural and stratigraphic culminations influencing fluid flow and entrapment, and may also have provided pathways for fluid ingress, e.g., most argillite hosted orebodies overlie anomalously condensed wedges of Mindola Clastics Formation, where the overlying Copperbelt Orebody Member is transgressive onto basement, coincident with fault related trough margins/basement highs. Many orebodies are also located at the intersection of basin bounding faults. In addition, footwall and hanging wall arenite hosted orebodies are also frequently aligned along basin margin faults (Selley et al., 2005 and references cited therein).
Many of the early basin controlling faults appear to have been reactivated during Mwashya to lower Nguba extension, reflected by changes in stratal thickness of Roan Group rocks and by increased folding and deformation that was commonly focused by these structures during Lufilian deformation (Hitzman et al., 2012).
Mafic Magmatism
Many of the largest deposits in the Central African Copperbelt, have a spatial association with mafic magmatism, e.g., Kamoa and Kolwezi in the DRC and Konkola and Kansanshi in Zambia, which are spatially associated with abundant mafic sills or flows or aeromagnetic anomalies that may represent buried mafic intrusions (M. Hitzman, unpub. data, 2010). The line of deposits of the Domes Region in Zambia (Sentinel, Enterprise, Lumwana and Kansanshi) fall within and are aligned along the major corridor of gabbroic intrusions that occupy that structural feature. In addition, the same gabbroic corridor obliquely intersects the Kafue Anticline from Luanshya in the south to Nchanga in the north, although smaller mafic dykes are also found at Konkola. The flanks of the Kafue Anticline over this interval hosts virtually all of the large Cu-Co deposits of the Zambian Copperbelt. The corridor of mafic magmatism in the Domes/Kafue Anticline region of Zambia is dated at ~753 and 742 Ma (from limited datings), while a belt of mafic intrusions and extrusions in the Congolese Copperbelt was emplaced from 765 to 735 Ma. Scattered outcrops of gabbroic rocks in the Katanga Core, both tholeiitic and alkaline, which are generally coeval with the Hook Granite batholith, give crystallisation ages from 570 to 520 Ma (Milani et al., 2015), and are possibly also present in the Domes Region.
Stratigraphic Control
Copper ±Co ±Ni ±Au ±Ag ±U mineralisation within the Central African Copperbelt, emplaced after the commencement of deposition of the Katanga Supergroup at <880 Ma, to the end of the late Lufilian Orogenic cycle, is found in rocks from the basement Lufubu Metamorphic Complex (e.g., Samba) to ~510 Ma (e.g., Kansanshi).
Stratabound mineralisation is restricted to host rocks from the "Footwall" orebodies in the Mindola Clastics Formation (Lower Roan Subgroup), to the Grand Conglomérat of the basal Nguba Group. Mineralisation in rocks older and younger are predominantly transgressive vein style, although they may include substantial amounts of bedding plane replacement outwards from mineralised vein margins.
Significant Cu-Co, or Cu-Au or Cu-Ag ore deposits, are found in association with the following units within the Central African Copperbelt, including those that only host ore in the DRC:
• A package of rocks, up to ~150 m thick, straddling the Copperbelt Orebody Member, incorporating sections of the upper Mindola Clastics and lower Kitwe formations of the Lower Roan Subgroup. The Copperbelt Orebody Member largely comprises a finer 0 to 100 m thick unit of generally carbonaceous argillites, carbonatic argillites and interbedded arenites, underlain and overlain by the coarser, oxidised, clastic successions of the Mindola Clastics and Kitwe formations respectively, on the SW fringe of the Kafue Anticline. The 30 to 80 m thick Mufulira ore-bearing sandstones to the NE of the same basement high, are an inferred equivalent of the Copperbelt Orebody Member, and comprises three "Orebody Quartzites", each within a separate upward-fining unit that has conglomeratic sandstone at their bases, followed upsection by sandstone (quartzite), and culminating a mudseam, dolomitic bed or argillaceous quartzite. Mineralisation occurs either: i). predominantly as disseminations within reduced arenites immediately below a capping finer facies bed (e.g., the Copperbelt Orebody Member at Mwambashi B; the mudseams at Mufulira; the Upper Banded Shale at Nchanga); or ii). mainly veins, both parallel and oblique to bedding within a reduced fine facies, and disseminated in sandy interbeds (e.g., the Copperbelt Orebody Member at Nkana-Mindola and Konkola-Lubambe). More than one style may occur at some deposits.
Individual ore zones within this package average 4 to 15 m in thickness (maxima of 15 to 30 m), with Nchanga an exception having an average thickness of 35 m and a maximum thickness of 91 m.
Some 65% of the mineralisation of the Zambian Copperbelt lies within or immediately below the Copperbelt Orebody Member (e.g., Luanshya-Baluba, Mufulira, Nkana-Mindola, Nchanga-Chingola, Chambishi and Konkola-Lubambe) and the inferred equivalent Mufulira ore-bearing sandstones (e.g., Mufulira).
A further 25% of the Zambian Copperbelt ore lies within coarser footwall clastics of the underlying Mindola Clastics Formation (e.g., at the Chibuluma deposits, where ore is capped by sandy argillites within the Mindola Clastics, whilst the Copperbelt Orebody Member and Kitwe Formation are absent, and the Mindola Clastics formation is directly overlain by the Upper Roan Subgroup; at Fitula, Mimbula and Chingola in the Nchanga district, where the ore steps upwards to the north, from the "footwall" Mindola Clastics formation, in the south, before being hosted within the Copperbelt Orebody Member at Chingola-Nchanga; and the Footwall ore below the Southern Ore Body at Nkana).
The remaining 10% of the ore within the Zambian Copperbelt lies within the coarse "Hanging Wall" arenites of the Kitwe Formation, above the main mineralised Copperbelt Orebody Member (e.g., the large deposit within The Feldspathic Quartzite at Nchanga, where it is 15 to 40 m thick, and is capped by the Upper Banded Shale, on the northern end of the string of deposits, where the upward transition described above has passed through the Copperbelt Orebody Member into the "hanging wall");
Lithologically, 60% of the ore in this package is hosted by argillites, and 40% in arkose, quartzites and conglomerates. Ore within this package is largely restricted to the eastern Domes Region in Zambia and southern DRC.
• The 'Lower' and 'Upper' orebody packages in the Mines Subgroup, only found in the DRC (e.g., at the Kolwezi and Tenke-Fungurume deposits), and sometimes the overlying 'Third orebody' position (e.g., Kambove-Ouest), also in the same subgroup. The Lower and Upper orebody packages overlie the red to lilac R.A.T. Subgroup and comprise two 5 to 15 m thick units of grey, laminated, fine-grained, argillaceous and chloritic dolostone to dolomitic siltstones, sandwiching a strongly silica-dolomite altered unit that is 10 to 30 m thick, originally composed of massive, porous reefal stromatolitic dolostones. The Third orebody position is 60 to 100 m above the the Upper orebody package, within carbonaceous massive to laminated dolostones. Where ore occurs in this latter position, the mineralised intervals lower in the sequence are often poor (Pelletier,1964). These rocks were deposited in the post-Lower Roan Subgroup sag phase facies of the Roan Group that transgressed northward from the main rift in Zambia, onto its margins in the DRC. For detail, see the Congolese/Katangan Copperbelt record;
• The Kansuki Formation of the Dipeta Subgroup, also restricted to hosting ore in the DRC (e.g., Mutanda and Deziwa), are part of the Roan Group sag phase facies, deposited at the transition to renewed extension. It comprises a generally >80 m thick sequence, mostly separated from the underlying Dipeta sequence by a structural contact, marked by an evaporite-facilitated breccia-detachment. It is characterised by alternating reefal to intertidal deposition, comprising laminated and massive stromatolitic or arenaceous dolostones, oolitic dolostone, erosional surfaces, intraformational conglomerates and pseudomorphs after anhydrite near the top of the formation. The Kansuki Formation also contains ~765 Ma (Key et al., 2001) mafic lavas, and extensive, 2 to 12 m thick beds of associated pyroclastic flows and volcaniclastic rocks interbedded with dolostones, defining a regional 'volcanic belt'. This sequence is underlain by the R.G.S. Formation, at the base of the Dipeta Subgroup, containing oxidised clastic rocks, similar to those of the R.A.T. Subgroup.
Ore zones are generally thicker than in the other Copperbelt mineralised positions, but with correspondingly lower grades. Thicknesses of ore as defined by a 0.4% Cu cut-off vary from a few, up to 150 m, with grades of ~1.4% Cu and 0.1 to 0.6% Co (e.g., Mutanda and Deziwa).
Where the Kansuki Formation hosts major deposits, it is uncertain if the Mines Subgroup is developed below, although it may only be represented by the normally barren Menda facies. For detail, see the Congolese/Katangan Copperbelt record;
• The Mwashya Subgroup in Zambia (e.g., Kansanshi) and DRC (e.g., Frontier), deposited in the deepening Katangan basin during the main episode of renewed extension and northward migration of the depocentre. The main prospective unit is the central, ~75 to 140 m thick Kafubu Formation, a sequence of finely bedded, grey to dark grey or black, pyritic carbonaceous shales with local sandy, silty and dolomitic interbeds. At Frontier, the normally underlying Lower Roan hosts are absent, and the Mwashya Subgroup represents the first reduced unit overlying an oxidised arenite sequence separating it from the basement. At Kansanshi, the underlying Lower and Upper Roan subgroups are exposed on the margins of the Solwezi Dome, 12 km to the south;
• The Grand Conglomérat of the basal Nguba Group (e.g., Kamoa in the DRC, Fishtie in Zambia, and to a lesser extent at Frontier in the DRC). Mineralisation extends into the lower Kakontwe Limestones at Fishtie. Like the Mwashya Subgroup, the Nguba Group was deposited during the 'renewed extension', as the Katangan Basin continued to deepen and spread outward onto the Kibaran and Irumide basement to the NW and SE respectively, without any underlying Roan Group, other than a thin oxidised conglomeratic wedge of Mwashya Subgroup at Kamoa.
At Kamoa, the Grand Conglomérat comprises a 'basal diamictite package', commencing with 0 to 30 m thick, clast-rich, sandy diamictite, overlain by 0 to 5 m of sandstone-siltstone to lithic greywacke and by 0 to 15 m of clast-poor, silty/muddy and weakly carbonaceous diamictite. This package hosts the bulk of the resource, and it is uncertain how much original pyrite was in the rock, prior to the introduction of the copper sulphides. It is overlain by a 15 to 45 m thick, dark, pyritic, siltstone-sandstone unit, which is predominantly well stratified and laminated, with bedded pyrite and little Cu sulphide. These are overlain by 0 to 900 m of diamictites and pyritic siltstones, and then by the Kakontwe Limestone (For detail, see the Congolese/Katangan Copperbelt, Kamoa and Fishtie records).
• The Kundelungu Group - a number of transgressive, relatively small, but high grade vein deposits are found outside of the main Lufilian Arc in the northern part of the Kundelungu Gulf/Foreland e.g., Dikulushi (1.138 Mt @ 8.11% Cu, 238 g/t Ag) hosted in the Ks-1 Kalule Subgroup); Kapulo group of deposits (4.2 Mt @ 3.4% Cu), 100 km to the NE, hosted within the underlying mid to upper Nguba Group Monwezi Subgroup).
No major stratabound Cu-Co deposits have yet been found (to 2015) higher than the Grand Conglomérat, although late stage pitchblende and uraninite veins penetrate overlying Nguba and Kundelungu group rocks at Shinkolobwe and related deposits both in the DRC and Zambian Copperbelt, while late stage Pb-Zn ore at Kipushi and similar occurrences to the south of the main Cu-Co zone in the DRC (e.g., Kalongwe) are also hosted by rocks at higher stratigraphic positions within the Nguba Group. Late stage Pb-Zn ore is also found in Upper Roan Subgroup carbonate rocks at the Kabwe group of deposit, ~150 km south of the main Zambian Copperbelt.
Sulphur and Sulphates
Anhydrite, dolomite and calcite are common throughout the Roan Group, occurring as coarsely crystalline anhydrite-dolomite beds, patchy to pervasive authigenic cements, replacive grains, nodules (up to several cm diameter), alteration products and as veins. They are most abundant within the Kitwe Formation and Upper Roan Subgroup, where they are probably of evaporitic origin.
Widespread discordant and semi-concordant breccias within the Upper Roan Subgroup have been interpreted to have resulted from the dissolution of extensive evaporites that have been halokinetically remobilised. Based on the thickness of breccias that are thought to represent original evaporite beds, and the absence of diapiric structures, it is calculated these evaporite beds were originally <500 m thick (Selley et al., 2005 and sources cited therein). These appears to be the first significant primary evaporite beds in the Katanga Supergroup of Zambia, and occur in the hanging wall of the main Zambian Copperbelt ore deposits.
However, anhydrite, dolomite and calcite are also present, within non-evaporitic deltaic and shallow marine lithologies of the Lower Roan Subgroup, mainly as authigenic cements (van Eden, 1974), e.g., a). ±30% anhydrite filling pores within the Porous Conglomerate member and other arenites of the Mindola Clastics Formation; b). quartz-dolomite-anhydrite veinlets in the lower Copperbelt Orebody Member argillites of the basal Kitwe Formation; and c). 20 to 30% anhydrite in the Mufulira ore-bearing sandstones. These include the main ore bearing units.
The presence of this assemblage in the non-evaporitic units of the Lower Roan Subgroup, below the only interpreted significant primary evaporitic source, has been postulated to be the product of diagenetic brine reflux (or thermohaline convection), whereby dense Mg-Ca-SO4 brines, sourced from the overlying evaporitic Upper Roan Subgroup, sink to displace lighter fresh pore waters, and precipitate interstitial evaporite minerals (Selley et al., 2005 and references cited therein).
Anhydrite from throughout the lower section of the Katangan Supergroup, both primary evaporites and disseminated sulphates in non-evaporitic units, have sulphur isotope values of 11.0 to 25.6‰, mostly between 14 and 19‰ (Dechow and Jensen, 1965; Claypool et al., 1980; Surman, reported in Annels, 1989; Hitzman and Broughton, unpub. data), compatible with published Neoproterozoic sea water δ34S sulphate values (Hurtgen et al., 2002).
As there is no evidence of a widespread magmatic sulphur source, and as sulphides can be widely observed replacing anhydrite disseminations and nodules, the source of sulphur in the Zambian Copperbelt has been interpreted to most probably be the disseminated anhydrite within the host rocks, derived from sea water sulphate, or sulphate redeposited from evaporite beds during early diagenesis.
There is a marked antithetic relationship between anhydrite and ore, which is taken to reinforce the interpretation that anhydrite may represent an in situ source of sulphur in ore minerals and was 'consumed' in the process of ore formation, to produce sulphides and a dolomite gangue, as described below (Reductants and Redox Boundaries section). Authigenic anhydrite nodules and crystals show progressive to total replacement by ore sulphides and gangue dolomite/carbonates at the lateral transition to ore (Hitzman et al., 2012; Selley et al., 2005; Sweeney and Binda, 1989; Annels, 1974).
However, sulphides of the Zambian Copperbelt ore deposits have irregular intra- and inter-deposit distribution trends and large ranges of δ34S sulphate values, ranging from -18.4 to +23‰ (Dechow and Jensen, 1965).
Never-the-less, trends are evident, e.g., sulphides in late-formed veins commonly have δ34S values heavier than those of earlier disseminated or veinlet sulphides (Annels, 1989; McGowan et al., 2003), locally at the upper range of assumed sea water values (21 to 23‰). Sulphides in late shear zones also may be heavier relative to nearby disseminated sulphides (Dechow and Jensen, 1965; Hitzman, unpub. data). These data suggest that sulphur isotope values became heavier with time (Selley et al., 2005).
Average isotopic fractionation (ΔSO4-sulphides) values for the arenite-hosted deposits are anomalously low, averaging from 5 to 6‰, at three examples, Chibuluma, Chibuluma West and the Nchanga Upper orebody. Fractionations for argillite-hosted deposits are higher, ranging from ~10.8‰ at Chambishi South East, to ~30‰ for the Luanshya deposit (Selley et al., 2005).
Source of Metals
A number of authors have proposed that the copper-cobalt mineralising fluids in the Zambian Copperbelt were derived from leaching by brines of early rift-cycle red beds of the lower Katanga Supergroup (Mindola Clastics Formation), containing mafic volcanic rocks and/or labile mafic detritus (e.g. Brown and Chartrand, 1986; Haynes, 1986; Rose, 1989; Walker, 1989; Jowett, 1989; Kirkham, 1989). However, Hitzman (2000), Selley et al. (2005), Cailteux et al. (2005), Kampunzu et al. (2005) and others agree that these rocks occupy insufficient volume by more than an order of magnitude to supply the volume of copper within the Central African Copperbelt. In addition, they do not have an appropriate composition, i.e., they lack mafic volcanic rocks and/or significant labile mafic detritus.
Kampunzu et al. (2005) estimated the contained copper, within past production and known resources (152 Mt in 80 deposits; Zientek et al., 2013), allowance for undiscovered deposits (~168 Mt; Zientek et al., 2013), plus subeconomic and anomalous copper accumulations between and surrounding these deposits, totalled ~1850 Mt, which would require the leaching of ~25 ppm Cu from a volume of ~1014 m3 (~100 000 km3) of source rock.
To leach this volume of rock, would require the inclusion either a significantly greater portion of the Katangan Supergroup stratigraphy, possibly including basement rocks, or an adjacent thicker basin succession. Selley et al. (2005) conclude that, based on the extent of observed alteration throughout the rocks of the Lower and Upper Roan, and Mwashia subgroups, and of the uppermost basement, metal leaching from these units above and below the level of ore (a thickness of ~1500 m) would be plausible (i.e., over an area of ~ 200 x 300 km).
Selley et al. (2005) suggest leakage of basement fluids via major rift-related fault systems along the basin margin, provides input from a much larger potential metal reservoir (e.g., Annels, 1989). Authors such as Sweeney et al. (1991) have argued that copper occurrences in basement rocks were the most likely source of copper for the deposits of the Zambian Copperbelt. However, the most significant of these, Samba (see Basement mineralisation below) has been shown to be Lufilian in age, and others are due to replacement into weathered basement in the immediate footwall of the main stratabound deposits, while others have similar gangue assemblages to the intrabasinal deposits, and many lie close to the basal unconformity of the Katangan Supergroup. These are therefore likely to be related to passage of fluid from basement during the main Copperbelt mineralising episode, rather than a source of copper (Selley et al., 2005).
Selley et al. (2005) quote estimates of µ values (238U/204Pb) for potential basement source reservoirs from Carr et al. (unpub. 1986) quoted in Sweeney et al. (1991), and analysed by Kamona et al. (1999). Three end-member source reservoirs were considered (after Kamona et al., 1999): a). upper continental crust Pb from Palaeoproterozoic Lufubu Metamorphic Complex schist and granodiorite (µ = 10.38 and 10.21, respectively); b). average crustal Pb for the Neoproterozoic Nchanga Red Granite (µ = 9.756); and c). upper mantle Pb for the intrabasinal gabbros (µ = 9.451). Stratabound chalcopyrite and carrollite from all deposits sampled yielded µ values of between 10.14 and 10.31 (Richards et al., 1988; Kamona et al., 1999). Regionally, these µ values increase southwards from Konkola to Luanshya, both in basement rocks and in Katangan stratabound sulphides from the ores (Carr et al., reported in Sweeney et al., 1991). These data are consistent with derivation of lead in the ore systems from local basement rocks or locally eroded basement sources.
The broad coincidence of cobalt mineralisation and the gabbroic corridor in Zambia was noted by Annels, (1974; 1984; 1989). If a common source for Pb, Cu and Co is assumed, a source from mafic rock is not supported by this Pb isotope data. However, the main Pb phase within these orebodies is paragenetically late, and may not necessarily be directly related to the main Cu-Co phase.
A study by Richards et al. (1988) analysed copper sulphides from stratabound ore and rutile in uraniferous ~500 Ma post-peak metamorphic albitised veins at Musoshi. Rutile from these veins yielded a µ value of 9.7, suggesting that these late veins derived Pb (and possibly also Cu) from a different source than the earlier ore-stage.
Basinal and Ore Fluids and Fluid Inclusions
Greyling (2009) studied fluid inclusion from the Chambishi, Nchanga, Nkana, Mufulira and Konkola deposits of the Zambian Copperbelt to investigate the nature of basinal, early orogenic and late orogenic fluids. The study and results can be summarised as follows:
• Basinal fluids - the earliest fluids documented from the Copperbelt, occurring in quartz veining introduced into the host arkosic sediments immediately after stratabound copper mineralisation, trapping early basinal brines, in addition to later circulating fluids. These brines are estimated to have been trapped under minimum pressure temperature conditions of 350 bar and 120°C and are characterised by three compositionally different fluids:
i). Moderately saline Na-Ca-Mg brine fluids (11.9 to 23.1 wt.% NaCl equiv.) at the Chambishi and Nchanga deposits, trapped at (minimum) temperatures of between 86 and 129°C, containing chloride ligands as their main anionic species. At Nchanga, a few NaCl saturated inclusions were also found.
ii). Low salinity basinal Mg-K rich fluid, with minor NaCl, is found at the Konkola deposit, with a minimum trapping temperature between 132 and 160°C, believed to have been derived from meteoric waters circulating through the basin during the onset of late diagenesis.
iii). MgCl2-KCl brines trapped together with CO2-CH4-N2, believed to have formed from mixing between the end member Mg-K-rich fluid seen at Konkola with volatiles such as CO2-CH4 that were probably derived from the decomposition of hydrocarbons in the host sediments. This fluid contains up to 600 ppm Co and 1680 ppm Cu. The CO2 may have been generated early during the basin maturation at temperatures between 50 and 150°C (catagenesis), with CH4 generated as temperatures exceeded 100°C during metagenesis.
• Early orogenic fluids - considered to be modified basinal fluids that circulated some time after the initial stratabound copper-cobalt mineralisation had formed. These fluid inclusions are hosted in veins which crosscut and occur along bedding planes, and inclusions are typically located along secondary fracture planes that formed during the onset of metamorphism. They have a variety of compositions, with abundant electrolytes in the aqueous phase and a compositionally variable gaseous component. Stable δD and δ18O isotope signatures show an overlap of metamorphic and sedimentary rock signatures, and were trapped at 550 to 800 bar and 153 to 260°C, which corresponds to depths of ~5.6 to 8.2 km in the absence of an additional heat source. Four fluid types were recognised:
i). Na-Ca-Mg-K brine, seen at Chambishi, made up of two distinct populations, namely a low-salinity (~6 wt.% NaCl equiv.) higher temperature (~140 to 210°C) group, considered to have formed from circulating basinal fluids that became increasingly saline and warmer with progressive burial and dissolution of evaporites from the rocks traversed; and a moderate-salinity (~18 to 23 wt.% NaCl equiv.) lower temperature (~130 to 160°C) group interpreted to be the result of devolatilisation reactions linked to the onset of Lufilian regional metamorphism.
ii). Ca-K brine, at Nkana and Nchanga, occurring as aqueous CaCl2-KCl fluids, which at Nkana contains methane hydrate (CH4•5.75 H2O) and solid graphite. CaCl2 is dominant at Nchanga, with only minor KCl, salinities are low (5.1 to 9.2 wt.% NaClequiv.) with minimum trapping temperatures of ~130°C.
iii). Na brine with CO2-CH4-N2, which at Chambishi is a mixed aqueo-carbonic fluid with aqueous phase salinities of 3.4 to 15.8 wt.%
NaCl equiv., with minimum trapping temperatures between 116 and 245°C. At Nchanga, the aqueous phase in mixed H2O-CO2 fluid inclusions are saline and the non-aqueous phase comprises CO2 rich fluids.
iv). Carbonic fluids, which at Nchanga is a pure CO2 fluid containing minor N2. Methane is also abundant at Nchanga, trapped as relatively pure monophase CH4 inclusions. At Chambishi, methane-rich inclusions contain N2 and C2H6, although with increasing metamorphism, the average hydrocarbon molecular weight decreases to that of methane and is considered to have been derived from the decomposition of organic hydrocarbon matter.
• Late orogenic fluids - are different from both previous generations of fluids in composition, temperature and pressure. Fluids of this generation were detected at Mufulira (in quartz±anhydrite veins crosscutting stratabound copper orebodies), Nkana (in quartz-K feldspar veins crosscutting weak mineral banding in disseminated chalcopyrite that was remobilised during late silicification) and Nchanga (at grain boundary junctions in footwall arkose, where inclusions were either re-filled during metamorphism, or secondary inclusions were trapped during the cracking and annealing of the host quartz).
These late orogenic fluids are recognised as being of modified metamorphic origin. They contain a diverse range of solutes and have minimum trapping temperatures significantly higher than the earlier fluids. Those from Mufulira and Nkana in particular, were the hottest and most complex fluids documented by Greyling (2009), containing mixtures of hypersaline NaCl-CaCl2-MgCl2±KCl and NaCl-CaCO3-saturated ±N2 brines. These fluids were trapped at minimum temperatures of 240°C and 1750 bar pressure. They have the highest salinities, the highest levels of Pb, Zn, Cu, Co and Sr of all three generations, and are more oxidised compared to the earlier relatively more reduced basinal fluids. Fluids from Mufulira also contain solid hematite crystals, consistent with the trapping of saline, high temperature, oxidised brines. The anomalously higher Sr (200 to 500 x modern sea water) supports an evaporite-derived origin for the abundant and diverse metal cations in this fluid type. Minimum depth estimates for late orogenic fluids are around 6.61 km. At this depth, if trapped under lithostatic conditions, the temperature gradient would be higher than the current global average, suggesting an additional heat source, possibly related to granitic intrusions. The four main fluids are:
i). NaCl saturated, Ca-Mg-K brine - found in small quantities at Nchanga only, with partial homogenisation temperatures of ~240°C to the vapour phase. Various characteristics suggest these inclusions were initially trapped during early basin evolution, and underwent a higher temperature overprint during orogenesis. They are regarded as re-filled and modified inclusions.
ii). MgCl2-NaCl±CaCl2 brine - detected at Nkana, with minimum trapping temperatures ranging from 98 to 400°C, and low to moderate salinities, indicating trapping occurred during the heating of an early orogenic MgCl2-NaCl fluid, with addition of CaCl2 at higher temperatures.
iii). NaCl-CaCO3 saturated brines with N2 - enclosing solid hematite crystals during fluid trapping at Mufulira at temperatures between 329 and 379°C. The majority of inclusions also contain solid calcite crystals, have the highest salinities and highest temperatures encountered in the Greyling (2009) study. Depressed eutectic melting temperatures of these inclusions suggests the presence of LiCl, CaBr2, FeCl2, FeCl3 and ZnCl2, with nitrogen as the dominant vapour phase constituent. This oxidised, neutral-to-alkaline (pH <5) NaCl-saturated fluid is regarded as being in equilibrium with the host rocks and capable of carrying high copper- and cobalt hydroxides and oxides.
iv). Low salinity CaCl2-MgCl2-H2O±CO2-CH4 fluid - found at Mufulira, and may either be indicative of meteoric waters, possibly circulating within the basin after peak metamorphic activity, or derived from metamorphic fluids.
Selley et al. (2005) similarly observed that salinities of fluid inclusions and homogenisation temperatures increase from:
• 11 to 21 wt.% NaCl equiv. and homogenisation temperatures of 110 to 170°C, from diagenetic early mineralisation (chalcopyrite-bearing carbonate nodules and subconcordant veins at the Chambishi and Chambishi South East deposits; Annels, 1989), to
• 21 to 38 wt.% NaCl equiv. and homogenisation temperatures of 155 to 185°C, from folded veins cutting stratabound ore (unmineralised, high-angle and deformed quartz±carbonate-K-feldspar-pyrite veins cutting the Lower and Upper orebodies, at the Nchanga deposit; McGowan, 2003), to
• ~39 wt.% NaCl equiv. and homogenisation temperatures of ~400°C at Musoshi, for a late, ~500 Ma, post-peak metamorphic albitisation event associated with quartz-carbonate veining, which is in places accompanied by Cu-U-Mo-(Au) mineralisation (Richards et al., 1988).
This trend indicates an increase in salinity and temperature with time. These fluid inclusions are the product of reactions between three components, namely the sulphur source (most likely authigenic sulphate in the Zambian Copperbelt), a reductant (either in situ or mobile) and the copper bearing fluid (an oxidised brine), rather than representing a single ore fluid.
Davey, Roberts and Wilkinson (2020) presented microthermometric, scanning electron microscopy and laser ablation-inductively coupled plasma-mass
spectrometry analyses of fluid inclusions from the Nkana-Mindola deposits in Zambia. From this data they found that base metal concentrations vary by one to two orders of magnitude between 'barren' and 'ore' fluids, with concomitant distinctions in major salt chemistry. Primary fluid inclusions, that were hosted in pre- to syn-kinematic mineralised quartz veins, were found to be characterised by high homogenisation salinities and temperatures of ∼200 to 300°C, with K/Na of >0.8 and elevated metal concentrations of 102 to 103 ppm Cu and Co. Conversely, they found that barren, post-kinematic vein quartz contained lower homogenisation temperature of ∼110 to 210°C and lower-salinity primary inclusions, characterised by K/Na of <0.8 with low metal contents of <102 ppm Cu and Co. This implies the fluids responsible for mineralisation in the Zambian Copper Belt were high-K brines, anomalously enriched in Cu, Co, Pb and Zn. The elevated base metal concentrations are attributed to the enhanced leaching efficiency of high-temperature, potentially sulphate-deficient, residual bittern brines that had long residence in the deep basin prior to Lufilian/Pan-African orogenesis. These high-K brines are regarded to be the result of progressive enrichment of K in residual brines as halite (and gypsum) is removed by precipitation. This is supported by low Cl/Br and Na/Br ratios in sulphide hosted inclusions obtained from several Central African Copperbelt deposits by Selley et al. (2018) which they claim is characteristic of bittern brines produced by extensive evaporation in a restricted environment. Davey, Roberts and Wilkinson (2020) also suggest the enrichment of K may have been exacerbated by temporal overlap with the inferred up to 200 m.y. period of CaCl2-rich and MgSO4-poor seas during the middle Neoproterozoic (e.g., Kah et al., 2004; Kovalevych et al., 2006; Spear et al., 2014). In sulphate poor brines, precipitation progresses direct from halite to sylvite (KCl), illustrating the availability and release of K after NaCl dissolution (e.g., Bonneville Salt Flats, Utah, USA; Valyashko, 1962).
Reductants and Redox Boundaries
Mineralisation within the Zambian Copperbelt is closely associated with stratigraphic or structural redox boundaries in the host units described above, related to rocks that may have contained reductants and that overlie coarse, permeable, oxidised arenites. The redox boundaries are related to reductants that may be either: i). in situ, largely occurring as relatively dark and locally carbonaceous siltstones and argillites, often with fine arenite interbeds; or ii). mobile e.g., sites that are geometrically favourable to the entrapment of liquid hydrocarbons and/or sour gas.
The layers that contain in situ hydrocarbons are almost always also aquitards that cap sites that are geometrically favourable to the entrapment of mobile hydrocarbons, and most likely were impregnated from below with the latter also, particularly within coarser interbeds and fractures.
These reduced rocks had two roles in the formation of Cu-Co deposits in Zambia: i). they acted as a redox boundary, whereby oxidised, sulphur deficient Cu-bearing brine, which is in equilibrium with footwall oxidised arenites, is reduced on contact with the reductant, drastically decreasing the solubility of Cu, and resulting in its release from solution; and ii). they contributed carbon to the reaction that produced sulphides and carbonate gangue, as explained below, particularly in the case of mobile hydrocarbons, such that remaining pyritic, carbonaceous rocks, with few exceptions, form a barren cap and or mark the lateral margins to ore, as well as in some cases, a barren footwall, but do not remain within the ore zone.
Of the major deposits, Luanshya, Nkana-Mindola, Chambishi, Konkola-Lubambe and in part Nchanga, are hosted by the Copperbelt Orebody Member which is taken to represent a unit that predominantly contained in situ hydrocarbons. Deposits of this type are laterally extensive with strike lengths of up to 17 km. The deposits hosted by Mwashya Subgroup (e.g., Frontier and Kansanshi) and Nguba Group (Fishtie in Zambia and Kamoa in DRC) are also most likely associated with in situ hydrocarbons.
Deposits interpreted to be related to accumulation of mobile hydrocarbons are usually those hosted by arenites which occur in both the footwall, hanging wall and equivalents of the Copperbelt Orebody member, and have maximum strike lengths of 5 km. Deposits of this type include Mufulira and much of the Fitula-Mimbula-Chingola-Nchanga string of deposits, as well as Mwambashi B and the Chibuluma deposits.
No direct evidence of mobile hydrocarbons has been recognised, e.g., pyrobitumen, although solid graphite has been observed at Nkana (Greyling, 2009), interstitial carbonaceous matter in the arenites hosting orebodies at the Mufulira deposit, and the strongly depleted carbon isotope signature of carbonate gangue at the Mufulira and Mwambashi B deposits are taken as indirect evidence for the involvement of hydrocarbons in the mineralising process (Selley et al., 2005).
On the eastern margin of the Kafue Anticline, a thick carbonaceous arenite unit, occurs at the same stratigraphic position as the Mufulira arenite hosted ore deposit, and forms the lateral gradational margin to mineralisation. The carbonaceous matter, which is finely disseminated throughout this sandstone suite, is interpreted to have originated as mobile hydrocarbons based on δ13C and δ18O values (see below; Annels, 1979; 1989). It transgresses bedding and is continuous over a strike length of >16 km, capped by less permeable shale and dolomite interbeds. Similarly, the string of deposits from Fitula, through Chingola and Nchanga gradually climbs through the sequence from the Mindola Clastics Formation in the south, to the hanging wall of the Copperbelt Orebody member of the Kitwe Formation 18 km to the north, much of which is hosted by arenites, capped by argillite bands.
The location, geometry and size of deposits, particularly those related to mobile hydrocarbons are fundamentally controlled by early sub-basin fault architecture and the supply of the reductants, which are also linked to basin architecture (Selley et al., 2005). The most common interpreted trap sites for mobile hydrocarbons include: i). where the argillites of the Copperbelt Orebody member lap onto basement up-dip, over a pinchout wedge of Mindola Clastics formation rocks; ii). early Lufilian antiformal culminations, as postulated at Nchanga and iii). culminations resulting from differential compaction of sediments over, or lapping onto a localised composite basement high, as at Mufulira, due to a lesser decrease in thickness of thinner sections over underlying basement hills (usually of granite), compared to the greater cumulative reduction in thickness over intervening basement lows (usually over Lufubu schists).
Mobile hydrocarbons are generated when organic rich rocks (usually argillites containing 4 to 20 wt.% total organic carbon) are heated to ~60°C, when petroleum begins to form in the source rock by the thermogenic breakdown (cracking) of organic matter (kerogen) known as maturation. The temperature dependant oil window, or interval over which oil is generated and expelled from the source rocks, is ~60 to 120°C. The succeeding gas window occurs over the temperature range of ~100 to >200°C. These temperatures are usually the result of burial, which at the typical thermal gradient of ~30°/km, is 2 to 4 km for the oil window and 3 to 6 km for the gas window. In addition, large intrusions may supply sufficient heat to complement the thermal gradient to reach the required temperature window at shallower depths.
Consequently, where mobile hydrocarbons are the indicated redox agent, mineralisation did not take place until the organic rich source rocks had been buried to sufficient depths to promote maturation. If those source rocks were from the Katanga Supergroup, such a thickness was not achieved in the immediate Zambian Copperbelt until late in the deposition of the Kundelungu Group during the early Lufilian Orogeny. If the hydrocarbons were formed in a deeper core of the Katanga Basin to the SW (or NW), where the host sequence may have been thicker, and then migrated up dip, this could have occurred earlier, most likely during deposition of the Nguba Group. This corresponds to the period of 'renewed extension' and deepening of the Katanga basin, when deeper water, more reduced equivalents of the Roan or Lower Nguba groups were buried and dropped to well below the level of the basin margins, and may have acted as source rocks. This would include potential source rocks such as the carbonaceous argillites of the Copperbelt Orebody Member and the Mwashya Subgroup. The extensive gabbro corridor rocks in Zambia and coeval mafic volcanic rocks in DRC, as described above, which were emplaced during the 'renewed extension' and deposition of the Mwashya Subgroup and Nguba Group, may also have contributed to maturation of source rocks. However, fluid inclusion studies by Greyling (2009) show minimum trapping temperatures of 120°C for fluids circulating at the time of diagenetic ore formation suggesting the presence of such a heat source.
Alternatively, potential source rocks in the Mesoproterozoic Irumide and Kibaran sequences would have passed into the 'oil window' earlier in the deposition of the Roan Group sequence and migrated up section along extensional structures. Whichever may have occurred, mobile hydrocarbons would not have been introduced to the host sequence until late- or post-diagenesis, after lithification had commenced, when porosity and permeability were facilitated by both interstitial/intergranular space that remained open, and early brittle fracturing.
Throughout the Zambian Copperbelt, the most carbonaceous rocks within an ore horizon are commonly not economically mineralised, i.e., there is an antithetic relationship between carbonaceous rocks and ore. This implies the carbonaceous reductant is 'consumed' during the ore forming process, as explained below. Also, where deposits occur in the stratigraphically lowermost reduced rocks (e.g., the Copperbelt Orebody Member), any overlying reduced or favourable rocks (e.g., the Mwashya Subgroup) were generally not mineralised. Conversely, deposits within higher stratigraphic level reduced rocks (e.g., the Mwashya Subgroup) are found where underlying reduced units are absent.
The antithetic relationships between copper sulphide mineralisation and both carbonaceous material and authigenic anhydrite (described above under Sulphur and Sulphates) within host units, suggests both carbon and anhydrite were an integral part of the formation of ore. Mobile hydrocarbons within a hydrocarbon reservoir react with authigenic anhydrite cements (using methane as a generic hydrocarbon), to produce H2S (sour gas) in the first instance: CaSO4 + CH4 → CaCO3 + H2S + H2O. The second step involves interaction with a Cu-bearing brine, with a most likely chloride ligand, as follows:
H2S + 2 CuCl → Cu2S + 2 HCl.
In the event of a Cu-bearing brine reacting with in situ carbonaceous material and entrained authigenic anhydrite cements, the process becomes: CaSO4 + CH4 + 2 CuCl → Cu2S + 2 HCl + CaCO3 + H2S + H2O.
However, in both cases, the HCl produced breaks down the CaCO3 and reverses the process. But, where excess Fe, Mg or Mn ions are present within the brine, the HCl is neutralised and the process becomes: CaCO3 + (Mg/Fe/Mn) + HCl → CaCl + (Mg/Fe/Mn)CO3 + H2O (Orr 1974 and Irwin, et al., 1977). This process destroys both the anhydrite and carbonaceous matter, where ore is formed, and produces a gangue of dolomite, ankerite, siderite, etc., deficient in free carbon and anhydrite, as is observed.
These formula represent the simplified process from ingredients to final products. However, it is apparent that the process took place in a chain of reactions from early pyrite, through chalcopyrite, bornite to chalcocite, as outlined in the Paragenesis and Zoning sections below.
Annels (1989) recognised that light δ13C and δ18O values can be the result of temperature effects during hydrothermal alteration, from the incorporation of isotopically light C and O, produced by oxidation of organic matter (in situ or mobile hydrocarbons), into secondary carbonates during low-temperature (~60 to 80°C) bacterial sulphate reduction, or from high temperature (>100°C) thermochemical sulphate reduction as in the processes outlined above.
Selley et al. (2005) collected δ13C and δ18O data from 369 carbonate samples (whole rock, veins and evaporite nodules) from 10 deposits and one regional drill hole, spanning the stratigraphic interval from rocks of the Mindola Clastics Formation to those of the Mwashya Group. These data revealed that samples from both ore zones and the immediate hanging walls to ore zones, have consistently light isotopic signatures compared with normal sedimentary values found in primary clastic and carbonate rocks (including 'barren gap' dolostones). Carbonates from the argillaceous rocks of the Copperbelt Orebody Member, and Mindola Clastics Formation contain a broad range of isotopic values (δ13C ~ -26 to +3‰ and δ18O ~ +7 to +23‰). However, strongly depleted isotopic values (δ13C < -14‰ and δ18O <+13‰) are entirely restricted to ore zones. There are a number of possible ways to produce this result, which Selley et al. (2005) discuss, but conclude that the most plausible is oxidation of organic matter to CO2 which then becomes incorporated in diagenetic or hydrothermal carbonates (e.g., Rollinson, 1993; Ohmoto and Goldhaber, 1997; Wilson et al., 2003).
Mineralisation Styles and Textures
Deposits have a range of differing mineralisation styles and textures that may occur together or alone. The chief of these are:
• Disseminated, generally fine-grained (<0.1 to 3 mm) sulphides within interstitial sites between detrital or authigenic grains, randomly distributed or aggregated to form bedding-parallel streaks and lenses of blebs. There is a correlation between the size of detrital and sulphide grains in this style of dissemination (Selley et al., 2005 and references cited therein). Sulphides may also occur replacing detrital grains and authigenic cements, including anhydrite, carbonate, quartz and feldspar, as well as diagenetic pyrite, anhydrite, carbonate, quartz, feldspar or heavy minerals such as rutile (Binda, 1975; Fleischer et al., 1976). Disseminated ores predominate in porous and permeable host rocks such as sandstones, coarse silts or shales with sandy interbeds (Hitzman et al., 2012);
Colour photos of disseminated mineralisation are included in the Mufulira and Nchanga records.
• Vein-hosted, which may occur as prefolding, layer-parallel and discordant veins or veinlets of sulphide, which have sharp contacts with argillaceous strata, and diffuse margins in arenites (Annels, 1989). Most deposits in the Central African Copperbelt contain both disseminated and vein- or nodule-controlled sulphides, although the majority are dominated by disseminated sulphides (Hitzman et al., 2012). Pre-folding veins can consist entirely of sulphides or be accompanied by other minerals, particularly quartz and dolomite, with lesser K feldspar, albite, chlorite, sericite, phlogopite (KMg3AlSi3O10(F,OH)3) and anhydrite (Hitzman et al., 2012).
Colour photos of vein-type mineralisation are included in the Nkana-Mindola record.
Vein-dominated deposits usually occur within strongly carbonaceous host rocks but are still accompanied by lesser economic disseminated mineralisation, e.g., Frontier, Kansanshi, Sentinel and Nkana South Orebody (SOB), which are mainly syn- or post-folding. This late-tectonic veining generally occurs as coarse sulphide grains (commonly >0.5 to 3 cm) in quartz-carbonate (dolomite, calcite) veinlets and subordinate veins, which may also contain K-feldspar, chlorite, sericite, biotite, and, very locally, albite, tremolite, and below the zone of supergene dissolution, anhydrite. Veinlets are typically ~0.5 to 2 cm wide, but may locally be up to several tens of cm thick and extend for distances of several tens of metres or more, with densities of 1 to 5 per metre, following and stepping up through the stratigraphy. Veins also occupy tension gashes on a small scale and other structures at a high angle to bedding. Veins and veinlets can be particularly abundant at the tops and/or bottoms of the stratabound orebodies, commonly coinciding with rheologic contrasts and occupying shear zones and décollements at the boundaries between more argillaceous and siliciclastic rocks. Veinlets often penetrate upwards from arenite hosted disseminated mineralisation into otherwise unmineralised, impermeable carbonaceous shales.
Mineralised veins are restricted to zones of disseminated mineralisation, and contain the identical sulphide assemblages found within the enclosing disseminated mineralisation throughout the zoned sulphide assemblages (Hitzman et al., 2012, and references cited therein; Sillitoe et al., 2010).
In addition to these Cu±Co vein systems, the Zambian Copperbelt contains volumetrically minor Cu-U-Mo-(Au)-(Ni) sulphide assemblages in post-folding veins with associated albite haloes, e.g., Mindola, which is the only known economic accumulation (Darnley et al., 1961). Generally sub-economic uranium occurs as late veins and local disseminations of pitchblende, uraninite, brannerite, coffinite and secondary oxide minerals below or adjacent to copper mineralisation (Selley et al., 2005).
• oxide ores, usually gradationally overlying the hypogene mineralisation, through a progression from primary sulphide (chalcopyrite-bornite-carrollite), through mixed oxide-sulphide (chalcocite-copper carbonate/sulphate-cuprite-native copper) to leached capping (usually ~30 m thick). The transition usually takes place over an interval of 30 to 70 m below the surface, although in some areas of deep faulting, malachite and chalcocite have been found to persist to depths of as much as 1 km at Konkola and >600 m at Nchanga Lower. As chalcocite generally occurs closest to the surface in the primary sulphide zonation, it is often difficult to differentiate between primary and supergene populations of the mineral (Selley et al., 2005).
• cupriferous mica mineralisation, which occurs at a number of deposits as an upper and/or lower fringe to sulphide orebodies, but never laterally. At the Mimbula-Fitula deposits, cupriferous micas persist to a depth of up to 150 m, although the bulk is in the zone from near surface to a depth of 60 m. The cupriferous micas are brown to dark brown in colour, and are visually indistinguishable from unmineralised mica. Stockpiles of as much as 150 Mt @ 1.2% Cu have been accumulated at Chingola, and resources of in situ mineralisation and stockpiles at Nchanga are >275 Mt @ 1 to 1.4% Cu. The mineralisation is refractory. Copper is held in the mica lattices in the exchange position, tied largely to hydroxyl groups in interstratified phlogopite-cupriferous chlorite micas. Some copper is also absorbed on the surfaces of layered mineral structures. Under tropical or sub-tropical conditions of weathering, it has been shown that copper may be dissolved out of primary minerals, and where the adjacent rock is micaceous, Cu is 'soaked up' by the micas as it passes through the rock. Weathering of primary phlogopite causes exchanges of K ions by hydrated Cu and Mg ions, followed by chloritisation. The Cu content of this mineralisation ranges from 1 to 8% Cu (Diederix 1977).
Alteration
Prolonged, repeated, multiple stages of alteration are evident within the Zambian Copperbelt, ranging from diagenetic overgrowths, to post-metamorphic vein selvedges, reflecting the similarly prolonged history of Cu mineralisation. Three dominant alteration types are widespread throughout the Copperbelt, controlled partly by lithology and indicative of the large scale passage of basinal brines. These are (after Selley et al., 2005 and references cited therein):
• Calcic-magnesian - an early diagenetic to early lithification, dominantly pre-ore, alteration suite, characterised by anhydrite, dolomite, calcite and later development of phlogopite. Anhydrite, dolomite and calcite are common throughout the Roan Group, as described above in the Sulphur and sulphates section, e.g., a). ±30% anhydrite filling pores within the Porous Conglomerate member and other arenites of the Mindola Clastics Formation; b). quartz-dolomite-anhydrite veinlets in the lower Copperbelt Orebody Member argillites of the basal Kitwe Formation; and c). 20 to 30% anhydrite in the Mufulira ore-bearing sandstones. There is a marked antithetic relationship between anhydrite and ore, as described above in the Sulphur and sulphates section.
The widespread development of phlogopite (KMg3AlSi3O10)(F,OH)2), rather than biotite, particularly in argillites, is suggested by Selley et al. (2005) to represent later isochemical metamorphism of previously pervasively magnesian metasomatised rocks throughout the succession. Within the Upper Roan and Mwashya subgroups, and the lower Nguba Group, above the main evaporite horizons, local significant albitisation and silicification accompanies the calcic-magnesian phase.
• Potassic - characterised by K feldspar and sericite-muscovite, which is most pronounced within formerly argillaceous strata, i.e., siltstones, shales and, to a lesser extent, argillaceous arenites. The feldspar content of detrital rocks within the Lower Roan Subgroup is virtually 100% potassic, at variance with the high sodic feldspar content of the source basement rocks. This and textural features of the rocks (e.g., the partial to total replacement of plagioclase detrital grains), are interpreted to be the result of intense K metasomatism of the Lower Roan Subgroup, below the first salt/evaporite beds in the Upper Roan Subgroup. Textures indicate multistage K feldspar replacement which took place from diagenesis (as pre-anhydrite-carbonate overgrowths) to orogenesis (vein-hosted K feldspar). Secondary K feldspar is a major gangue mineral in many Zambian Copperbelt deposits, typically intergrown with Cu-Co sulphides, particularly in argillite hosted deposits where sulphides and K feldspar are intergrown in coarse clots throughout the detrital network. In addition, there is a concomitant increase of the K2O:Al2O3 ratio and Cu within rocks of the Copperbelt Orebody Member. However, elevated K2O:Al2O3 ratios persist for hundreds of metres into the hanging wall of mineralisation and for kilometres laterally beyond the margins of ore (Selley et al., 2005), including sections of the Upper Roan and Mwashya subgroups. Potassic alteration is represented by sericite, in close association with Cu-Co mineralisation, at the expense of K feldspar (and Ca-Mg-bearing minerals), in a number of (but not all) deposits, particularly those hosted by arenites, as described below.
• Sodic - late-stage, structurally controlled alteration, usually as vein-associated, albite and/or scapolite. Secondary albite alteration dominates in rocks above the evaporite horizons in the Upper Roan and Mwashya subgroups and the lower Nguba Group. However, it is more erratic and relatively rare in the Lower Roan Subgroup, occurring in some 'footwall' arenite-hosted deposits (e.g., Chibuluma), where the Upper Roan section containing breccias interpreted as after evaporites, has been stepped down to be juxtaposed with the Mindola Clastics Formation. It is generally volumetrically subordinate to preexisting K-feldspar in the lower Roan Subgroup.
In Upper Roan Group strata, intense albitisation is mainly found in brecciated rocks, where coarsely crystalline matrix albite is in apparent textural equilibrium with dolomite, anhydrite, talc, tremolite, phlogopite, and accessory hematite and rutile. Breccias clasts are also thoroughly albitised. Many, but not all breccias contain albitised gabbroic bodies, suggesating a maximum age constraint on Na alteration within the breccias. Albitization also occurs as alteration halos around postfolding veins in rocks of the Mwashia and Lower Kundelungu groups at the Frontier and Kansanshi deposits (Broughton et al., 2002; Broughton, unpub. data, 2004).
Within the Zambian Copperbelt, sodic alteration is associated with late events, e.g., as a halo to discordant, post-folding, barren to sub-economic vein-fracture zones superimposed on the stratabound ore, as at Chambishi and Musoshi, but also as albite associated with an increase in economic mineralisation elsewhere in the same deposits, associated with shearing at the base of the Copperbelt Orebody Member. Albite is developed as a partial replacement of secondary K feldspar and sericite, and occurs both in equilibrium with, and overprinting, Cu-Co sulphides at different deposits.
Scapolite, predominantly marialite (3NaAlSi3O8•NaCl), is widespread and abundant in most marbles, calc-silicate and metagabbroic rocks in the Lufilian-Zambezi belts in Zambia, but may also occur as pervasive replacement of plagioclase in granite gneiss and amphibolites (Mendelsohn, 1961; Cosi, et al., 1992).
A late, post-peak metamorphic paragenesis is indicated, where geochronological and geological data is available, for some vein and fracture-related albite alteration, such veins contain molybdenite (e.g., Chambishi Ore Shale, Jolly, 1971; or Musoshi Ore Shale, Lefebvre and Tshiauka, 1986; Richards et al., 1988), or that introduce late U-Mo-Cu-(Au) (e.g., Mindola, Darnley et al., 1961). Veins of this type in the footwall of the Musoshi orebody were dated at ~514 Ma (Richards et al., 1988). Albite alteration is associated with post-folding quartz-dolomite-chalcopyrite ore veins at the Kansanshi deposit (dated at ~510 Ma; Torrealday et al., 2000) and is the main alteration phase at the Frontier deposit (Broughton, unpub. data, 2004).
• Regional IOCG-style - comprising sodic and sodic-calcic (albite-actinolite-scapolite) alteration that overprints the pervasive potassic altered rocks of the region, as does regionally distributed occurrences of Cu, Cu-Au, Au, magnetite/hematite and U. The sodic stage described above, is part of this regional alteration. This alteration and mineralisation occurs in the following regions:
a). the Katanga Core, centred on the syn- to post-tectonic ~560 to 530 Ma Hook Granite complex, and include the Mumbwa/Kitumba Cu deposit (Resources of 38.8 Mt @ 2.19% Cu at a 1% Cu cutoff; Blackthorn Resources website, 2012), Kalengwa, (1.9 Mt @ 9.44% Cu, 50 g/t Ag, which has undergone supergene enrichment; Lobo-Guerrero, 2010) and the Kasempa Fe deposit (~230 Mt @ 66% Fe in 10 deposits; Nisbet, et al., 2000). These deposits are mostly associated with vertically oriented, structurally-controlled magnetite-hematite-rich breccia zones in Nguba Group sedimentary rocks, and in 'Hook' granites and syenites (Hitzman, 2001). Gabbro, as well as syenite is also found at some of these occurrences. At Mumbwa, potassically altered rocks are cut by magnetite, which is in turn replaced by hematite during a hydrolytic (sericite-chlorite) alteration event, overprinted by a final sulphidation event resulting in the precipitation of pyrite and then chalcopyrite (Lobo-Guerrero, 2010; Hitzman, 2001);
b). the Domes Region and Inner External Fold and Thrust Belt, extending from southern DRC and western Zambia, to and overlapping the southern Kafue Anticline. This belt of Fe, Cu, Au and U occurrences forms an inner arc, SW of the Zn-Pb zone (e.g., Kipushi and Kalongwe) and the outer main Lufilian Arc Zambian and Congolese copperbelts Cu-Co deposits further to the NE and east. Many of the iron occurences in this belt comprise magnetite and hematite occurring as cements to hydrothermal breccias and as fracture fillings, with or without sulphides (e.g., Lobo-Guerrero, 2010). Massive to semi-massive magnetite bodies are also associated with mafic intrusive stocks or sills (gabbros) which have undergone extreme sodic alteration, resulting in the formation of albite-actinolite-scapolite assemblages (e.g., in the northern and western parts of the Nkana-Chambishi Basin, where a ~170 m thick metagabbro sill is underlain by ~52 m of granophyre, ~8 m of magnetite rich rock and a further 35 m of gabbro, all enclosed within Upper Roan Group carbonates; Garlick, 1961). Others are the stratabound magnetite-hematite lenses and units interbedded with the mafic volcanic rocks of the Dipeta Subgroup Kansuki Formation (previously the Lower Mwashya Subgroup) and equivalent uppermost Upper Roan Subgroup (e.g., Kasumbalesa Fe on the DRC-Zambia border, 80 km SE of Kipushi), which are also found in the outer External Fold and Thrust Belt in the Cu-Co zone, but not directly related to the regional sodic-calcic alteration (Cailteux et al., 2007).
Hitzman (2001) notes that Cu-(U-Au) deposits associated with sodic alteration (e.g. Kansanshi; >730 Mt @ 0.86% Cu, 0.14 g/t Au) in this part of the Lufilian arc, generally lack significant iron oxides, although the intense sodic alteration is somewhat similar to that observed in many magnetite-apatite systems (Hitzman, 2001).
c). The Irumide Belt, south of the Kafue Anticline, where the Fishtie (55 Mt of 1.04% Cu at a 0.5% Cu cut-off; Hendrickson, 2014) deposit is located. At Fishtie, stratabound, replacement magnetite-ankerite-apatite-quartz mineralisation hosted by the Grand Conglomérat of the basal Nguba Group, was overprinted by later hydrothermal alteration and sulphide mineralisation. The stratabound early iron phase tapers outwards from steep syn-sedimentary faults. The overprinting sulphide and Cu mineralisation is spatially closely controlled by, and is not extensively developed distal to, the same faults, but is zoned relative to them, from proximal bornite, outward to ore stage pyrite with chalcopyrite. This deposit has characteristics of both IOCG and sediment hosted mineralisation, but lacks a thick siliciclastic palaeoaquifer beneath the Grand Conglomérat Formation as is present at the stratabound Kamoa Cu-Ag deposit, which is in a similar setting in the DRC (Hendrickson et al., 2015);
d). the Zambezi Belt, south of Lusaka, where plug-like and tabular bodies of magnetite and hematite, some of which carry disseminated sulphides, are associated with hydrothermal breccias and syenitic and gabbroic intrusive bodies (Lobo-Guerrero, 2010; Burnard, et al., 1993).
These observations suggest two IOCG events occurred in the broader Lufilian Arc, one during the 'renewed extension' (~765 to 736 Ma) and the second during the late Lufilian orogenesis (~560 to 500 Ma), both of which contributed fluids that produced either IOCG sensu stricto deposits, or overprinted earlier sediment hosted copper mineralisation, or produced hybrids with the characteristics of both styles.
• Deposit scale alteration - In many Zambian Copperbelt deposits, secondary potash feldspar is a major gangue mineral (e.g., Mendelsohn, 1961), where it is typically intergrown with Cu-Co sulphides. Ore sulphides in argillite-hosted deposits, are commonly intergrown with secondary K feldspar as coarse-grained clots disseminated throughout the detrital framework, and within former anhydrite nodules. However, this alteration is not restricted to ore and may occur for many hundreds of metres into the hanging wall (Hitzman et al., 2012).
Croaker (2011) records the main metamorphosed assemblage in the altered Copperbelt Orebody Member at Nkana-Mindola on the Zambian Copperbelt, comprises calcite-dolomite-quartz-phlogopite-sericite-tremolite±albite-talc-anhydrite-scapolite. In lower strain areas, the assemblage observed includes a faint banding of siliceous dolomite and micaceous argillite. The carbonate bands comprise 50 to 60% dolomite-calcite, 30% quartz and 10% phlogopite, while the micaceous argillite is composed of 40% quartz, 20% dolomite-calcite, 15% sericite, 15% phlogopite and 10% albite. This assemblage may include both earlier potassic and overprinting sodic alteration phases.
At a number of (but not all) Zambian Copperbelt deposits, particularly those hosted by arenites (e.g., Mufulira), Cu-Co mineralisation formed in close association with sericite, at the expense of K feldspar (and Ca-Mg-bearing minerals). This has been taken to reflect evolving conditions in the potassic environment, resulting from a more acid phase, possibly associated with H2S in sour gas within the sandstones, and/or HCl generated from the reaction between Cu-bearing fluids and anhydrite, and/or the progressive enrichment of Cu in sulphide minerals (see the reactions outlined in the Zoning section below). In these deposits, sericite is much more closely associated with the ore mineralisation than K feldspar alteration is elsewhere. Sericite is also localised along faults and shear zones at a number of deposits (Selley et al., 2005).
In comparison, in the dolomitic shale hosted Congolese Copperbelt deposits of the Mines Subgroup, alteration commences with a potassic phase, resulting in the formation of K feldspar in argillaceous and siliciclastic rocks. This is followed by several generations of silica-dolomite alteration, occurring as magnesite and/or dolomite and silica (or Mg-chlorite in more argillaceous rocks), with fine-grained quartz±dolomite accompanying Cu-Co ore, followed by late coarse-grained, disseminated, and vein-controlled dolomite±quartz±Cu-Co sulphides (Hitzman et al., 2012). For more detail see the Congolese/Katangan Copperbelt record.
Paragenesis
Croaker (2011) determined the following paragenesis for the Nkana deposit in Zambia:
• Pyrite - the earliest sulphides are a disseminated pyrite phase, which is interpreted to be of late diagenetic origin, typically replacing earlier diagenetic anhydrite and/or dolomite (Annels, 1989).
Diagenetic framboidal pyrite is widespread in both Zambian and Congolese copperbelts, but is not always accompanied by Cu-Co ore (Sweeney and Binda, 1989; Dewaele et al., 2006). Croaker (2011) identified a number of pyrite types in the Chambishi Basin deposits. These included pyrite grains hosted within black carbonaceous argillite, with 'spongy' cores, and the isotopically lightest sulphur signatures, at the poorly deformed Chambishi SE deposit, 10 km to the north, interpreted as typical of early diagenetic origin. At the more heavily metamorphosed Nkana deposit, fine grained (~0.1 mm or less) disseminated pyrite is observed in low strain zones, interpreted to be late diagenetic. This is suggested by the rotation of some equivalent, but coarser (0.2 to 10 mm), inclusion free pyrite porphyroblasts, with quartz-calcite strain shadows in high strain domains, suggesting they are pre-tectonic. In addition, a further variety of disseminated pyrite with inclusions of mica laths aligned parallel to the regional structural foliation is taken to be syn-tectonic.
Similarly, late diagenetic (pre-kinematic), syn-tectonic and late-tectonic (or recrystallised) pyrite veins were identified, based on their structural relationships. These later generations may not represent introduction of new pyrite, but recrystallisation and the localised deformation and remobilisation of the sulphides on the scale of centimetres to metres within the confines of the stratabound copper ore envelope. Locally pyrrhotite dissolves and partially replaces pyrite along cleavage and fracture planes in the high strain domain.
• Copper sulphides - predominantly chalcopyrite, with lesser bornite and carrollite. An early phase of chalcopyrite and carrollite overgrows and/or replaces pyrite, whilst chalcopyrite locally displays myrmekitic, vesicular and exsolution textures with bornite. A second generation of chalcopyrite-bornite frequently overgrows carrollite, or encloses fractured carrollite. A volumetrically minor late stage of carrollite, confined to the high strain domain, overgrows both chalcopyrite and bornite. A minor bornite phase may contain exsolved or pseudomorphic intergrowths of chalcocite. Chalcopyrite and bornite have been recrystallised and locally remobilised during peak deformation and metamorphism and are commonly aligned parallel to cleavage and intergrown with, or overprinted by, silicate minerals.
These generations are represented in both disseminations and veins of late diagenetic, syn-tectonic and late-tectonic origin. Croaker (2011) notes that whilst these relationships may be interpreted to indicate syn-orogenic introduction of metals, the existence of disseminated sulphides in more poorly deformed parts of the orebody that are truncated/overprinted by cleavage, is considered to be evidence of at least some pre-existing sulphide assemblages prior to deformation which was remobilised.
• Accessory sulphides - sphalerite occurs as anhedral grains within black carbonaceous shales, commonly on the margins of chalcopyrite grains aligned parallel to cleavage. Molybdenite occurs as rare 10 to 30µm subhedral grains, associated with veins parallel to and crosscutting cleavage, commonly intergrown with chalcopyrite and bornite.
• All mineralised veins are confined to the stratabound, folded copper orebody envelope and regardless of their generation, contain a similar sulphide and gangue assemblage to the host lithology.
Zonation of Ore Minerals
There is a zonation of minerals, on both a deposit and local scale reflecting the paragenesis. At the deposit scale, there is a gross mineral zonation from hematite in oxidised footwall rocks to a chalcocite-bornite assemblage in immediately adjacent or overlying reduced rocks, particularly those adjacent to syn-sedimentary faults. The chalcocite-bornite assemblage then grades stratigraphically upward and/or laterally away from the footwall or syn-sedimentary structures, to a bornite-chalcopyrite assemblage, then a chalcopyrite-rich assemblage, a chalcopyrite-pyrite assemblage and, finally to pyrite (Hitzman et al., 2012). This zonation reflects the flow of Cu-bearing ore fluids, from chalcocite-bornite proximal to pyrite distal.
On a finer scale, zonation may occur between individual beds, being relatively simple where host rocks lack significant compositional layering, to complex and overlapping where bedding is well developed (e.g., the Mines Subgroup orebodies), and fluids are contemporaneously penetrating along and outwards from closely spaced layers.
Individual grains of sulphide may also display sequential, concentric replacement textures, mimicking the observed broader scale mineral zonation. Similarly, the transition from early to late-formed sulphides is reflected by identical zoning in disseminated sulphides, veins and nodules (Brummer, 1955; Mendelsohn, 1961; Richards et al., 1988; Selley et al., 2005; Sillitoe et al., 2010). The complex and overlapping mineral zonation observed in some deposits, particularly those of the Mines Subgroup in the DRC, could reflect either multiple fluid ingress events, controls on sulphide speciation by local host-rock variability, or both (Hitzman et al., 2012).
The earliest stage pyrite may be formed during early diagnesesis in anaerobic tidal marsh conditions, from the reaction between iron oxides, organic matter and sea water sulphate, as follows: Fe2O3 + 4 SO42- + 8 CH2O + 0.5 O2 → 2 FeS2 + 8 HCO3- + 4 H2O (Fanning et al., 2012). Alternatively it may be formed from sour gas generated by the reaction between mobile hydrocarbons and authigenic anhydrite, as follows: CaSO4 + CH4 → CaCO3 + H2S + H2O. The H2S then reacts with iron oxide in the reservoir rocks in a two stage process to first produce the monsulphide FeS (Mackinawite): Fe2O3 + 3 H2S → 2 FeS + S + 3 H2O which then reacts further with H2S to produce the more stable pyrite: FeS + H2S = FeS2 + H2 (Schoonen, 2004). The two reactions tend to produce a different gangue. Reduction of seawater sulphide produces the weak carbonic acid, which may be flushed in the shallow aqueous conditions, while the production of H2S and then pyrite results in a carbonate gangue of dolomite as detailed below. These reactions represent the first stages of sulphide which are overprinted by copper sulphides as Cu-rich solutions infiltrate in situ reductant bed, or enter the mobile reductant reservoir. Early pyrite is preserved either as cores to later sulphides, or distal to both ore and the influx of Cu-rich solutions, where they are not replaced.
Influx of an oxidised Cu-bearing brine, most likely with a chloride ligand, which is in equilibrium with footwall oxidised arenites, is reduced on contact with the reductant, decreasing the solubility of Cu, and resulting in its release from solution. This leads to the generation of chalcopyrite, either by addition of Cu to the lattice of existing pyrite, without additional sulphur: 2 FeS2 + 2 CuCl + H2O → 2 CuFeS2 + 2 HCl + 0.5 O2, or by direct combination with iron oxide and H2S in the mobile reductant reservoir: Fe2O3 + 4 H2S + 2 CuCl → 2 CuFeS2 + 2 HCl + 3 H2O. The addition of further Cu progressively converts chalcopyrite into bornite, either by complete replacement, or as rims to a chalcopyrite (or pyrite) core, as follows: 4 CuFeS2 + 6 CuCl + 3 H2O → 2 Cu5FeS4 + 6 HCl + Fe2O3. While Cu-bearing brine remains, or continues to ingress, the bornite is progressively converted to chalcocite: 2 Cu5FeS4 + 6 CuCl + 3 H2O → 8 Cu2S + 6 HCl + Fe2O2.
The zoning pattern and paragenesis suggests that after initial pyrite and early chalcopyrite deposition, the process of ore formation predominantly involved the addition of Cu to alter existing sulphides, with lesser new sulphur added to the assemblage.
Each new generation of Cu sulphide with a progressively higher Cu:S ratio, begins to be generated from the last, at the interface between the incoming oxidised Cu-bearing brine in the oxidised arenites and the sulphide body, whilst sulphides with the lowest Cu:S ratio are distal, grading outward into barren pyrite. The above zoning pattern suggest the fluid influx was both from below and laterally from the syn-sedimentary structures, i.e., the fault structures were the conduits for the mineralising fluids, which were released into porous footwall arenites, and precipitated when reducing hydrocarbons were encountered, either within those arenites or an overlying aquitard.
As indicated above (Reductants and redox boundaries section), the excess HCl produced by these processes may reverse or terminate the initial generation of H2S, unless buffered by excess Fe, Mg or Mn ions which produces a gangue of dolomite, magnesite and ferroan dolomite as excess Fe is released.
As the excess Fe, Mg or Mn ions are utilised and the system become more acidic, it is unlikely that new H2S can be produced. In this event, the only avenue for the takeup of Cu released from the reduced brines is by combining with existing sulphides converting them to species with progressively higher Cu:S ratios.
Borg et al. (2012) suggest that a similar process of sulphide formation and acid generation, promoted the dissolution of interstitial sulphate and carbonate cements in the Kupferschiefer of Europe, and increased permeability, allowing the progress of the mineralising front into cemented host rocks and ultimately the slow escape of depleted mineralised brines into the rocks above, permitting the influx of new brines from below.
Geochronology of Ore
An Re-Os isochron age for chalcopyrite in diagenetic evaporitic nodules in the hanging wall (not ore) of the Konkola deposit (Barra et al., 2004), yielded an age of 816±62 Ma presumed to be close to the depositional age, which is post 877±11 Ma (basement Nchanga Red Granite age).
The minimum age of 645±15 Ma determined for copper-sulphides in little deformed disseminated ores from the Musoshi deposit, DRC (Richards et al., 1988) would be consistent with a late diagenetic emplacement.
Most datings of sulphides and ore-related minerals in the Zambian Copperbelt give Lufilian ages of 670 to 550 Ma. Available data from Selley et al. (2005) and Zientek et al. (2014) includes the following:
• Nkana-Mindola, Chibuluma West and Nchanga - 576±41 Ma (7 point Re-Os, from disseminated & veinlet chalcopyrite and bornite in altered siltstone; Barra and Broughton, unpub.);
• Nkana, Chibuluma and Nchanga - 583±24 Ma (5 point isochron with analyses from the 3 deposits; Barra et al., 2004);
• Nkana-Mindola - 520±20 Ma (U-Pb uraninite; Cahen et al.,1971 and references therein); 522±15 Ma (U-Pb uraninite; Darnley et al., 1961);
525±3.4 Ma (Re-Os molybdenite from a chalcopyrite-bearing vein that cuts stratabound mineralisation; Barra et al., 2004);
• Musoshi - 514±2 Ma (U-Pb rutile in quartz-hematite-(microcline-biotite-rutile) veins with late calcite, siderite, anhydrite, and barite cutting stratabound Cu; Richards et al., 1988); 514±3 Ma (U-Pb uraninite from a late cross-cutting vein; Richards et al., 1988); 496±1 Ma (U-Pb rutile; Richards et al., 1988); 645±15 Ma (Pb-Pb Cu sulphide; Richards et al., 1988);
• Kansanshi - 503±15 Ma (U-Pb uraninite; Darnley et al., 1961); 512.4±1.2 Ma (Re-Os molybdenite; Torrealday et al., 2000); 502.4±1.2 Ma
(Re-Os molybdenite; Torrealday et al., 2000); 511±11Ma (U-Pb monazite; Torrealday et al., 2000).
The range of dates, styles of mineralisation, textural evidence and the presence of ore in rocks younger than this earliest age, suggest mineralisation took place over a protracted period from late diagenesis to post orogenesis (Hitzman et al., 2012), or record remobilisation of sulphide minerals during metamorphism and deposition (Zientek et al., 2014).
A postpeak metamorphism albitisation event associated with quartz-carbonate veining is in places accompanied by Cu-U-Mo-(Au) mineralisation, and occurred at ~512 to 500 Ma (Musoshi; Richards et al., 1988; Mindola and Kansanshi; Darnley et al., 1961; Torrealday et al., 2000).
Late stage mineralisation is represented by concordant Rb-Sr and Re-Os ages on sphalerite-bornite and bornite-renierite of ~451 Ma at Kipushi (Schneider et al., 2007), and 39Ar-40Ar ages of 490 Ma and ~463 Ma, respectively, for biotite and muscovite intergrown with sulphides at Samba (M. Hitzman, unpub. data, 2006, reported in Hitzman et al., 2012).
Basement Mineralisation
Basement mineralisation is found in numerous locations within the Lufubu Complex gneisses, schists and granitoids, both in close association with, and distal to, Roan Group hosted deposits. Mineralisation occurs in a number of forms, specifically:
• within palaeosols/palaeoregolith formed at the Katangan unconformity, where Roan Group hosted orebodies rest directly onto basement topographic highs. This is interpreted to represent mineralising fluids that have penetrated into the weathered regolith during the main ore formation. In some localities this mineralisation persists below the regolith, e.g., at Mufulira, where deep fissures filled with Roan Group sands, pebbles and boulders contain disseminated pyrite and chalcopyrite. Similar mineralised palaeosols have been outlined at Nkana and Nchanga (Pienaar, in Mendelsohn, 1961).
• mineralised veins within basement below orebodies, e.g., at Luanshya, where the main orebody in the lower Roan Subgroup rests directly on basement, chalcopyrite and minor pyrite occurs in sporadic, short, discontinuous quartz-calcite veins and as disseminations within schist (mainly biotite schist). The overall average grade in the zone is ~1% Cu, over a thickness of 10 to 15 m below the unconformity. Similar mineralisation has been outlined at Nchanga in both the palaeosol and as dissemination and veins in the underlying granite to depths of ~8 m, carrying an average of 0.77% Cu. At Mufulira, pyrite and chalcopyrite occur in short, erratic and discontinuous quartz-carbonate veins which contain some anhydrite. The more persistent veins strike NE and dip to the NW. At the Selkirk shaft (Mufulira), mineralised veins up to 2 cm thick occur in swarms up to 400 m below the unconformity, hosted by both schists and granites (more abundant near granite contacts), with maximum grades of up to 3% Cu over 2 m intervals (Pienaar, in Mendelsohn, 1961).
• basement host deposits removed from Roan Group ores, the most significant of which is the Samba deposit, hosted in 1.9 Ga (Rainaud et al., 2005) basement metavolcanic rocks on the western edge of the Zambian Copperbelt, 21 km south of Chingola and 24 km west of Chambishi. Mineralisation is hosted by quartz-sericite to quartz-biotite and chloritic schists, which have gradational contacts with sandwiching granodioritic to quartz-monzonitic gneisses (Wakefield, 1978; Freeman, 1988). It occurs as disseminations, stringers and veinlets of pyrite, chalcopyrite and bornite, with minor shallow malachite development. The mineralisation forms lenses that dip at 45 to 80°S and vary from a few mm to ~10 m in thickness, usually decreasing in width with depth. Grades vary from trace to 2.16% Cu over 8.15 m widths, the best being 1.68 m of 4.11% Cu. Two or three, locally up to eight, bands are present at any one interval, each separated by waste bands that are from 2 to 140 m thick. Samba has been interpreted to be a Palaeoproterozoic porphyry Cu deposit with a resource of ~50 Mt @ 0.5% Cu (Wakefield 1978). Freeman (1988) quotes a resource of 14 Mt @ 1.1% Cu with a higher cut-off, based on the tabular bands of ore. However, Hitzman et al. (2012), quotes unpublished data (M. Hitzman, unpub. data, 2006), which has demonstrated that mineralisation took place between 490 and 460 Ma, during late to post Lufilian orogenesis.
Another of the basement accumulations (undated) is the Fimpimpa Prospect, on the NE tip of the Kafue Anticline, 20 km NE of Nchanga and <10 km west of Lubembe, where copper mineralisation occurs along a massive quartz vein cutting basement garnetiferous quartz-mica schists and biotite gneisses, and in an adjacent, vermiculite-rich lamprophyre dyke (Freeman, 1988) or feldspathised quartz diorite (Pienaar, in Mendelsohn, 1961). The lamprophyre dyke is continuous for 20 km south to the Nchanga River Lode. The quartz vein is ~8 m thick with sporadic malachite, chalcopyrite and bornite and is largely < 0.4% Cu (Pienaar, in Mendelsohn, 1961). The lamprophyre dyke is host to fracture, quartz-calcite vein to veinlet hosted and disseminated malachite, chalcopyrite and bornite mineralisation over widths of up to 14 m. The known resource over a 1000 m interval tested was estimated at 1.2 Mt @ 1.23% Cu to a depth of 100 m, including 0.2 Mt @ 1.19% Cu as cupriferous mica to a depth of 15 m (Freeman, 1988).
The Mkushi copper deposits are hosted by late Palaeoproterozoic to Mesoproterozoic Irumide Belt basement rocks that extend from the Kafue Anticline of central Zambia into northern Malawi. Mkushi is located 123 km SSE of Ndola and ~100 km NE of Kabwe. It occurs as a series of shear controlled, curvilinear, left stepping en echelon lenses of disseminated chalcopyrite.
Most of the basement hosted occurrences below the palaeoweathering zone have been assumed to represent pre-Katangan mineralisation and illustrate the fertility of the basement as a source of metals. However, the dating of Samba questions this latter assumption. If other occurrences are shown to be Lufilian in age, they may instead reflect the passage of mineralised fluids upward into the Katangan sequence.
Central African Copperbelt - Lufilain Arc Architecture and Distribution of Mineralisation
The development of the Lufilian structural arc, its key elements and the distribution and variations in pre-, syn- and post folding mineralisation it encompasses, may be summarised as follows:
• Kundelungu Gulf Foreland - which is peripheral to the outer Lufilian Arc. Exposure is dominated by the late Kundelungu Group, particularly the syn- to post-orogenic, continental molassic siliciclastic rocks of the Plateau des Biano (Ku-3; Kampunzu and Cailteux,1999) overlying a condensed sequence of late Roan and Nguba groups rocks. Deformation in this foreland is interpreted to be by D1 and D2 faulting above a flat lying detachments separating the Katangan sequence from basement.
A number of small, but high grade deposits occur within the northern Kundelungu Gulf Foreland, emplaced during the Lufilian orogenic event only e.g., Dikulushi, a vein Cu-Zn-Pb-Ag deposit hosted by Lufilian faults cutting Lubudi (Ku-1.4) and Mongwe (Ku-2.1) Formation rocks of the Kundelungu Group hosts, where syn-orogenic, moderately saline (20 to 25 wt.% CaCl2 equiv.) and warm (90 to 140°C) fluid precipitated Cu-Pb-Zn-Fe ore in a zone of crosscutting east and north-east oriented faults (Van Wilderode et al., 2014).
• Outer External Fold and Thrust Belt - which has been subjected to the D1 Kolwezian event, characterised in this section of the arc by north to NE vergent folding, thrusting and nappe development, with many of the thrusts exploiting the base of the R.A.T. Subgroup/megabreccia package in the Congolese Copperbelt, where a residue of salt remained. These structures followed the interpreted preceding gravity gliding planes and closely post-dated deposition of the Kundelungu Group Mongwe Formation (Ku-2.1). D1 thrust faults propagated to the surface, and transported allochthonous, flat lying to shallowly dipping sheets of Roan, Nguba and basal Kundelungu group rocks to the north and NE, to structurally overlie para-autochthonous Ku-2.1 over an area of >1400 km2 in the outer Congolese Copperbelt, (François, 1973; 1991; 1994).
Substantial areas of Roan megabreccia (200 to >400 km2) are exposed in the main zone of thrust and nappe development in this section of the arc (e.g., Kolwezi Klippe, Tenke-Fungurume window), hosting many of the larger Cu-Co deposits or deposit swarms, which occur as stratabound bodies within megabreccia écailles. These deposits are largely composed of pre-folding diagenetic sulphides, but most also include a coarser post-folding sulphide overprint. Most have been deeply weathered and predominantly exploited as supergene oxide ores.
• Inner External Fold and Thrust Belt, to the south and SW, where Roan Group rocks hosting significant Cu-Co mineralisation, occur i). in smaller windows (<1 to <10 km2) of megabreccia in faulted D1 antiforms (e.g., as at Kambove, Kakanda, Kisanfu and Etoile, within Mines Subgroup écailles, and Mutanda, Deziwa, Tilwezembe, within Dipeta Subgroup rocks), and progressively to the south and SW, ii). as diapirs of similar dimensions, within the D2 Monwezian strike slip faults with uranium and lesser Cu-Co (e.g., Shinkolobwe Swambo, Menda and Kalongwe) in Mines Subgroup écailles, and iii). further to the south and SW again, an arcuate belt of Zn-Pb-Cu-Ag occurrences and deposits (e.g., Kengere, Lombe and Kipushi) in Mwashya Subgroup, and Nguba and Kundelungu group écailles within late diapiric megabreccia. See geological maps in the Congolese/Katangan Copperbelt record for locations and settings of these deposits.
Mineralisation within the Mines Subgroup hosted deposits are similar to those described in the outer External Fold and Thrust Belt, i.e., dominantly pre-folding diagenetic sulphides, but most also include a coarser post-folding sulphide overprint. Those deposits within the Dipeta Subgroup include fine sulphides developed along and across bedding planes, but also later fault controlled sulphides and appear to represent both pre-folding and syn- to post-deformational ores. The Zn-Pb-Cu-Ag occurrences and deposits predominantly represent late, Lufilian age mineralisation.
These diapiric windows and diapirs indicate appreciable thicknesses of salt had survived in the Roan Group in this region up to and into the Lufilian Orogeny.
• Straddling the inner External Fold and Thrust Belt and the Domes Region, further south again, folding predominates, and Roan Group diapirs become increasingly rare towards the Domes Region, where basement is exposed, as discussed below. Mineralisation predominantly occurs within Mwashya Subgroup and Nguba Group rocks (although some are found in exposed Roan Group rocks), mainly as numerous small occurrences of iron, copper, gold and uranium, all associated with regional sodic-calcic alteration. The largest of these is the vein swarm Kansanshi Cu-Au deposit, hosted within metamorphosed rocks interpreted to belong to the Mwashya Subgroup and Grand Conglomérat of the Nguba Group, on the northern margin of the Domes Region. Mineralisation at Kansanshi appears to be exclusively post-folding and was introduced in two pulses dated at ~512 and 502 Ma.
Many of the iron occurences in this part of the arc comprise magnetite and hematite occurring as cements to hydrothermal breccias and as fracture fillings, with or without sulphides (Lobo-Guerrero, 2010). Others are the stratabound magnetite-hematite lenses and beds interbedded with the mafic volcanic rocks of the Dipeta Subgroup Kansuki Formation (previously the Lower Mwashya) and equivalent uppermost Upper Roan Subgroup (e.g., Kasumbalesa Fe on the DRC-Zambia border, 80 km SE of Kipushi), which are also found in the further to the north and NE in the main Cu-Co zone of the inner External Fold and Thrust Belt. In addition, metagabbro sills within the Domes Region and margins of the Kafue Anticline, are known to contain magnetite rich layers up to ~8 m thick.
• Domes Region, which forms a broad arcuate trend, extending east to SE from the Angolan border, to obliquely intersect and overprint the Kafue Anticline in the east. It involves significant basement in the deformation pattern in the form of the domal cores, which act as buttresses during Lufilian deformation. NE vergent folding and thrusting predominantly occurs above broad mylonite and white schist zones, which follow the detachments, separating the basement domal cores from the overlying Katanga Supergroup rocks. Like the Kafue Anticline, the domes are interpreted to be underlain by mid- to lower crustal ramps (Daly et al., 1984), developed during compression. Isoclinal folds were formed on the NE margins of the domal buttresses. Roan Group rocks are prominent again and have been locally interleaved with basement by differential displacement along the multiple thrusts that consitute the detachments. Significant Cu, Cu-Co, Ni-Cu-Co and Cu-Au deposits are hosted by carbonaceous beds in close association with the mylonitic detachments of the domes (e.g., Sentinel Cu-Co and Enterprise Ni-Cu in the hanging wall, and Malundwe and Chimiwungo Cu-Co-U at Lumwana in the footwall). These deposits may have been emplaced during either the renewed extension, or Lufilian compressive events, or both. The Kansanshi Cu-Au deposit, described above, is located 12 km north of, and well into the hangingwall of the Solwezi Dome, in a zone of complex isoclinal folding, shearing and faulting.
• Zambian Copperbelt, is characterised by two NW-SE trending parallel lines of Cu mineralisation some 20 km apart, straddling the Palaeoproterozoic basement gneisses, granitoids and schists, and Mesoproterozoic conglomerates, quartzites and granitoids of the NNW-SSE trending Kafue Anticline. Unlike the outer sections of the Lufilian Arc, basement played an important role in the structural architecture of the Zambian Copperbelt. Daly et al. (1984) interpret the structural pattern to reflect ENE vergent thrusting above a deep décollement within the basement of the Kafue Anticline, with associated branching subsidiary thrust ramps that climbed up through the sequence. In places the thrusting passes up into the Lower Roan Subgroup to separate competent basal beds immediately above basement from overlying shale units (often the Copperbelt Orebody Member). Otherwise, basement faulting is predominantly expressed as folding within the overlying Roan Group rocks. Mineralisation is predominantly hosted by the Lower Roan Subgroup, composed principally of coarse siliciclastics (conglomerate to arkose and siltstone, with lesser carbonate rocks). In the major deposits (Luanshya-Baluba, Nkana-Mindola, Mufulira, Chambishi, Nchanga-Chingola, Konkola-Kirilabombwe-Lubambe and Musoshi), sulphides are mostly diagenetic, pre-folding disseminations and veinlets, with a significant, overprinting, coarser, post-folding generation. In the southern part of the eastern line of mineralisation, the first reduced unit above basement is the Mwashya Subgroup carbonaceous shales. These host the Frontier and Lonshi deposits both of which are structurally controlled and post-folding.
• Onlap sequences overlying Kibaran and Irumide basement adjacent to the main rift basin, deposited during the renewed extension period. These generally comprise Nguba Group rocks, overlying basement direct, or separated by thin Roan Group slivers.
To the NW in the DRC, the Kamoa Cu-Ag deposit is hosted by the Grand Conglomérat of the Nguba Group, in which carbonaceous diamictites and pyritic siltstones to fine sandstones, also includes interbedded mafic volcanic rocks. This sequence is separated from Kibaran basement by a thin (several tens to several hundreds of metres thick) wedge of oxidised coarse, permeable clastic rocks of the Mwashya Subgroup. The mineralisation appears to comprise diagenetic early pyrite, overprinted by high temperature (~225°C) hydrothermal copper sulphides with potassic, silica and magnesian alteration, but only minor local veining which has albitic-dolomitic selvages. Copper sulphides are both fine-grained replacing diagenetic pyrite and coarse grained clusters overprinting finer grains, to semi-massive accumulations, suggesting two phases of ore formation.
To the SE in Zambia, the Fishtie copper deposit, is hosted by the flat lying Grand Conglomérat and Kakontwe Limestones, directly overlying largely impervious quartz-muscovite schists and quartzites of the Irumide basement, without an underlying permeable bed. Mineralisation comprises early diagenetic pyrite, followed by a stratabound replacive phase of alternating bands of magnetite, magnetite-quartz, magnetite-ankerite, ankerite or apatite, hosted within the Grand Conglomérat, decreasing in intensity away from a series of steep faults. Later chalcopyrite and lesser bornite copper mineralisation overprints the iron oxides, within weakly carbonaceous Grand Conglomérat and in highly carbonaceous dolomitic siltstone of the lower Kakontwe Limestones. The Cu sulphides are focused along normal faults, over vertical intervals of up to 50 m, but with ore grades laterally restricted to within 50 m of the inferred fault plane, zoned outward from bornite to chalcopyrite to pyrite. The age gap between the iron oxides and Cu-sulphides is uncertain.
• The Synclinorial zone lacks structural complexity and significant mineral occurrences.
• The Katanga Core is characterised by felsic intrusions, comprising the syn- to post-tectonic, composite, Hook Granite batholith dated at ~560 Ma and related, younger megacrystic phases, and extensive hypabyssal granitoid to rhyolite ring complexes and syenites that were intruded into the Roan and Nguba Group sequences between ~550 and 530 Ma, along with lesser, generally coeval, gabbroic rocks.
Mineralisation within the Katanga Core is largely associated with the regional sodic-calcic alteration that overprints the early calcic and potassic stages detailed previously. It is characterised by albite and scapolite that may extend over areas of >100 km2 (Lobo-Guerrero, 2010). Associated mineralisation includes widely distributed occurrences of magnetite/hematite, copper and precious metals, including some significant deposits (e.g., Mumbwa/Kitumba, Kasempa and Kalengwa) as detailed in the Regional IOCG-style paragraph of the Alteration section above. This alteration and style of mineralisation is very similar to occurrences seen in the inner External Fold and Thrust Belt, Domes Region and Zambezi Belt. Mineralisation appears to have occurred during both the renewed extension at ~735 Ma when magnetite deposits were formed, and during the period of late to post-tectonic relaxation. It is uncertain if significant Cu accompanied the first phase. The main Cu would appear to have been emplaced during the latter, after 530 Ma (e.g., as is suggested at Mumbwa), through to 500 Ma (e.g., at Kansanshi in the Domes Region). The Cu sulphide mineralisation overprints the magnetite phase at Mumbwa and Kalengwa, while no iron oxides, only pyrite and pyrrhotite, are present at Kansanshi, and only minor Cu-sulphides accompany the magnetite at Kasempa.
Timing, Origin and Discussion
Based on the observations and interpretations outlined and cited throughout this summary, and that of the Congolese/Katangan Copperbelt record, the following sequence of events and processes are proposed to explain the development of the Lufilian Arc and the ore deposits of the broader Central African Copperbelt in both Zambia and the DRC:
• Following basin inversion of the NW-SE trending Kibaran and Irumide rift systems, and amalgamation of the Rodinia Supercontinent by ~1.05 Ga, there was an ~100 to 200 m.y. lull in tectonic activity in what is now southern and central Africa, although deposition continued in the Damaran region to the SW.
• Early extensional magmatism - After ~900 Ma, extension commenced in what was to become the Damara-Katanga belt, part of a world wide event that heralded the progressive break-up of Rodinia. This break-up continued over the next 200 m.y., to around 700 Ma. Extension in the Damara-Katanga belt produced intra-continental rift basins, while on a global scale, by the end of this interval, Rodinia was broken into five new continents, the larger West Gondwana (which includes the Damara-Katanga belt) and the smaller East Gondwana (Australia, Antarctica and India), Laurentia, Siberia and Baltica.
On a continental scale, the morphology of the NE-SW elongated composite Damara-Katanga belt basins was influenced by sutures resulting from the Palaeoproterozoic collision between the predecessors of the Kalahari, Angolan/Congo and Tanzanian(-Bangweulu Block) cratons. Extension appears to have been oblique to the main trend of the belt, accommodated by translation along the sutures marked by the Mwembeshi Shear Zone in the NE and Matchless belt to the SW.
On a regional scale, NE-SW directed extension began in the area of the Central African Copperbelt, immediately to the SW of the main Kibaran-Irumide depocentres. This extension resulted in early, ~880 to 850 Ma bimodal volcanic activity and granitic intrusion in what is now the Zambezi Belt and possibly the southern Lufilian Arc, while in the main Zambian Copperbelt, no volcanic rocks are evident, and only limited granite intrusion is preserved (e.g., the ~880 Ma Nchanga Red Granite). This latter intrusive activity accompanied the uplift of a NW-SE elongated horst block or upwarp, that was to become the Kafue Anticline and form the core of the Zambian Copperbelt. Following intrusion, the horst/upwarp was eroded to expose the granites, prior to deposition of the basal Katanga Supergroup on its flanks.
This early bimodal magmatism is characteristic of intra-continental rifting, taken to be the result of crustal thinning due to extensional attenuation, shallowing of the Moho, concomitant mantle upwelling, pooling and development of a thick, hot, mafic underplate, with consequent anatexis of the lower crust. The mafic volcanism comprises mantle derived magma that has been differentiated and contaminated by crust to achieve sufficient buoyancy to rise above the Moho, while the felsic volcanism and intrusions are anatectic crustal melt material.
• Main extension - Extension continued with the development of a number of NW-SE trending extensional fault controlled rift basins (grabens or half grabens), flanking the "Kafue Anticline" basement high, restricted to the Zambian Copperbelt area. These basins were normal to the overall trend of the Damara-Katanga belt, and were filled with diagnetically reddened coarse, rift facies clastic sediments (Mindola Clastics Formation) of limited lateral extent. With progressive extension and subsidence, these became interconnected along fault structures and grew into a larger rift basin, predominantly in Zambia. There is no convincing evidence of similar and coeval rocks in the Congolese Copperbelt.
The diagenetic reddening of rift facies clastic sediments within an intracontinental basin involves the oxidation of common iron-bearing minerals (ferruginous silicates and iron oxides) by oxidised pore fluids (most likely oxidised meteoric water) to release iron and produce iron hydroxides and oxides. This process may progressively take place over millions of years. Conditions in these basins are unlikely to have been truly reducing or significantly acid, but more likely, close to the Fe2+/Fe(OH)3 or Fe2+/hematite transition, due to the pH-buffering effect of the reddening process itself, i.e., oxidants would be consumed by the production of iron hydroxides (e.g. goethite) and hematite, and any significant acidity would be neutralized by reactions such as plagioclase and biotite alteration to smectite (Brown 2005 and references cited therein). Brown (2005) indicates that this diagnetic reddening simultaneously releases trace amounts of copper, first to be adsorbed onto early goethite, then to be re-released to pore brine as goethite crystallises to form the hematitic pigment typical of red beds.
• Deposition continued with the laterally more extensive Kitwe Formation, composed of clastic rocks, passing upward into dolomitic sediments within the main rift basin in Zambia. The upper dolomitic beds mark the waning of rifting and the onset of a thermal sag phase.
A 10 to 15 km wide corridor of argillaceous rocks of the Copperbelt Orebody Member occur at the base of the Kitwe Formation, fringing and lapping onto the western margin of the "Kafue Anticline" basement, capping the Mindola Clastics Formation, but lensing out into the basin to the west.
The Copperbelt Orebody Member represents a transgressive event. It contains ample in situ hydrocarbons and early diagenetic, generally framboidal, pyrite disseminations and nodules, formed by bacterial sulphate reduction from sea water soon after deposition. See the Zonation of Ore Minerals section for the chemical reactions.
• Thermal sag phase - The succeeding thermal sag phase is represented by the Upper Roan Subgroup, dominantly composed of carbonate rocks, including the first interpreted evaporite unit, now represented by a breccia zone after total dissolution. These sag phase rocks transgressed onto basement, well beyond the margins of the main rift basin, particularly to the north and NE into the DRC, where salt/evaporite, carbonate and reefal units were developed in a lagoonal to shallow marine environment, forming the R.A.T., Mines and Dipeta subgroups of the DRC Roan Group.
Evaporitic sequences of the type envisaged, are usually composed of cyclic repetitions from carbonatic mudflats → limestone/dolomite → anhydrite/gypsum → halite/sylvite (e.g., the Permian Zechstein in Europe, which includes the Kupferschiefer Cu deposits at its base). The mudflat to carbonate segments represent transgressive conditions and the anhydrite to halite, regression. The salt phase may be followed by a further anhydrite/gypsum band as the regression turns to the next transgression. Sequences of this type up to 2 km thick can be deposited in 0.3 m.y. and occur in shallow basins isolated from, or with only restricted access to the open ocean. In these restricted basins, in tropical climates, evaporation results in supersaturation and precipitation of salts to the basin floor, with only limited recharge to maintain the water level, avoid dilution and flushing, but supply new sea water to seep in and replace that lost by evaporation (e.g., Börner, 2004).
A stromatolitic reef appears to have formed in the Congolese Copperbelt area, possibly in part on a shallow rise marking the northward continuation of the "Kafue Anticline" basement high. Evidence of the reef is seen in the Mines Subgroup (particularly in the R.S.C. Member), where facies changes are evident from SW to NE across strike, from massive stromatolitic reef, to coarse reef debris, to finer reefal bioclastics, to the absence of a reef facies. This reef restricted sea water flow between the main marine basin to the SW, and the shallow embayment to its north and NE, between the Congo Craton and Bangweulu Block, and led to the concentration of hypersaline brines and the accumulation of a thick (>500 m) salt/evaporite bed in a lagoonal setting with landward sabkha margins. The R.A.T. Subgroup, is interpreted to be the clastic residue after the post burial to orogenic dissolution of most of these salt/evaporite beds. It comprises clastic matrix to the evaporites, and interbeds of mudstone, siltstone to fine sand. The lagoonal to marine shelf Upper Roan Subgroup in Zambia was not restricted, although the basin may have been partially isolated from the ocean, and consequently, the salt/evaporite unit was thinner.
Following the initial shallow, oxidised R.A.T. Subgroup evaporitic sequence, the Mines Subgroup in the Congolese Copperbelt marked a transgressive event, and deepening of the basin over and north of the reef. It is largely composed of reduced carbonate-mudflat rocks that, like the Copperbelt Orebody Member in Zambia, contain ample in situ hydrocarbons and early diagenetic, generally very fine grained, pyrite disseminations and nodules, formed by bacterial sulphate reduction from sea water. The 10 to 30 m thick R.S.C. Member, originally largely composed of stromatolitic dolostones, occurs about 10 m above the base of the Mines Subgroup, overlain and underlain by laminated dolomitic shales that were deposited within a shallow-water, high-salinity, reducing, intertidal environment. These two laminated members were to become hosts to the main Lower and Upper orebodies, separated by barren, highly silica-dolomite altered R.S.C. in most of the Congolese Copperbelt orebodies. Similar reduced beds occur higher in the Mines Subgroup forming a third orebody horizon, marking a minor transgression.
The Mines Subgroup is overlain by the Dipeta Subgroup, with the basal R.G.S. Member marking a regression and change to oxidised clastic and evaporitic conditions, partially analogous to the R.A.T. Subgroup, before transgression and a return to reduced carbonatic and reduced rocks more similar to those of the Mines Subgroup.
• Regional calcic-magnesian alteration - At some time following the deposition of the salt/evaporite unit within the Upper Roan Subgroup in Zambia, the Roan Group was subjected to regional calcic-magnesian alteration, in the form of diagenetic anhydrite, dolomite, calcite and quartz, mainly occurring as interstitial disseminations, replacements, veinlets and overgrowths throughout the Roan Group. This alteration is inferred to be related to the dissolution and redistribution of the evaporite bed(s) by diagenetic brine reflux, a process whereby dense Mg-Ca-SO4 brines, dissolved from salt/evaporite beds, sink to displace lighter fresh pore waters in underlying units to precipitate the alteration minerals. It resulted in up to 30% authigenic anhydrite in large sections of the underlying, Lower Roan Subgroup in Zambia, particularly within non-evaporitic arenite beds of the Mindola Clastics and Kitwe formations. Moderately saline Na-Ca-Mg brines, with trapping temperatures of 86 to 129°C, that were detected in early basinal fluid inclusions (Greyling, 2009) may represent the fluids responsible for this alteration. The presence of NaCl in a solution (which is soluble at relatively low temperatures), increases the solubility of calcite, dolomite and magnesite, and to a lesser extent gypsum.
Early diagenetic celestite, gypsum and anhydrite nodules are similarly recorded (where described) in the Congolese Copperbelt deposits, while carbonate (mainly magnesite) crystal growth occurs in carbonate and argillaceous-carbonate rocks, and Mg-chlorite in more argillaceous rocks. This alteration is attributed to dissolution, circulation and redistribution of evaporite/salt beds in the the R.A.T. and R.G.S., into other parts of the Mines and Dipeta subgroups. This stage of altersation is less obvious in the evaporitic Roan Group sequences int the Congolese Copeprbelt, in contrast to the evaporite poor Lower Roan Subgroup in Zambia.
• Regional potassic alteration - Mg-K rich basinal fluid inclusions with trapping temperatures of 132 to 160°C (Greyling, 2009), may represent brines that were dissolved from evaporite beds in the Roan Group, and then circulated within the basin, overlapping with and succeeding the cooler Na-Ca-Mg brines. These brines were probably responsible for the regional pre-ore potassic alteration. Sylvite (KCl), and to a lesser extent Bischofite (MgCl2) becomes progressively more soluble with increasing temperatures, as the sequence thickened and burial depths increased.
In the carbonate dominated Mines Subgroup in the Congolese Copperbelt, an equivalent early regional potassic alteration is also obvious. However, before the deposition of ore, the subsequent dominant district to deposit scale alteration is characterised by pulses of carbonate and/or silica, generally i). an early carbonate (mainly magnesite) crystal growth in carbonate and argillaceous carbonate rocks, and Mg-chlorite in more argillaceous rocks, ii). followed by silicification, commonly as microcrystalline quartz, and iii). prismatic inclusion-rich quartz±carbonate (generally dolomite), and iv). quartz±dolomite accompanying fine grained stratabound Cu-Co ore.
• Renewed extensional event - The Upper Roan and Dipeta Subgroups, in Zambia and the DRC respectively, are conformably overlain by the deeper water, predominantly shallow marine black pyritic shales of the Mwashya (or Mwashia) Subgroup. Mafic magmatism, which commenced during deposition of the upper Dipeta Subgroup in the DRC, and intruded Roan Group rocks in the Domes Region, heralded the beginning of a major renewed phase of extension, which resulted in:
i). deepening of the Katanga basin and associated transgression;
ii). northward migration of the main centre of deposition into the DRC, where the environment had previously been lagoonal to marine shelf;
iii). expansion of the sequence onto Kibaran and Irumide basement on the basin margins to the NW and SE respectively, and into the extensive Kundelungu Gulf Foreland to the NE;
iv). development of an elongate, arcuate, metamorphic core complex (or string of complexes), the undulose crest of which is exposed as the individual domes, defining the Domes Region in Zambia, updoming the Roan Group, and acting as basement highs during Nguba and Kundelungu groups deposition (see the Tectonic, Tectono-stratigraphic and Structural Setting, Domes Region paragraphs above). The complex (or complexes) brought deep, hot, devolatolising metamorphic rocks to shallow depths below a regional detachment, from which listric growth faults branched into the overlying Katanga Supergroup sequence;
v). a broad corridor of 753 to 742 Ma gabbroic intrusions coincident with the Domes Region, from the Kafue Anticline to the Angola border. These intrusions cut rocks from the Palaeoproterozoic Lufubu Metamorphic Complex in the dome cores to the lower Nguba Group on their flanks, but were preferentially emplaced as sills in the Upper Roan Subgroup. Granitic to syenitic intrusions, dated at ~735 Ma, are found in the same zone, predominantly to the west. Similar age gabbro and granite-syenite intrusions are also found in the Katanga Core (e.g., in the Kalengwa-Kasempa district);
vi). an extensive regional 'volcanic belt' was developed, preserved further to the north, defined by thin (<50 m thick) mafic and lesser felsic sills, lavas and associated pyroclastic flows and volcaniclastic rocks, interbedded with dolostones, shales and diamictites, from the Kansuki Formation (in the upper Dipeta subgroup), to the Grand Conglomérat of the Nguba Group. This curved belt extends from NW Zambia, following the Congolese Copperbelt to the north, east and SE to as far south as the DRC-Zambian border, just north of Konkola. It may have been transported northwards from closer to the broadly coeval Domes Region gabbroic intrusions during gravity gliding and over detachments during Lufilian folding. Over the period 765 to 735 Ma, this extrusive mafic magmatism progressed from continental tholeiite in the Dipeta/Mwashya subgroups, to alkaline and tholeiitic magmas, and finally to lavas with E-MORB affinities in the Nguba Group Grand Conglomérat Formation diamictite sequence (i.e., a transition from crust-contaminated to less modified asthenospheric melt).
This period of extension resulted in additional crustal attenuation, further shallowing of the Moho, large scale mafic pooling in the lower crust, the release of considerable energy, and a consequent increase in the thermal gradient. Although mafic magmatism was widespread, felsic equivalents are subordinate. This may be because the less readily melted mafic underplate from the first extension insulated the felsic crust from appreciable anatectic melting by the upwelling asthenosphere, but still allowed differentiation and ingestion of crustal material to produce sufficiently buoyant mafic magma that could rise and form the mafic intrusive and volcanic rocks observed.
• Following the Grand Conglomérat, deposition continued with the remainder of the Nguba Group in a deepening basin, starting with the Kakontwe Limestones, which are best developed in the Domes Region in Zambia to late siliciclastic siltstone and mudstones. The Kundelungu Group conformably follows the Nguba Group, commencing deposition towards the end of the period of extension with the Petit Conglomérat Formation, correlated with the global 635 Ma Marinoan glaciogenic event. The Kundelungu Group continued deposition into the main Lufilian Orogeny, where the uppermost unit, the flat lying syn- to post-orogenic siliciclastic rocks of the Plateau des Biano (Ku-3), were deposited in the Kundelungu Gulf Foreland at ~550 Ma (from the coincidence of the palaeomagnetic poles of the different blocks composing central Gondwana; Tohver et al., 2006). Ku-3 is interpreted as a continental molasse (Kampunzu and Cailteux,1999) related to the Lufilian orogenic event.
• Hydrocarbons - At some stage following deposition of the Roan Group, most likely during Nguba Group sedimentation, mobile hydrocarbons were generated and migrated up-dip or up-structure (faults), into permeable and porous stratigraphic or structural traps below a number of aquitards within the Roan Group, including i). argillaceous interbeds within the Mindola Clastics Formation; ii). the Copperbelt Orebody Member; iii). argillaceous beds within the Mufulira ore-bearing sandstone; iv). argillaceous beds higher in the Kitwe Formation; and v). the Mwashya Subgroup shales. In some cases, the mobile hydrocarbons infiltrated oxidised aquitards, locally converting them into reduced, carbonaceous siltstones/argillites, or augmented in situ hydrocarbons already within capping reduced argillites.
The stratabound copper sulphide deposits of the Roan Group in Zambia are almost invariably located within, or immediately above, concordant, stratigraphic or structural features that could trap migrating fluids, and which in most cases, contain (or can be inferred to have contained) a mobile hydrocarbon. These trap sites are dominantly, but not universally, where the fine grained cap rocks/aquitards listed above, overstep thinning wedges of underlying oxidised arenite, to lap, up-dip, onto basement, usually coinciding with syndepositional growth faults. Ore occurs either immediately below the cap rock, or within it, or both.
As outlined in the Reductants and Redox Boundaries section above, at a normal thermal gradient, mobile hydrocarbons are produced by the maturation of organic-rich sedimentary rocks, when they pass through the oil window at depths of 2 to 4 km and the gas window from 3 to 6 km, representing temperature ranges of ~60 to 120°C and ~100 to >200°C respectively, in the absence of other heat sources.
Potential source rocks containing sufficient organic matter include the Copperbelt Orebody Member, the Mwashya shale, other reduced facies that may be buried in the core of the Katanga Basin, and carbonaceous argillites at the top of the Irumide and Kibaran sequences. Most of these units would need to have been structurally displaced to a lower level during the renewed rift extension so that the hydrocarbons generated could migrate upwards to trap sites that are mostly in the Lower Roan Subgroup.
Except for the Kibaran-Irumide rocks, a sufficient burden of overlying rocks alone would not have been reached until deposition of the Kundelungu Group, immediately prior to basin inversion with the onset of the Lufilian Orogeny deformation. However, the shallowing of the Moho and the injection of mafic magmatism during both the initial and renewed extension would have raised the thermal gradient, particularly the sill like gabbro bodies preferentially emplaced in the Upper Roan Subgroup, between the potential kerogen rich source rocks of the Copperbelt Orebody Member and the Mwashya shales. This additional energy input would have decreased the depth of burial required to achieve the oil and gas windows temperature ranges.
By the time the source rocks within the core of the basin (not necessarily those at the site of the potential ore zones on the shallower basin margin) had been buried to the necessary depths, lithification would have been well advanced, and the hydrocarbons were generated and emplaced during late diagenesis. The reservoir rocks would have not only interstitial porosity, but also brittle fractures. The hydrocarbons would have to be emplaced before the permeability of the reservoir rocks was occluded by cements and advanced lithification. Although some authors suggest loss of permeability and porosity in a few million years (e.g., Hitzman et al., 2012), the prolonged preservation of permeability in this type of sedimentary sequence/setting over >100 m.y. is common, e.g., the Permian sandstones that host and form the footwall to the Kupferschiefer ores in Europe, also host petroleum reservoirs in Poland and the major North Sea oil fields, and are still both permeable and porous, allowing hydrocarbons to be extracted, almost 300 m.y. after deposition. In addition, formation of mobile hydrocarbons is known to occur within lithologies of this age elsewhere, as evidenced by petroleum bleeds into deep oil wells cutting the temporally, lithologically and tectonically comparable succession in the Amadeus Basin of Central Australia (e.g., Marshall et al., 2007). Another example is the 1.7 million km2, Triassic to Cretaceous Great Artesian intra-continental basin in Australia that supplies high volumes of artesian to sub-artesian brackish and saline waters through lithified Triassic (>200 m.y. old) aquifers from depths of up to 3000 m in central Australia, >1000 km from the recharge areas in the ranges of the tropical north, and also hosts commercial gas and oil fields. Waters in the southern extremities are up to 2 m.y. older than those in the recharge areas, permeating at ~1 to 5 m/year, related to the rate of discharge though seeps, springs and bores.
Alternatively, Selley et al. (2005) explore the possibility that the restriction of arenite-hosted deposits to within a 200 m thick fringe straddling the in situ hydrocarbon bearing Copperbelt Orebody Member, suggest that unit may have been a major source of mobile hydrocarbons that were released along the lower and upper boundary of the unit, and directed laterally through permeable media or vertically along fracture zones toward physical traps without substantial basinal migration. However, to do so, the ore-bearing section of the basin would have to go through the oil and/or gas window. This may well be the case, as fluid inclusion homogenisation temperatures of 110 to 170°C are quoted for diagenetic early chalcopyrite-bearing mineralisation replacing carbonate nodules at Chambishi by Annels (1989), and minimum fluid inclusion trapping temperatures of 120°C have been measured for fluids circulating at the time of diagenetic ore formation at a number of Zambian Copperbelt deposits (Greyling, 2009).
• Early pyrite - Following emplacement of mobile hydrocarbons into Roan Group reservoirs, it reacted with the up to 30% authigenic anhydrite precipitated during the earlier diagenetic calcic-magnesian alteration to produce H2S, which in turn reduced hematite within the oxidised arenites of the reservoir to produce pyrite in a dolomitic gangue, consuming the hydrocarbon in the process. Sulphate reduction continued until either all of the sulphate or hydrocarbon was exhausted, or until all of the Mg and Mn ions necessary to inhibit reversal of the sulphide reduction had been utilised (see the Reductants and Redox Boundaries and Zonation of sulphides sections above for the chemical reactions and constraints).
This late diagenetic pre-ore 'early pyrite' preceded Cu mineralisation, and was emplaced after lithification had commenced, but before loss of porosity and permeability. It is interstitial to arenites and sandy siltstones, but may form thin veins and veinlets in adjacent siltstones and shales that it infiltrates.
This 'early pyrite' associated with the influx of mobile hydrocarbons is younger than the early diagenetic 'early pyrite' of the argillaceous hosts produced soon after deposition of each of the main units containing in situ hydrocarbons, specifically, the Copperbelt Orebody Member (Lower Roan Subgroup), the Mwashya Subgroup and Grand Conglomérat (Nguba Group). As such, formation of 'early pyrite' took place on a number of different occasions in different parts of the basin and stratigraphic levels.
The 'early pyrite' associated with in situ hydrocarbons precedes and spatially overlaps ore zones, but is often much more laterally extensive, and may occur without any associated ore. It is also more likely to be disseminated in finer sedimentary rocks, rather than being confined to arenites and sandy silts. However, mobile hydrocarbons and associated pyrite are often less extensive because of the dependence upon trap sites.
Weak Cu-Co mineralisation may also have formed soon after early diagenetic pyrite, sourced from saline and K-Mg brines circulating and scavenging metal from the basin, particularly the Cu released into pore fluids during diagenetic reddening of the rift stage rocks (as described above, after Brown, 2005), and reacting with 'early pyrite', or becoming involved in the 'early pyrite' production reaction (e.g., the 816±62 Ma chalcopyrite in diagenetic evaporitic nodules at Konkola; Barra et al., 2004). This may have produced the kind of low-grade, but usually sub-economic mineralisation widespread in many rift basins.
The role of mobile hydrocarbons within the Mines Subgroup in the Congolese Copperbelt is uncertain, as most hydrocarbons there appear to be in situ. However, the presence of chalcopyrite and bornite cores to some framboidal 'early pyrite' in these deposits may indicate that more than one generation of 'early pyrite' and diagenetic copper mineralisation occurred.
• Sulphide generations - Petrographic observations and isotope studies indicate three broad generations of sulphide deposition within the Central African Copperbelt, both within Zambia and the DRC. Each is made up of multiple pulses that vary in timing and intensity across the Copperbelt. These are: i). widespread, early to late diagenetic pyrite - the 'early' pyrite described immediately above; ii). pre-folding, generally fine-grained, disseminations and veins containing copper and cobalt sulphides and pyrite, that usually replace 'early' pyrite, and have isotopic signatures suggesting low temperature bacterial sulphate reduction, most likely deposited during late diagenesis; iii). syn- to post-folding, coarser veins and lesser disseminations of copper sulphides, that formed during the main Lufilian Orogeny and late orogenic extensional collapse, to be described later.
• Pre-folding copper sulphide generation - These sulphides were the result of the ingress of oxidised, sulphur deficient, Cu-Co rich saline to hypersaline brines, probably at temperatures of >100°C, with Cu bound to a chloride ligand. When these oxidised brines, which were in equilibrium with oxidised footwall rocks, are reduced on contact with mobile or in situ hydrocarbons (thus decreasing the solubility of Cu), they are released from solution and may combine with an available sulphur source to generate copper sulphides. The available sulphur source in this instance is either H2S associated with mobile hydrocarbons, or 'early' pyrite. See the Zonation of Ore Minerals section for the chemical reactions.
The following constraints and relationships influenced the timing and distribution of this generation of mineralisation, namely:
- U/Pb isotope data for lead within the ore are consistent with derivation from basement rocks or from sedimentary rocks eroded from such basement (see the Source of Metals section above). However, much of the lead and uranium within these deposits is a late, post-copper addition, and may be related to a later evolution of the basin, and fluid generation that scavenged metal from another source, and as such, not reflect the source of copper and cobalt. The Central African Copperbelt is the world's largest cobalt resource, while some deposits have anomalous to economic nickel (e.g., Enterprise). Consequently, it would be unusual for there to be no contribution to the metal budget from the mafic intrusions of the region that appear to have a close spatial association with the ore deposits (see the Mafic Magmatism section above). It is likely that the mafic magmatism i). was a heat source that contributed to driving fluid convection; and ii). contributed fluids containing Cu-Co-Ni that mixed with the hot saline MgCl2-KCl basinal brines that were circulating and scavenging metals from the Roan Group and basement;
- The ~1850 Mt of copper estimated to be contained within the economic deposits, low grade halos and regionally distributed anomalous accumulations of the Central African Copperbelt (in both the second and third sulphide deposition stages) would require scavenging of an ~100 000 km3 of rock, which exceeds the volume of Lower Roan Subgroup rocks within the Katanga Basin - see the Source of Metals section above. Intense regional potassic alteration, as K feldspar and sericite-muscovite, predominantly affected the Lower Roan Subgroup, but also the Upper Roan, and Mwashia subgroups. This alteration phase is regionally distributed throughout the basin, both laterally and vertically, and as such is much more extensive than the Cu-mineralisation, although is also intergrown with Cu-Co sulphides within the orebodies. This implies significant fluid circulation, and reaction, within these sequences, which may have been related to metal scavenging. This is consistent with the metal rich MgCl2-KCl brines in fluid inclusions Greyling (2009) found associated with pre-folding ores in the Zambian Copperbelt. If so, at least some of the metal may have been extracted from the Roan Group rocks, which were originally derived from the basement, although the balance was probably derived from the basement (Selley et al., 2005).
- Zoning of sulphides strongly suggests that for the major deposits, Cu-mineralising brines were introduced from below via syn-sedimentary growth faults and released into either a porous and permeable unit, a clastic sedimentary wedge (usually an arenite in Zambia) or a concordant structure (e.g., décollements within the Copperbelt Orebody Member) that underlies a reduced aquitard, or a capped reservoir containing mobile hydrocarbons (see the Zonation of Ore Minerals section above). In the Congolese Copperbelt, the porous and permeable unit in the Mines Subgroup is the barren reef debris (i.e., more porous) facies of the R.S.C., Member, fringed above and below by mineralised carbonaceous dolomitic shale units, with a zoning suggesting outward permeation of Cu-mineralising brines from the R.S.C. into the hanging- and footwall (see the Congolese/Katangan Copperbelt record for detail);
- The Cu-mineralising brines are responsible for stratabound primary Cu-Co sulphide deposits that occur at a range of stratigraphic intervals from the 'Footwall deposits' of the Mindola Clastics Formation, to the Grand Conglomérat of the Nguba Group (see the Stratigraphic Control section above). However, ore is almost invariably found in association with the first reduced section and aquitard above basement. In Zambia this is commonly the package straddling the Copperbelt Orebody Member. If this position is mineralised, all overlying reduced beds are usually barren. Conversely, if this unit is absent, the next available above will host mineralisation.
These relationships suggest that the mineralising fluid was introduced from below, through a single, or a narrow corridor of parallel growth faults, as one, probably multiphase event, and is not compatible with separate mineralising fluids for each stratigraphic interval, introduced at different times and from different conduits as the basin architecture evolved with time.
- The relationship with faulting is consistent with the distribution of significant deposits in a linear, 10 to 25 km wide corridors in both Zambia and the DRC, each of which persists over strike lengths of 100 to 200 km. However, in addition, a large number of lesser Cu-Co occurrences are distributed across the Lufilian Arc beyond these corridors. The latter may be related to basinal brines that migrated up-sequence to be trapped by overlying reduced aquitards over large areas across the basin, but were not focussed like those fluids that were structurally channelled up fault conduits into traps bounded by the same faults;
- This second, pre-folding sulphide stage followed the precipitation of 'early' pyrite and introduction of mobile hydrocarbons, but preceded both the development of megabreccias in the Congolese Copperbelt, and folding associated with the main Lufilian orogenesis, which commenced at ~590 Ma and peaked between 560 to 530 Ma.
These observations would favour introduction of the main pre-folding Cu-mineralising fluid during the renewed extension, following deposition of the last stratigraphic interval hosting significant stratabound mineralisation (the Grand Conglomérat), although it is likely that, prior to this event, relatively passive circulation of basinal fluids has precipitated weak Cu mineralisation within the same pyritic and reduced host rocks.
The leaching and transport of the volume of copper estimated within the Copperbelt, would involve large scale convection cells, driven by a commensurate large scale energy source, as well as the development of an extensive system of structural permeability within the basement. It is unlikely that a passive tapping and percolation of fluids out of a tight schist/gneiss basement could yield this quantum of copper. The only potential pre-folding driver of such a system would be the renewed extension, with its inferred mafic underplating, consequent elevation of the thermal gradient and accompanying associated magmatism. In addition, this same period of extension resulted in displacement over deep extensional décollements within the basement, and the complex of branching listric faults which pass up into basinal growth faults. This structural activity would have increased the structural permeability of the basement, particularly between the base of the Katanga Supergroup and a deep extensional décollement(s).
In addition, if the Domes Region does represent an arcuate metamorphic core complex, or string of complexes, also formed during the renewed extension, they would enhance the structural porosity of the basement, and provide conduits for the transport of deeply sourced, hot fluids to shallower levels along their capping detachments, to be transferred into branching listric faults in the overlying brittlely distended upper plate. A genetic link between hydrothermal base and precious-metal mineralisation and fluid circulation, driven by high heat flow associated with metamorphic core complexes, has been documented in North America and Europe (e.g., Eliopoulos and Kilias, 2007; Spencer and Reynolds, 1986; Reynolds and Lister, 1987). The presence of talc-kyanite alteration along these detachment/décollements, may be interpreted to represent high-salinity, Mg-rich metasomatism associated with the passage of hot brines. In addition, the development of a string of deposits in carbonaceous schists in the hanging wall (e.g., Sentinel, Enterprise) and footwall (e.g., Malundwe and Chimiwungo at Lumwana) of detachments capping domes in the western Domes Region, are consistent with their being conduits for the postulated ingress of hot mineralised brines from basement, either during the renewed extension, or when reactivated as thrusts during the Lufilian Orogenic event.
In summary, it is suggested that renewed extension had greatly enhanced the structural permeability of that part of the crust above the main listric extensional detachment. In addition, the associated mafic underplating that accompanied the extension, and the consequent anatectic melting and bimodal magmatism resulted in an increase in the thermal gradient, as did the rise of the metamorphic core complexes which brought hot, deep, devolatolising rock below the detachment closer to the surface and added further fluids into the detachment. All of these processes drove thermohaline convection of brines formed from dissolution of the Roan Group salt/evaporite beds, which scavenged metals from the Katanga Supergroup and basement above the main listric extensional detachment, complemented by deep fluid release from below the detachment, plus cobalt and nickel rich contributions from the gabbroic intrusions. Fault dilatancy pumping mechanisms drove the composite brines up the detachment and into listric extensional growth faults that branch from the detachment, into the Katanga basin, and focussed them into the fluid traps with which the growth faults are intimately associated. These brines were saline, oxidised and sulphur deficient, and contained copper and cobalt attached to chloride ligands. Further west in the Domes region, the same fluids reacted with reduced, sulphate/sulphide rich rocks adjacent to the main detachment to deposit ore.
In the fluid trap zones, these fluids were reduced on contact with in situ or mobile hydrocarbons, decreasing the solubility of Cu and Co and releasing metals from solution. The released metals reacted with either 'early pyrite', or H2S in mobile hydrocarbons, or with anhydrite and hydrocarbons, to generate chalcopyrite. As the Mg and Mn in the brine was depleted, and replacement of pyrite progressed, the acidity of the reaction increased, inhibiting thermochemical sulphate reduction and the volume of sulphur available for reaction, leaving the only option to replace 'early pyrite', or progress up the copper enrichment chain by reacting with chalcopyrite to produce bornite (see the Reductants and Redox Boundaries and Zonation of sulphides sections above for reactions and implications).
• Megabreccia development in the Congolese Copperbelt - Following deposition of the second sulphide (and first main Cu) generation in the Congolese Copperbelt, the R.A.T., Mines and Dipeta subgroups underwent substantial structural mobilisation and internal deformation, such that in the mid to outer sections of the External Fold and Thrust Belt, these units were largely converted into a megabreccia. Within these megabreccias, coherent sections of Mines Subgroup rocks are only preserved as elongate, slabs or écailles, occurring as clasts within the megabreccia. Individual écailles generally represent the more brittle (silica-dolomite altered) units, and vary in size from a millimetre in diameter, to several km long by several hundred metres both down dip and in thickness, and are bounded by faults or breccia matrix on all sides. They are randomly set within a matrix of R.A.T. Subgroup and comminuted tectonic gravel- to silt-sized, to rock flour of the same lithologies, including talc, dolomite, albite, quartz and/or Mg-rich chlorite. Virtually all of the Mines Subgroup orebodies are hosted within écailles (see the Megabreccias section of the Congolese/Katangan Copperbelt record for detail).
The formation of these megabreccias has generally been assumed to accompany the compression, thrusting and nappe development of the Lufilian Orogeny (e.g., Selley et al., 2005; Hitzman et al., 2012). However, whilst the individual écailles have been rotated and redistributed, a gross structural alignment is still evident in some areas (e.g., the Dipeta Syncline in the Tenke-Fungurume window or within the core of the Luiswishi-Etoile anticline) which are not consistent with the Lufilian structural grain, suggesting the breccia development episode preceded the Lufilian folding. Similarly, the cores to a number of the sheared anticlinal structures of the Lufilian Arc, defined by Nguba and Kundelungu groups rocks (e.g., at Etoile-Ruishi, Luiswishi and Luishia), are occupied by synclines of Roan Group rocks whose axes are oblique to that of the anticline. In addition, the megabreccia development would appear to have accompanied collapse related to the main dissolution of the salt/evaporite bed(s) of the R.A.T. Subgroup, which produced the chaotic structure within the megabreccia. In contrast, the Lufilian folds, thrust planes and lithological boundaries bounding the megabreccia are relatively sharp and planar, which would not be expected if dissolution accompanied orogenesis, unless the salt dissolution deflation was very regular. It is therefore suggested the megabreccia formed at some time prior to the main Lufilian orogenic activity.
• Deformation and dissolution of salt/evaporite beds - Natural salt units are usually very fine grained with a very low permeability, and normally deform by plastic flow, which is ductile and non-dilatant. Consequently they are resistant to the entry of fluid, usually forming very effective fluid pressure seals (Magri et al., 2008; Urai et al., 2008). Dissolution normally takes place from the outer surfaces of the bed, without affecting its interior. Consequently, it is likely that salts and gypsum dissolved in this way to produce the early regional Ca-Mg and K-Mg alteration, left much of the major salt beds intact. Two processes are known to increase permeability in salt beds: i). micro-cracking and associated dilation; and ii). the formation of topologically connected brine-filled pores and triple-junction tubes in halite grain aggregates, both of which occur at a pressure and temperature corresponding to burial depths of >3 km. Such lithostatic fluid pressure increases the permeability of rock salt by many orders of magnitude, allowing ingress of fluids and dissolution to begin (Magri et al., 2008; Urai et al., 2008).
Studies of naturally deformed salt show that low temperature grain boundary dissolution-precipitation processes, such as solution-precipitation (or transfer) creep, water assisted grain boundary migration (dynamic recrystallisation) and grain boundary sliding are the principal means that influence the ductile characteristics of salt beds. Under conditions where micro-cracking allows free brine or water vapour access to the interior of a creeping salt mass, both recrystallisation and solution-precipitation creep effects can be strongly enhanced. The presence of small amounts of saturated grain boundary brine results in solution-precipitation creep becoming especially efficient, leading to quite rapid linear viscous deformation in rock salt (Magri et al., 2008; Urai et al., 2008).
It is therefore possible that during a late stage in the renewed extension, the interpreted R.A.T. halite beds were buried to a sufficient depth to generate micro-cracking and permeability, and allow ingress of basinal brines which greatly enhanced viscous flow and promoted gravity gliding, while also allowing dissolution of the salt bed to slump, deflate and dislocate the Roan Group, and promote diapiric thickening in the deepest part of the basin.
It is therefore suggested here, that renewed extension, uplift on the Domes metamorphic core complex (or complexes) and concomitant subsidence in the new depocentre to the north, produced a gradient which promoted gravity gliding over the ductile halite beds of the R.A.T. Subgroup at the base of the Roan group, and the R.G.S. Member of the Dipeta Subgroup. This resulted in transport of the Roan Group sequence, northward towards the main basin depocentre. As this proceeded, much of the salt beds underwent dissolution, collapse, and with the slumping of the sequence, the more competent, altered and mineralised Mines Subgroup was dislocated into a series of écailles which were enveloped by finer comminuted fragments of interbeds and the insoluble residue of the R.A.T. Subgroup salt bed(s). The overlying, less ductile Mwashya Subgroup and lower Nguba group were partially rafted with the Mines and Dipeta subgroups, but were structurally separated from the megabreccia mass by a detachment, now represented by a breccia zone at the base of the Kansuki Formation, the uppermost, less deformed unit of the Dipeta Subgroup that is conformably continuous with the overlying Mwashya Subgroup.
• Regional sodic-calcic alteration - Generation of regional sodic-calcic alteration principally affected the sequence above the Roan Group halite/evaporite, namely the overlying Upper Roan, Mwashya Subgroup, Nguba and Kundelungu groups, but also, locally the Lower Roan Subgroup rocks. It appears to have been active during the late renewed extension in the Domes and Katanga Core regions, but was accelerated and intensified during the main Lufilian Orogeny and late orogenic extensional collapse. This alteration may be related to two causes:
i). Accelerated dissolution of the Roan Group halite/evaporite sequence, forming concentrated brines that were subjected to thermohaline convection, resulting from the coupling of heat and dissolved halite, which have different rates of diffusion (i.e., heat diffuses faster than salt; Magri et al., 2008). In sedimentary basins, temperature gradients normally increase with depth. The presence of thick salt beds and diapirs influences the temperature gradient, as salt has a thermal conductivity two to four times greater than that of other sedimentary rocks. Consequently, salt masses buried in strata of much lower thermal conductivity will act as a conduit for heat transfer, both vertically and horizontally. This preferential path for heat conduction causes high-temperature anomalies, the magnitude of which depends upon the size, shape and depth of the salt mass (Magri et al., 2008).
Where the brines are derived from the upper surface of a salt mass, both temperature and salt concentration increases with depth in a basin not affected by other inhomogeneities such as large scale intrusions. The deeper brines are heated from below and are therefore less dense, triggering an upward fluid flow. As the flow progresses, the brines will cool, while loosing little salt, thereby diminishing the upward buoyancy force, until they eventually become more dense than water without dissolved salts and begin to sink. The converse occurs below a salt bed, where brines are denser than water without dissolved salts. The denser fluid will therefore sink into the deeper and hotter part of the basin (also known as diagenetic brine reflux at shallow depths). At the same time, lighter and hotter fluids will move upward to be replaced by the denser brines, until they are heated, or loose part of their dissolved salts, and begin to rise (Magri et al., 2008).
Thermohaline convection would also have influenced the earlier calcic-magnesian (as diagenetic brine reflux) and potassic alteration systems.
ii). Mingling of mantle derived mafic magma and anorogenic granitoids in an extensional setting, to produce iron oxide melts and hypersaline sodic-calcic brines carrying high concentrations of iron oxides. These processes are known to produce regional scale sodic-calcic and hematite alteration, and culminate in magnetite accumulations in large scale "iron oxide-alkali altered mineralised systems" that embrace apatite-magnetite and IOCG sensu stricto deposits (e.g., Porter, 2010; Chen et al., 2010). Mafic and felsic intrusions of these types developed during both the renewed extension (i.e., the gabbro and broadly coeval granitic to syenitic intrusions of the Domes Region of Zambia) and late Lufilian orogenesis (as described below), and are found in association with mineralisation in the Katanga Core (e.g., Mumbwa Cu-Au, Kalengwa Cu-Ag and Kasempa Fe), and most likely the regional Cu, Cu-Au, U and Fe occurrences distributed through the Domes Region and inner External Fold and Thrust Belt.
• First uranium pulse - As the depth of burial of the Roan Group salt/evaporites and the temperature and pressure increased, the composition of the brine generated evolved and uranium was scavenged from felsic minerals during convection, to be precipitated in reduced rocks through which the pregnant fluids were circulated, which included the hosts to earlier ore adjacent to the same associated fluid traps/pathways. Dating of uranium mineralisation suggests this first pulse occurred in the Congolese Copperbelt at ~670 to 620 Ma, prior to Lufilian orogenesis (e.g., Shinkolobwe, Luisha, Luiswishi, Monwezi; see the Geochronology of Ore section in the Congolese/Katangan Copperbelt record). This mineralising event is much more restricted than the Cu-Co ores of the Copperbelt, only being of economic significance in a small number of Cu-Co deposits and geochemically anomalous in others, whilst occurring without significant associated Cu-Co at other locations. A further generation of uranium mineralisation dated at 530 to 500 Ma is indicated during the Lufilian orogenesis, as described towards the end of the "Syn- and post-folding mineralisation generation" section below.
• Lufilian orogenic event - Onset of the composite, >100 m.y., Lufilian orogenic event, was related to the amalgamation of the supercontinent Pannotia, which commenced at ~600 Ma, and is regionally manifested by the Mozambique Belt to the east, part of the East African Orogen or Trans-Gondwana Super-mountains, formed by the east-west directed collision between West and East Gondwana (e.g., Meinhold et al., 2013). The stress field caused the oblique inversion of the NE-SW elongated Damara-Katanga rift basin system, comprising an initial NE vergent D1, compressional event, and a D2 stage of sinistral, branching, strike slip faults. The tectonic architecture was complicated by lateral mechanical constraints between the Kibara belt to the NW, and Bangweulu block to the east, in a transitional orogenic bending separating D1 and D2. The main period of orogenesis appears to have persisted to ~530 Ma.
Inversion progressively formed the Lufilian Arc, an arcuate structural element imposed upon the rocks of the Katanga sequence, substantially reactivating older structures and reflecting the earlier tectonic architecture in both the basement and Katanga Supergroup. The arc varies in character from an outer zone of thin skinned deformation (External Fold and Thrust Belt), only involving the Katanga Supergroup, which is separated from basement by shallow detachments, to an inner core of deep imbrication and thickening (Katanga Core), involving basement wedges, accompanied by syn- to post-tectonic felsic magmatism. The compressive phase was followed by a period of late orogenic extensional collapse between 530 and 500 Ma, and cooling to ~460 Ma or later.
• Lufilian magmatism - Porada and Berhorst (2000) suggest that during the Lufilian orogenic event, the Katanga Core represented a zone of imbrication related to NE directed thrusting involving deep crustal detachments and forward-propagating thrust faults that passed up into the overlying platform and slope deposits. In the Domes region, the Katangan platform sequence was detached from its basement and displaced NE over the buttressing domes, above extensions of these same detachments.
The Hook granite would appear to be the result of anatectic melting initiated by imbrication and crustal thickening during the main compressive stage. Imbrication weakened the bond between the upper crust and the sub-crustal lithospheric mantle (SCLM) allowing delamination. The SCLM had been isostatically depressed into the mantle during thickening and metamorphosed to a denser assemblage which was then detached and sank, allowing an upwelling of lighter decompression melting asthenosphere, which Milani et al. (2015) suggest occurred during the late stage of compression. Milani et al. (2015) suggest geochemical and isotopic evidence support the interaction between mantle components and portions of the deep crust at pressure of <10 kbar, where decompression melting of upwelling asthenospheric mantle ponded at the base of the crust, to heat, and ultimately melt and rework basement crustal material to form and propagate the voluminous granitic complex over a period of as much as 30 m.y., from ~560 to 530 Ma. They also suggest an additional and crucial contribution to the crustal melting was likely provided by internal radiogenic heat production of the thickened crust, which is in agreement with the highly radiogenic character of the pluton. Low-pressure mineral phases in metasedimentary wall rocks along the eastern margin of the pluton indicate that the magma was emplaced at shallow crustal depths (Milani et al., 2015).
Delamination and detachment of heavier SCLM culminated in uplift and erosion to the north of the Mwembeshi Shear Zone to expose the Hook Granite, while the same intrusions further north remained largely concealed.
Both Porada and Berhorst (2000) and Milani et al. (2015) suggest the zone of imbrication NE of the Mwembeshi Shear Zone is a result of subduction and collision between the Congo and Kalahari cratons. Milani et al. (2015) suggest the mantle underplating described above was preceded by subduction, then slab retreat and lithospheric mantle thinning, prior to asthenospheric upwelling. However, the absence of a clear indication of major calc-alkaline volcanic piles, and other signatures of subduction, would tend to favour the interpretation of inversion of an intracontinental rift basin between the two cratonic masses, as described above. This is also consistent with the presence in the basin of an earlier suite of extension related gabbroic intrusions and coeval thin mafic volcanic units intercalated with lesser felsic volcanics rocks in the middle of a thick sedimentary pile, part of the bimodal magmatism normally found in rift basins, rather than representing relict ophiolites and metamorphosed oceanic floor of a vanished ocean.
• Syn- and post-folding mineralisation generation - The Lufilian orogenic event represented a major, long-lived energy pulse, which i). reactivated many extensional structures as compressional thrusts; ii). generated new structural permeability and pathways in the Katanga sequence and basement rocks; iii). produced significant bimodal, but dominantly felsic, magmatism in the Katanga Core towards the end of the orogenic event; iv). progressively elevated the thermal gradient; v). accelerated dissolution of the remaining Roan group salt/evaporite beds and consequent generation of brines; vi). facilitated fault dilatancy pumping mechanisms extracting mineralised brines through the branching thrust network above deep basement detachments; and vii). drove thermohaline convective circulation of those brines which became more significant as the thermal gradient was increased and compression abated, in the transition to orogenic relaxation.
Fluid circulation systems allowed further leaching of metals from the basement and Katanga Supergroup, and the transport of those metals in sulphur-deficient, oxidised brines, to be deposited in reduced rocks where an available sulphur source existed. This led to regional potassic and sodic-calcic alteration and the deposition of new mineralisation, as well as both upgrading and remobilising pre-existing sulphide accumulations. In doing so, it initiated the third major sulphide generation, and the second main episode of copper mineralisation in the Central African Copperbelt.
Fluid inclusion studies from ore deposits in the Zambian Copperbelt (Greyling, 2009) recognised two main, but quite different fluid populations related to this generation of sulphide mineralisation. The first is a syn-folding phase, emplaced during early compression (>560 Ma), and is characterised by brines that were very similar to the early basinal fluids, but at higher temperatures of 150 to 260°C, and may have resulted in potassic and sodic alteration, depending on depth. This phase likely corresponds to the group of 585 to 575 Ma depositional ages evident in radiometric dating of samples from Nkana-Mindola, Chibuluma West and Nchanga in the main Zambian Copperbelt deposits and Kamoto in the DRC (see the Geochronology of ore sections).
The second, a late- to post-orogenic phase during orogenic relaxation (<530 Ma to ~500 Ma and younger), was much hotter, with saturated sodic-calcic brines at temperatures of up to ~400°C, fluid inclusions of which contain appreciable levels of Cu and Co. These brines were responsible for the widespread later episodes of sodic-calcic alteration seen throughout much of the Lufilian Arc, and likely corresponds to the group of 525 to 500 Ma depositional ages evident in radiometric dating of samples from Nkana-Mindola (in addition to the 585 to 575 Ma group), Musoshi and Kansanshi (see the Geochronology of ore sections).
Studies encountered from the Congolese Copperbelt have not differentiated syn- and post-folding phases, although El Desouky et al. (2009) measured temperatures of 270 to 385°C from coarser-grained, post-folding sulphides at Kamoto and Luiswishi.
The following observations point to the mode and conditions of deposition of sulphides during this syn- to post-folding generation of sulphide development, as follows:
- Mineralised veins (pre-, syn- and post-folding) are restricted to zones of disseminated mineralisation, and contain the same sulphide and alteration assemblages found within the enclosing disseminated mineralisation throughout the zoned sulphide assemblages (Hitzman et al., 2012, and references cited therein; Sillitoe et al., 2010).
- At a number of the key Zambian Copperbelt 'argillite-hosted' deposits (Luanshya, Nkana-Mindola, Chambishi and Nchanga-Chingola), the lower unit or units of the Copperbelt Orebody Member comprises schistose ore up to several metres or more thick, composed of sheared white micas, dolomite and anhydrite with variable discordant veinlets and shear foliation parallel bands and veins of chalcopyrite and bornite, and in some cases, representing the highest grade ores.
- Veins and veinlets can be most abundant at the bottom and/or tops of the stratabound orebodies, usually coinciding with rheologic contrasts and occupying shear zones and décollements that have stepped up from the basement to the boundaries between footwall siliciclastic and more argillaceous overlying host rocks.
- More than one generation of pyrite, chalcopyrite, carrollite, bornite and chalcocite are recognised in both the Zambian and Congolese copperbelts, represented both as disseminations and veins of late diagenetic, syn-tectonic and late- to post-tectonic origin.
- Hypogene sulphides of the syn- to post-folding generation display isotopic signatures that are either similar to those of the sulphides of the diagenetic pulse, or are comparable to that of Neoproterozoic sea water (at Luiswishi and Kamoto in the DRC; El Desouky et al., 2010), which those authors conclude, indicates sulphides of the more recent pulse obtained their sulphur from both early diagenetic sulphides and lesser thermochemical sulphate reduction.
- At the large low grade Kansanshi deposit, mineralisation appears to be almost entirely emplaced in the post-folding sulphide generation, suggesting this pulse added new Cu to the system, rather than just remobilising existing metal.
- Carbon and oxygen isotope signatures of dolomites associated with the syn- to post-folding generation of mineralisation, are mostly similar to those of the host rock and the dolomite of the diagenetic stage, which are interpreted to indicate the dolomites of the most recent pulse precipitated from a high-temperature, host rock-buffered fluid, possibly under the influence of thermochemical sulphate reduction (at Luiswishi and Kamoto in the DRC; El Desouky et al., 2010).
The following sequence of ore forming processes is therefore suggested for the syn- and post-folding generation of sulphide development:
- Oxidised Cu-Co bearing brines were introduced from below, along thrusts ramps and décollements connected to the compressive Lufilian basement detachment network. In the Zambian Copperbelt, basement thrusts and décollements passed up into, and were propagated along competency contrasts below folded, but not faulted, argillaceous rocks that comprised reduced, anhydrite-bearing and mineralised aquitards of the Roan (or Nguba) group. In the Congolese Copperbelt, it is likely that similar basement structures delivered brines into the overlying oxidised R.A.T. Subgroup, to permeate upwards to the R.A.T. Grises and reduced Mines Subgroup;
- These oxidised Cu-Co bearing brines were in equilibrium with early Roan Group oxidised facies in Zambia, and the similarly oxidised R.A.T. Subgroup in the Congolese Copperbelt, but released Cu-Co when coming into contact with the overlying reduced facies. In the DRC, the higher temperature brines, particularly the up to 385°C late-orogenic fluids, would have been sufficient hot to generate mobile hydrocarbons from the lower Mines Subgroup, to be released into, and reduce the upper few metres of the underlying R.A.T. Subgroup and form the R.A.T. Grises;
- The Cu-Co released was taken up to form sulphide minerals via three main routes:
i). reaction with hydrocarbons and anhydrite by thermochemical sulphate reduction (TSR) to produce copper sulphides and a carbonate gangue, requiring buffering by excess Mg, Mn and Fe to form dolomite and raise the pH so the reaction is not reversed (see the Reductants and Redox Boundaries section above for reactions). Sulphur from this reaction would give a TSR signature.
ii). reaction with existing pyrite and copper sulphides to produce copper sulphides with a higher Cu:S ratio (i.e., pyrite → chalcopyrite, chalcopyrite → bornite, etc.). These sulphides would retain the sulphur signature of the precursor sulphides, but would result in increased grades (see the Zonation of Ore Minerals section above for reactions). This process produces HCl and increases acidity, tending to inhibit TSR. The increased acidity would promote the dissolution and subsequent replacement of interstitial sulphate, carbonate cements, susceptible clastic grains and increase permeability, allowing the progress of the mineralising front into previously mineralised and cemented rocks (e.g., Borg et al., 2012 in the European Kupferschiefer). In carbonate-rich hosts (e.g., the Mines Subgroup) dissolved carbonates and silica would be redeposited as coarse crystals when buffered by the enclosing wall rocks to produce the characteristic silica-dolomite alteration in the Congolese Copperbelt.
iii). dissolution of pre-existing sulphides by the increasingly more acid fluids and redeposition by reaction with pre-existing sulphides or carbonate wall rocks.
- New sulphides would be concentrated as:
i). growth of new disseminated and fracture controlled veinlet sulphides by thermochemical sulphate reduction, replacing interstitial spaces, earlier non-sulphide grains, and filling fractures in the lithified rocks;
ii). quartz-carbonate veinlets in dilatant structures associated with competency changes on argillaceous host margins and décollements;
iii). coarsening grains and clusters nucleating on pre-existing earlier, pre-folding sulphides, cements and detrital grains;
iv). passive replacement and coating of fine, pre-existing pre-folding sulphides.
These prolonged processes would culminate in the alteration and mineralisation pervasively overprinting and obliterating earlier assemblages to equilibrate with the most recent fluids.
- It is likely that the syn-folding, early tectonic fluids were driven by tectonic 'fault-pumping', prior to the development of major anatectic magmatism, while during late- to post-folding, the latter then increased the thermal gradient and hydrothermal fluid supply to drive Thermohaline convection.
- The syn-folding phase probably corresponds to the onset of compression, whilst the peak compression from ~550 to 530 Ma would inhibit fluid flow, which was reinstated after 530 Ma by orogenic relaxation during the post-folding phase.
- The syn- and late- to post-folding generation of mineralisation was coeval with or followed the development of the characteristic folding of the Central African Copperbelt, in particular the axial plane thrusted anticlines that host many of the deposits of the inner External Fold and Thust Belt (e.g., Kambove, Luiswishi, Etoille) and the fault localised diapiric structures (e.g., Shinkolobwe, Menda, Kakanda, Kipushi) in the DRC. As such these structures may well have provided culminations or fluid escape pathways that channelled brines and influenced the location and degree of mineralisation.
- Post-folding fluids associated with, and permeating and partially emanating from, the highly radiogenic Lufilian felsic magmatism would appear to have contributed a second pulse of uranium mineralisation, dated at 530 to 500 Ma at Nkana-Mindola, Kansanshi, Musoshi, Luiswishi and Kamoto. However, early syn-folding ages of 580 to 550 Ma for uraninite have also been encountered at Kambove and Kamoto in the DRC.
- The influence of the syn-folding, early tectonic stage of brine generation, circulation and Cu-Co deposition is likely less intense than the late sodic-calcic high temperature brines which would have been more pervasive and more reactive. The signature of the latter is seen throughout the Lufilian Arc, upgrading existing and generating new mineralisation, in sediment hosted, IOCG and hybrid ore deposits in the Congolese and Zambian Copperbelts, Domes Region and Katanga Core.
• Domes Region Mineralisation - The Lumwana deposits are hosted within strongly metamorphosed and mylonitised rocks of the Mwombezhi Dome. Barrick (2014, pers. com.) suggest a pre- to syn-folding early generation of sulphide mineralisation, which utilises an anastomosing network of faults/shears that ramp from a basal detachment. These shears, which are accompanied by feldspar destructive alteration, straddle the contact between basement granite gneiss and a structurally underlying, overturned, interleaved sliver of metamorphosed Roan and Nguba groups rocks. Zoned mineralisation and alteration assemblages were established following late Lufilian remobilisation, or introduction of, sulphide and uranium minerals localised at redox boundaries. This may well reflect an early syn-folding phase, followed by late- to post-folding mineralisation, both using the major detachment zone which had been reactivated as a shear zone. The peak metamorphism in the surrounding dome is Lufilian aged, and accompanied shearing and complex deformation along the structural basement-Katanga Supergroup interface.
• Lead-zinc-silver-copper mineralisation appears to have been emplaced on a number of occassions. At Kipushi, SW of Lubumbashi in the DRC, mineralisation is hosted within a diapiric window of younger megabreccia developed from Roan, Nguba and Kundelungu groups rocks, and has been dated at 451.1±6 and 450.5±3.4 Ma by direct Rb-Sr and Re-Os dating of sulphides from two drill cores, respectively (Schneider et al., 2007) and would appear to represent a late generation of mineralisation. Pb isotopic compositions of ore galena from the Kabwe Pb-Zn deposit, hosted by Upper Roan Subgroup rocks to the south of the main Zambian Copperbelt in Zambia, has been estimated at 680±13 Ma (Kamona et al., 1999), whilst Cahen (1974) suggested a Pb model age of ~600 Ma for the Kengere Zn-Pb mineralisation, 50 km to the south of Kolwezi in the DRC, hosted by middle Nguba Group rocks (Kampunzu et al., 2009, and references cited therein).
* Footnote - Solubility of Copper - The following affect the solubility of copper in fluids:
• Salinity - copper is only very poorly soluble in pure water, but can be dissolved, attached to chloride ligands, in saline to hypersaline brines at suitable temperatures and oxidation conditions;
• Oxidation state - copper is readily dissolved in cooler (<250°C), oxidised saline to hypersaline brines (e.g., in sediment hosted Cu systems), but will be released from the fluid by reaction with reductants such as graphite, mobile and in situ hydrocarbons, H2S, sulphates (particularly anhydrite/gypsum), pyrite, pyrhotite and magnetite;
• Temperature - saline to hypersaline reduced to weakly oxidised brines may carry appreciable dissolved copper at temperatures of >250 to 350°C (e.g., in porphyry systems), to be precipitated when the temperature drops below this range;
• Acidity - cold (<250°C), oxidised, acid (pH 3 to 5) fluids (e.g., meteoric water) may dissolve and transport copper until neutralised (e.g., in leached capping and supergene sulphide blanket systems).
FULL REFERENCE LIST
Annels, A.E. 1989 - Ore Genesis in the Zambian Copperbelt, with Particular Reference to the Northern Sector of the Chambishi Basin: in Boyle R W, Brown, A.C., Jefferson, C.W., Jowett, E.C., Kirkham, R.V., (eds), Sediment-hosted Stratiform Copper Deposits: Geological Association of Canada, Special paper 36 pp 427-452.
Annels, A.E. 1984 - The Geotectonic Environment of Zambian Copper-cobalt Mineralization: in Journal of the Geological Society of London v141 pp 279-289.
Annels, A.E. 1974 - Some Aspects of the Stratiform Ore Deposits of the Zambian Copperbelt and their Genetic Significance: in Bartholome P (Ed), Gisements Stratiformes et Provinces Cupriferes: Societe Geologique de Belgique, Liege, pp 235-254.
Annels, A.E., 1979 - Mufulira Greywackes and their associated sulphides: in Trans. IMM, Section B v.88 pp B15-B23.
Annels, A.E., Vaughan D J, Craig J R 1983 - Conditions of Ore Mineral Formation in Certain Zambian Copperbelt deposits with Special Reference to the Role of Cobalt: in Mineralium Deposita, v18 pp 71-88.
Appleton, J.D., 1978, The geology of the Kabampo Gorge area: Zambian Geological Survey, Report no. 40.
Armstrong, R.A., Master, S and Robb, L.J., 2005 - Geochronology of the Nchanga Granite, and constraints on the maximum age of the Katanga Supergroup, Zambian Copperbelt : in J. of African Earth Sciences, v. 42 pp 32-40.
Armstrong, R.A., Robb, L.J., Master, S., Kruger, F.J., Mumba, P.A.C.C 1999 - New U-Pb age constraints on the Katangan Sequence, Central African Copperbelt: in Journal of African Earth Sciences, Elsevier, Amsterdam, v. 28, no.4A pp 6-7.
Arthurs, J. and Legg, C.A., 1974, The geology of the Solwezi area, explanation of degree sheet 1225, NW quarter: Geological Survey of Zambia, Report no. 36.
Barr, M.W.C., Cahen, L. and Ledent, D., 1977 - Geochronology of syntectonic granites from central Zambia: Lusaka Granite and granite NE of Rufunsa; Annales Societe Geologique Belgique, v.100, pp. 47-54.
Barr, M.W.C., Cahen, L. and Ledent, D. 1978 - Geochronology of syntectonic granites from central Zambia: Lusaka Granite and granite NE of Rufunsa. Annales Societe Geologique Belgique, v.100, pp. 47-54.
Barron, J.W., 2003, The stratigraphy, metamorphism, and tectonic history of the Solwezi area, Northwest Province, Zambia: Integrating geological field observations and airborne geophysics in the interpretation of regional geology: Unpublished Ph.D. thesis, Golden, Colorado School of Mines, 233p.
Bernau, R., 2007, The geology and geochemistry of the Lumwana Basment-hosted copper-cobalt (uranium) deposits, NW Zambia: Unpublished Ph.D. thesis, University of Southampton, School of Ocean and Earth Science, 187p.
Binda, P.L. and van Eden J G, 1972 - Sedimentological evidence on the origin of the Precambrian Great Conglomerate (Kundelungu Tillite), Zambia: Palaeogeography, Palaeoclimatology, Palaeoecology, v. 12, pp. 151-168.
Borg G, Piestrzynski A, Bachmann G H, Puttmann W, Walther S and Fiedler M 2012 - An Overview of the European Kupferschiefer Deposits: in Hedenquist J W, Harris M and Camus F, 2012 Geology and Genesis of Major Copper Deposits and Districts of the World - A tribute to Richard H Sillitoe, Society of Economic Geologists, Denver, Special Publication 16, pp. 455-486.
Borner, K., 2004 - Evaporite basins with emphasis on the Permian Zechstein; Course Notes, Institute of Geology, University of Mining and Technology, Freiberg, Germany, http://www.geo.tu-freiberg.de/oberseminar/os03_04 /Kristin%20B%F6rner.pdf, 10p.
Brandt, R.T., Burton, C.C.J., Maree, S.C., Woakes, M.E. 1961 - Mufulira: in Mendelsohn, F. (ed), Geology of the Northern Rhodesian Copperbelt McDonald, London pp 411-461.
Broughton, D.W., Hitzman, M.W., and Stephens, A.J., 2002, Exploration history and geology of the Kansanshi Cu-(Au) deposit, Zambia: Society of Economic Geologists, Special Publication 9, p. 141-153.
Brown, A.C., 2005 - Iron transport in redbeds during the genesis of sediment-hosted stratiform copper deposits; Mineral Deposit Research: Meeting the Global Challenge, Springer, pp 83-86.
Burnard, P.G., Sweeney, M.A., Vaughan, D.J., Spiro, B. and Thirlwall, M.F., 1993 - Sulfur and lead isotope constraints on the genesis of a southern Zambian massive sulphide deposit; Econ. Geol. v. 88, pp. 418-436.
Cahen, L., 1974 - Geological background of the copper-bearing strata of southern Shaba (Zaire); in: Bartholomé, P. (Ed.), Gisements stratiformes et provinces cupriferes. Centenaire de la Societe Geologique de Belgique, Liege, pp. 57–77.
Cailteux, J.L.H., Kampunzu, A.B. and Lerouge, C., 2007 - The Neoproterozoic Mwashya-Kansuki sedimentary rock succession in the central African Copperbelt, its Cu-Co mineralisation, and regional correlations: in Gondwana Research, v. 11 pp. 414-431.
Cailteux, J.L.H., Kampunzu, A.B. and Lerouge, C. Kaputo, A.K. and Milesi, J.P., 2005 - Genesis of sediment-hosted stratiform copper-cobalt deposits, Central African Copperbelt: in Journal of African Earth Sciences, v. 42 pp 134-158.
Cairney, T., Kerr, C.D., 1998 - The geology of the Kabwe area: explanation of degree sheet 1428, NW quarter; Report of the Geological Survey, Zambia, v. 47 40p.
Chen, H., Clark, A.H. and Kyser, T.K., 2010 - The Marcona Magnetite Deposit, Ica, South-Central Peru: A Product of Hydrous, Iron Oxide-Rich Melts?: Economic Geology, v. 105, pp. 1441-1456.
Croaker, M.R.D., 2011 - The Geology of the Nkana-Mindola sediment-hosted Copper-Cobalt Deposit, Zambian Copperbelt, Zambia; PhD Thesis, University of Tasmania, Australia, 363p.
Daly, M.C., Chakraborty, S.K., Kasolo, P., Musiwa, M., Mumba, P. Naidu. B., Namateba, C., Ngambi, O., Coward, M.P. 1984 - The Lufilian Arc and Irumide Belt of Zambia, Results of a Geotraverse across their Intersection: in Journal of African Earth Sciences, Elsevier, Amsterdam, v. 2, no.4, pp. 311-318.
Darnley, A.G. 1960 - Petrology of Some Rhodesian Copperbelt Orebodies and Associated Rocks: Transactions of the Institution of Mining and Metallurgy, v. 69 pp. 137-173.
De Waele, B., 2004 - The Proterozoic geological history of the Irumide belt, Zambia; PhD thesis, Curtin University, Western Australia.
De Waele, B. and Fitzsimons, I.C.W., 2007 - The nature and timing of Palaeoproterozoic sedimentation at the southeastern margin of the Congo Craton; zircon U-Pb geochronology of plutonic, volcanic and clastic unitsin northern Zambia; Precambrian Research, v. 159, pp. 95-116.
De Wit, M., 2009 - Copper Belt extends to Botswana; Inside Mining, v.2, June. 2009, pp. 22-26.
Ellis, M.W., Austen, A.L., Garlick, W.G., Gane, P.G, Cornwall, F.W. 1961 - Exploration: in Mendelsohn, F. (ed), Geology of the Northern Rhodesian Copperbelt, McDonald, London, pp 166-212.
Eliopoulos, D.G. and Kilias, S.P., 2007 - Hybrid Carlin gold and core complex exhumation sulphide mineralization: the Asimotrypes deposit of submicroscopic gold, Rhodope Massif, N. Greece; Proceedings of the Ninth Biennial SGA Meeting, Dublin 2007, pp. 617-620.
Fanning, D.S., Rabenhorst, M.C., Wagner, D.P., Lowery, D.3 and James, B.R., 2012 - Rethinking sulfidization and the role of hydrogen sulfide; in Osterholm, P., Yli-Halla, M. and Eden, P., (eds.), Geological Survey of Finland, Guide 56, pp. 44-46.
Fleischer, V.D. 1984 - Discovery, Geology and Genesis of Copper-cobalt Mineralisation at Chambishi Southeast Prospect, Zambia: in Precambrian Research, v. 25, no.1-3, pp. 119-133.
Fleischer, V.D., Garlick, W.G., Haldane, R., 1976 - Geology of the Zambian Copperbelt - (Excerpt on Genesis): in Wolf K H, (ed), Handbook of Strata-bound and Stratiform Deposits; II. Regional Studies and Specific Deposits Elsevier, Amsterdam, v.6, Cu, Zn, Pb and Ag Deposits, pp. 323-352.
Fleischer, V.D., Garlick, W.G., Haldane, R., 1976 - Geology of the Zambian Copperbelt (Exerpt covering Roan Antelope and Baluba): in Wolf K H, (ed), Handbook of Strata-bound and Stratiform Deposits; II. Regional Studies and Specific Deposits Elsevier, Amsterdam, v.6, Cu, Zn, Pb and Ag Deposits, pp. 285-297.
Fleischer, V.D., Garlick, W.G., Haldane, R., 1976 - Geology of the Zambian Copperbelt - Mufulira Mine, Zambia (Exerpt on Mufulira only): in Wolf K H, (ed), Handbook of Strata-bound and Stratiform Deposits; II. Regional Studies and Specific Deposits Elsevier, Amsterdam, v. 6, Cu, Zn, Pb and Ag Deposits, pp. 304-323.
Fleischer, V.D., Garlick, W.G., Haldane, R., 1976 - Geology of the Zambian Copperbelt (Exerpt covering Nkana): in Wolf K H, (ed), Handbook of Strata-bound and Stratiform Deposits; II. Regional Studies and Specific Deposits Elsevier, Amsterdam, v. 6, Cu, Zn, Pb and Ag Deposits, pp. 275-285.
Fleischer, V.D., Garlick, W.G., Haldane, R., 1976 - Geology of the Zambian Copperbelt (Excerpt covering Konkola and Musoshi): in Wolf K H, (ed), Handbook of Strata-bound and Stratiform Deposits; II. Regional Studies and Specific Deposits Elsevier, Amsterdam, v. 6, Cu, Zn, Pb and Ag Deposits, pp. 244-249.
Fleischer, V.D., Garlick, W.G., Haldane, R., 1976 - Geology of the Zambian Copperbelt (Exerpt covering Chibuluma and Chibuluma West): in Wolf K H, (ed), Handbook of Strata-bound and Stratiform Deposits; II. Regional Studies and Specific Deposits Elsevier, Amsterdam, v. 6, Cu, Zn, Pb and Ag Deposits, pp. 298-304.
Fleischer, V.D., Garlick, W.G., Haldane, R., 1976 - Geology of the Zambian Copperbelt (Excerpt covering the geology only): in Wolf K H, (ed), Handbook of Strata-bound and Stratiform Deposits; II. Regional Studies and Specific Deposits Elsevier, Amsterdam, v. 6, Cu, Zn, Pb and Ag Deposits, pp. 223-243.
Fleischer, V.D., Garlick, W.G., Haldane, R., 1976 - Geology of the Zambian Copperbelt (Exerpt covering Chambishi): in Wolf K H, (ed), Handbook of Strata-bound and Stratiform Deposits; II. Regional Studies and Specific Deposits Elsevier, Amsterdam, v. 6, Cu, Zn, Pb and Ag Deposits, pp. 249-256.
Fleischer, V.D., Garlick, W.G.,and Haldane, R., 1976 - Geology of the Zambian Copperbelt (Exerpt by Garlick and Haldane covering Nchanga): in Wolf K H, (ed), Handbook of Strata-bound and Stratiform Deposits; II. Regional Studies and Specific Deposits Elsevier, Amsterdam, v. 6, Cu, Zn, Pb and Ag Deposits, pp. 256-274.
Freeman, P.V., 1988 - Description of mineral deposits on the Copperbelt (and Kabwe, Nampundwe): Lukasa, Zambian Consolidated Copper Mines Ltd. (ZCCM) unpublished company report, 88p.
Garlick, W.G. 1961 - Chambishi: in Mendelsohn, F. (ed), Geology of the Northern Rhodesian Copperbelt McDonald, London, pp. 281-297.
Greyling, L.N., 2009 - Fluid evolution and characterisation of mineralising solutions in the Central African Copperbelt; Thesis submitted to the Faculty of Science, University of the Witwatersrand, Johannesburg, South Africa, in fulfilment of the requirements of the Degree of Doctor of Philosophy in Science, 278p.
Greyling, L.N., Robb, L.J., Master, S., Boiron, M.C. and Yao, Y., 2005 - The nature of early basinal fluids in the Zambian Copperbelt: A case study from the Chambishi deposit; Journal of African Earth Sciences, v. 42 pp. 159-172.
Gregory, J., Journet, N. and White, G., 2012 - Trident Project, Enterprise Nickel Prospect, North West Province Zambia; NI 43-101 Technical Report for the Mineral Resources of the Enterprise Nickel Prospect for First Quantum Mineral Limited, 181p.
Gunning, H.C., 1961 - The Early Years: in Mendelsohn, F. (ed), Geology of the Northern Rhodesian Copperbelt McDonald, London; pp. 3-10.
Hanson, R.E., Wardlaw, M.S., Wilson, T.J. and Mwaled, G., 1993 - U/Pb zircon ages from the Hook granite massif and Mwembeshi dislocation: constraints on Pan-African deformation, plutonism, and transcurrent shearing in Central Zambia; Precambrian Research, v. 63, pp. 189–209.
Hanson R E, Wilson T J and Munyanyiwa H, 1994 - Geologic evolution of the Neoproterozoic Zambezi Orogenic Belt in Zambia; Journal African Earth Sciences, v.18, pp. 135-150.
Haynes D W 1986 - Stratiform Copper deposits hosted by low-energy sediments: II. Nature of source rocks and composition of metal-transporting water; Economic Geology, v. 81 pp. 266-280.
Haynes D W 1986 - Stratiform Copper deposits hosted by low-energy sediments: I. Timing of Sulfide precipitation - an hypothesis; Economic Geology, v. 81, pp 250-265.
Hendrickson, M.D., 2013 - Geology of the Fishtie copper deposit, Central Province, Zambia; Unpub. M.S. thesis, Colorado School of Mines, Golden, CO.
Hendrickson, M.D., Hitzman, M.W., Wood, D., Humphrey, J.D. and Wendlandt, R.F. 2015 - Geology of the Fishtie deposit, Central Province, Zambia: iron oxide and copper mineralization in Nguba Group metasedimentary rocks; Mineralium Deposita, Online, DOI: 10.1007/s00126-014-0570-z.
Hitzman M W 1998 - Petrographic Studies of Ore Shale from the Zambian Copperbelt, Implications for Models of Ore Formation: in Geological Society of America, 1998 Annual Meeting Geological Society of America - Abstracts with Programs v30, No.7 pp 19-20.
Hitzman M W, Selley, D. and Bull S, 2010 - Formation of Sedimentary Rock-Hosted Stratiform Copper Deposits through Earth History; Economic Geology, v105 pp 627-639.
Hitzman, M.W., 2001 - Fe oxide-Cu-Au sytems in the Lufi lian Orogen of southern Africa; SEG Special Session I: Iron-Oxide(-Copper-Gold) Systems-Deposit Studies to Global Context, GSA Annual Meeting, November 5-8, 2001, Boston, Massachusetts, Abstract: 1p.
Hitzman M W, Broughton, D., Selley, D., Woodhead J, Wood D and Bull S, 2012 - The Central African Copperbelt: Diverse Stratigraphic, Structural, and Temporal Settings in the Worlds Largest Sedimentary Copper District: in Hedenquist J W, Harris M and Camus F, 2012 Geology and Genesis of Major Copper Deposits and Districts of the World - A tribute to Richard H. Sillitoe Society of Economic Geologists, Special Publication 16, pp. 487-514.
Hitzman M W, Kirkham R, Broughton, D., Thorson, J. and Selley, D., 2005 - The sediment-hosted stratiform copper ore system: in Hedenquist J W, Thompson J F H, Goldfarb R J and Richards, J.P. (Eds.), 2005 Economic Geology 100th Anniversary Volume, Society of Economic Geologists, Denver, pp. 609-642.
Intiomale, M.M., 1982 - Le gisement Zn–Pb–Cu de Kipushi (Shaba, Zaïre). Etude géologique et métallogénique; Ph.D. Thesis, Université Catholique de Louvain (Belgium).
John, T., Schenk, V., Mezger, K., and Tembo, F., 2004 - Timing and PT evolution of whiteschist metamorphism in the Lufilian arc-Zambezi belt orogen (Zambia): Implications for the assembly of Gondwana; Journal of Geology, v. 122, p. 71–90.
Jons, N. and Schenk, V., 2004 - Petrology of Whiteschists and Associated Rocks at Mautia Hill (Tanzania): Fluid Infiltration during High-Grade Metamorphism?; Journal of Petrology, online, doi:10.1093/petrology/egh044, 23p.
Jordaan, J. 1961 - Nkana: in Mendelsohn, F. (ed), Geology of the Northern Rhodesian Copperbelt McDonald, London pp 297-328.
Kamona, F., Friedrich, G., Sweeney, M.A., Fallick, A.E., 1991 - Stable isotopes of the Kabwe lead–zinc deposit. in Pagel, M., Leroy, J.L. (Eds.), Source, Transport and Deposition of Metals. Balkema, Rotterdam, pp. 313–316.
Kamona, A.F., Leveque, J., Friedrich, G., Haack, U., 1999 - Lead isotopes of the carbonate-hosted Kabwe, Tsumeb, and Kipushi Pb-Zn-Cu sulphide deposits in relation to Pan African orogenesis in the Damaran–Lufilian fold belt of Central Africa; Mineralium Deposita, v. 34, pp. 273–283.
Kampunzu, A.B. and Cailteux, J.L.H., 1999 - Tectonic Evolution of the Lufilian Arc (Central Africa Copper Belt) During Neoproterozoic Pan African Orogenesis; Gondwana Research, v.2, pp. 401-421.
Kampunzu, A.B., Cailteux, J.L.H., Kamona, A.F., Intiomale, M.M. and Melcher, F., 2009 - Sediment-hosted Zn–Pb–Cu deposits in the Central African Copperbelt; Ore Geology Reviews v.35 pp. 263-297.
Key, R.M., Liyungu, A.K,. Njamu, F.M., Somwe, V., Banda, J., Mosley, P.N. and Armstrong, R.A., 2001 - The western arm of the Lufilian Arc in NW Zambia and its potential for copper mineralization: in Journal of African Earth Sciences, v.33 pp. 503-528.
Kipata, M.L., 2013 - Brittle tectonics in the Lufilian fold and thrust belt and its foreland; An insight into the stress field record in relation to moving plates (Katanga, DRC); Doctor of Science, Geology, Thesis, Katholieke Universiteit Leuven, Groep Wetenschap & Technologie, Arenberg, Heverlee, Belgium, 182p.
Kirkham, R.V., (eds), Sediment-hosted Stratiform Copper Deposits Geological Association of Canada Special paper 36 pp 499-518.
Klinck, B.A., 1977 - The geology of the Kabompo Dome area, Explanation of Degree Sheet 1227 NE Quarter: Geological Survey of Zambia, no. 44.
Kortman, C.R., 1972 - The geology of the Zambia Broken Hill Mine, Kabwe; Geologie en Mijnbouw, v. 51, pp. 347–356.
Laghmouch, M., Fernandez, M.A. and Dewaele, S., 2012 - Uniform GIS‐Compilation (1: 750.000) of a geological map of the Kibara fold belt, Katanga, Democratic Republic of Congo; 4th International Geologica Belgica Meeting 2012, 1p.
Lobo-Guerrero. S.,A., 2005 - Pre- and post-Katangan granitoids of the greater Lufi lian Arc, geology, geochemistry, geochronology and metallogenic significance; unpublished Ph.D. thesis, Faculty of Science, University of the Witwatersrand, Johannesburg, South Africa, 734p.
Lobo-Guerrero, S.A., 2010 - Iron Oxide-Copper-Gold Mineralization in the Greater Lufi lian Arc, Africa; in Corriveau, L. and Mumin, H., (Eds.), Exploring for Iron Oxide Copper-Gold Deposits, Canada and Global Analogues; Geological Association of Canada, pp. 161-184.
Magri, F., Littke, R., Rodon, S., Bayer, U. and Urai, J., 2008 - Temperature fields, petroleum maturation and fluid flow in the vicinity of salt domes; in: Littke, R., Bayer, U., Gajewski, D. and Nelskamp, S. (Eds.), Dynamics of complex intracontinental basins: The Central European Basin System, Springer-Verlag, Berlin Heidelberg, pp. 277-290.
Marshall, T.R., Dyson, I.A. and Liu, K., 2007 - Petroleum systems in the Amadeus Basin, central Australia: Were they all oil prone?; in Munson, T.J. and Ambrose, G.J. (eds.), 2007 - Proceedings of the Central Australian Basins Symposium (CABS), Alice Springs, Northern Territory, 16-18 August, 2005, Northern Territory Geological Survey, Special Publication 2.
Master, S., Rainaud, C., Armstrong, R.A., Phillips D and Robb L J, 2005 - Provenance ages of the Neoproterozoic Katanga Supergroup (Central African Copperbelt), with implications for basin evolution: in J. of African Earth Sciences v42 pp 41-60.
Master, S., Rainaud, C., Armstrong, R.A., Philips D and Robb L J, 2005 - Provenance ages of the Neoproterozoic Katanga Supergroup (Central African Copperbelt), with implications for basin evolution: in J. of African Earth Sciences v.42 pp. 41-60.
McGowan R 2001 - Towards a New Genetic Model for Precambrian Sediment-hosted Copper Deposits: in A brief overview of the Zambian Copperbelt - Web page extract, from: www.soc.soton.ac.uk/SOES/STAFF/ sr1/Ross/RMcGowan_HP.htm 5p.
McKinnon D, Smit N J 1961 - Nchanga: in Mendelsohn, F. (ed), Geology of the Northern Rhodesian Copperbelt McDonald, London pp 234-275.
Meinhold, G., Morton, A.C. and Aviga, FD, 2013 - New insights into peri-Gondwana paleogeography and the Gondwana super-fan system from detrital zircon U-Pb ages; Gondwana Research, v. 23, pp. 661-665.
Mendelsohn, F. 1961 - Roan Antelope: in Mendelsohn, F. (ed), Geology of the Northern Rhodesian Copperbelt McDonald, London pp 351-405.
Mendelsohn, F. 1989 - Central/Southern African Ore Shale Deposits: in Boyle R W, Brown A C, Jefferson C W, Jowett E C, Kirkham R V, (eds), Sediment-hosted Stratiform Copper Deposits Geological Association of Canada Special paper 36 pp 453-469.
Mendelsohn, F., Garlick, W.G., Pienaar P J, Demesmaeker G 1961 - General Geology (Extract): in i>Mendelsohn, F., (Ed.), Geology of the Northern Rhodesian Copperbelt McDonald, London pp 17-56.
Milani, L., Lehmann, J., Naydenov, K.V., Saalmann, K., Kinnaird, J.A., Daly, J.S. and Lobo-Guerrero, S.A., 2015 - A-type magmatism in a syn-collisional setting: The case of the Pan-African Hook Batholith in Central Zambia; Lithos, v. 216–217, pp. 48-72.
Moore, T.A., 1964 - The geology of the Chisamba area: explanation of degree sheet 1428, SW quarter; Report of the Geological Survey. Zambia v. 5, 32p.
Moore C M, Danio J R and Altman K A, 2012 - Technical Report on the Lumwana Mine, North Western Province, Republic of Zambia; NI 43-101 Technical Report by Roscoe Postle Associates Inc. for Barrick Gold Corporation.
Mulelua, D., and Seifert, A.V., 1998, Geology of the Mwombezhi Dome and Jiwundu Swamp areas: Explanation of degree sheet 1225 NE quarter and 1125, part of SE quarter: Report of the Geological Survey of Zambia, no. 83, 28 p.
Naydenov, K., Lehmann, J., Saalmann, K., Milani, L., Kinnaird, J., Charlesworth, G. and Frei, D., 2013 - Structural study and geochronology in the Hook Batholith, Central Zambia; American Geophysical Union, Fall Meeting 2013, abstract only.
Nisbet, B., Cooke, J., Richards, M. and Williams, C., 2000 - Exploration for Iron Oxide Copper Gold Deposits in Zambia and Sweden: Comparison with the Australian Experience; in Porter, T. M., (ed.), Hydrothermal Iron Oxide Copper-gold and Related Deposits: A Global Perspective, PGC Publishing, Adelaide, v. 1, pp. pp 297-308.
Porada, H. and Berhorst V, 2000 - Towards a new understanding of the Neoproterozoic-Early Palaeozoic Lufilian and northern Zambezi Belts in Zambia and the Democratic Republic of Congo: in Journal of African Earth Sciences, v.30 pp. 727-771.
Porada, H. and Druschel, G., 2010 - Evidence for participation of microbial mats in the deposition of the siliciclastic ‘ore formation’ in the Copperbelt of Zambia: Journal of African Earth Sciences v.58 pp. 427-444.
Porter, T.M., 2010 - Current understanding of iron oxide associated-alkali altered mineralised systems: Part I, An Overview; in Porter, T.M., (ed.), Hydrothermal Iron Oxide Copper-Gold and Related Deposits: A Global Perspective, v. 3 - Advances in the Understanding of IOCG Deposits; PGC Publishing, Adelaide, pp. 5-32.
Rainaud, C., Master, S., Armstrong, R.A. and Robb L J, 2005 - Geochronology and nature of the Palaeoproterozoic basement in the Central African Copperbelt (Zambia and the Democratic Republic of Congo), with regional implications: in Journal of African Earth Sciences v42 pp 1-31.
Rainaud, C., Master, S., Armstrong, R.A. and Robb L J, 2005 - Geochronology and nature of the Palaeoproterozoic basement in the Central African Copperbelt (Zambia and the Democratic Republic of Congo), with regional implications: in Journal of African Earth Sciences v.42 pp. 1-31.
Rainaud, C., Master, S., Armstrong, R.A., Phillips D and Robb L J, 2005 - Monazite U-Pb dating and 40Ar-39Ar thermochronology of metamorphic events in the Central African Copperbelt during the Pan-African Lufilian Orogeny: in Journal of African Earth Sciences v.42 pp. 183-199.
Reynolds, S.J. and Lister, G.S., 1987 - Structural aspects of fluid-rock interactions in detachment zones; Geology, v. 15, pp. 362-366.
Richards, J.P., Cumming G L, Krstic D, Wagner P A, Spooner E T C 1988 - Pb isotope constraints on the age of Sulfide ore deposition and U-Pb age of late Uraninite veining at the Musoshi stratiform Copper deposit, central African Copper belt, Zaire; Economic Geology, v83 pp 724-741.
Richards, J.P., Krogh T E, Spooner E T C 1988 - Fluid inclusion characteristics and U-Pb Rutile age of late hydrothermal alteration and veining at the Musoshi stratiform copper deposit, central African Copper belt, Zaire; Economic Geology, v83 pp 118-139.
Schneider, J., Melcher, F., Brauns, M., 2007 - Concordant ages for the giant Kipushi base metal deposit (DR Congo) from direct Rb–Sr and Re–Os dating of sulphides; Mineralium Deposita, v. 42, pp. 791–797.
Selley, D., Broughton, D., Scott R, Hitzman M, Bull S, Large R, McGoldrick P, Croaker M and Pollington N, 2005 - A new look at the geology of the Zambian Copperbelt: in Economic Geology, 100 Anniversary Volume, Society of Economic Geologists, pp. 965-1000.
Sillitoe R H, Perello J and Garcia A, 2010 - Sulfide-Bearing Veinlets Throughout the Stratiform Mineralization of the Central African Copperbelt: Temporal and Genetic Implications; Econ. Geol. v105 pp 1361-1368.
Sutton, S.J. and Maynard, J.B., 2005 - A fluid mixing model for copper mineralization at Konkola North, Zambian Copperbelt : in J. of African Earth Sciences v42 pp 95-118.
Sweeney, M.A. and Binda, P.L. 1989 - The Role of Diagenesis in the Formation of the Konkola Cu-Co Orebody of the Zambian Copperbelt: in Boyle, R.W., Brown, A.C., Jefferson, C.W., Jowett, E.C.
Schoonen, M.A.A., 2004 - Mechanisms of sedimentary pyrite formation; in Amend, J.P., Edwards, K.J., and Lyons, T.W., eds., Sulfur biogeochemistry - Pastand present: Geological Society of America, Boulder, Colorado, Special Paper 379, pp. 117–134.
Spencer, J.E. and Reynolds, S.J., 1986 - Some aspects of the middle Tertiary tectonics of Arizona and southeastern California; Arizona Geological Society Digest v. 15, pp. 102-107.
Sweeney, M.A., Binda, P.L. 1994 - Some Constraints on the Formation of the Zambian Copperbelt Deposits: in J. of African Earth Sciences v19 pp 303-313.
Sweeney, M.A., Binda, P.L., Vaughan D J 1991 - Genesis of the Ores of the Zambian Copperbelt: in Ore Geology Reviews v6 pp 51-76.
Sweeney M, Turner P, Vaughan D J 1986 - Stable isotope and geochemical studies of the role of early diagenesis in ore formation, Konkola Basin, Zambian Copper belt; Economic Geology, v81 pp 1838-1852.
Tack, L., Wingate, M .T.D., De Waele, B., Meert, J., Belousova, E., Griffin, B., Tahon, A. and Fernandez-Alonso, M., 2010 - The 1375 Ma “Kibaran event” in Central Africa: Prominent emplacement of bimodal magmatism under extensional regime; Precambrian Research, v. 180, pp. 63-84.
Taylor, J.H., 1954 - The lead–zinc–vanadium deposits at Broken Hill, Northern Rhodesia; Colonial Geology and Mineral Resources v. 4, pp. 335–365.
Thompson, A.B., 1999 - Some time-space relationships for crustal melting and granitic intrusion at various depths; in Castro, A., Fernandez, C. and Vigneresse, J.L., (eds.), Understanding Granites; Integrated new and Classical Techniques, Geological Society of London, Special Publications, v. 168, pp. 7-25.
Unrug R 1988 - Mineralization Controls and Source of Metals in the Lufilian Fold Belt, Shaba (Zaire), Zambia and Angola; Economic Geology, v83 pp 1247-1258.
Unrug R 1989 - LANDSAT-based Structural Map of the Lufilian Fold Belt and the Kundelungu Aulacogen, Shaba (Zaire), Zambia and Angola, and the Regional Position of Cu, Co, U, Au, Zn and Pb Mineralisation: in Boyle R W, Brown A C, Jefferson C W, Jowett E C, Kirkham R V, (eds), Sediment-hosted Stratiform Copper Deposits Geological Association of Canada Special paper 36 pp 519-524.
Unrug R 1988 - Mineralization controls and source of metals in the Lufilian Fold Belt, Shaba (Zaire), Zambia, and Angola; Economic Geology, v83 pp 1247-1258.
Urai, J., Schleder, Z., Spiers, C. and Kukla, P.A., 2008 - Flow and Transport Properties of Salt Rocks; in: Littke, R., Bayer, U., Gajewski, D. and Nelskamp, S. (Eds.), Dynamics of complex intracontinental basins: The Central European Basin System, Springer-Verlag, Berlin Heidelberg, pp. 277-290.
van Eden J G 1974 - Deposition and Diagenetic Environment Related to Sulfide Mineralization, Mufulira, Zambia; Economic Geology, v69 pp 59-79.
Van Wilderode, J., El Desouky, H.A., Elburg, M.A., Vanhaecke, F. and Muchez, P., 2014 - Metal sources for the Katanga Copperbelt deposits (DRC): insights from Sr and Nd isotope; ratios; Geologica Belgica, v. 17, pp. 137-147.
Wendorff M, 2005 - Sedimentary genesis and lithostratigraphy of Neoproterozoic megabreccia from Mufulira, Copperbelt of Zambia: in Journal of African Earth Sciences v42 pp 61-81.
Winfield O 1961 - Chibuluma: in Mendelsohn, F. (ed), Geology of the Northern Rhodesian Copperbelt McDonald, London pp 328-342.
Zientek, M.L., Bliss, J.D., Broughton, D.W., Christie, Michael, Denning, P.D., Hayes, T.S., Hitzman, M.W., Horton, J.D., Frost-Killian, Susan, Jack, D.J., Master, Sharad, Parks, H.L., Taylor, C.D., Wilson, A.B., Wintzer, N.E. and Woodhead, Jon, 2014 - Sediment-Hosted stratabound copper assessment of the Neoproterozoic Roan Group, Central African Copperbelt, Katanga Basin, Democratic Republic of the Congo and Zambia; U.S. Geological Survey Scientific Investigations Report 2010–5090–T, 162p., and spatial data, http://dx.doi.org/10.3133/sir20105090T.
Zientek M L, Hayes T S and Hammarstrom J M 2013 - Overview of a New Descriptive Model for Sediment- Hosted Stratabound Copper Deposits: in Zientek M L, Hammarstrom J M and Johnson K M, 2013 Descriptive Models, Grade-Tonnage Relations, and Databases for the Assessment of Sediment-Hosted Copper Deposits - With Emphasis on Deposits in the Central African Copperbelt, Democratic Republic of the Congo and Zambia USGS Scientific Investigations, Report 2010-5090-J pp. 2-16.
The most recent source geological information used to prepare this decription was dated: 2015.
Record last updated: 27/4/2015
This description is a summary from published sources, the chief of which are listed below. © Copyright Porter GeoConsultancy Pty Ltd. Unauthorised copying, reproduction, storage or dissemination prohibited.
|
|
Annels A E 1984 - The Geotectonic Environment of Zambian Copper-cobalt Mineralization: in Journal of the Geological Society of London v141 pp 279-289
|
Annels A E 1989 - Ore Genesis in the Zambian Copperbelt, with Particular Reference to the Northern Sector of the Chambishi Basin: in Boyle R W, Brown A C, Jefferson C W, Jowett E C, Kirkham R V, (eds), Sediment-hosted Stratiform Copper Deposits: Geological Association of Canada Special paper 36 pp 427-452
|
Annels A E 1974 - Some Aspects of the Stratiform Ore Deposits of the Zambian Copperbelt and their Genetic Significance: in Bartholome P (Ed), Gisements Stratiformes et Provinces Cupriferes: Societe Geologique de Belgique, Liege pp 235-254
|
Annels A E, 1979 - Mufulira Greywackes and Their Associated Sulphides: in Trans. IMM, Section B v.88 pp B15-B23
|
Annels A E, Vaughan D J, Craig J R 1983 - Conditions of Ore Mineral Formation in Certain Zambian Copperbelt deposits with Special Reference to the Role of Cobalt: in Mineralium Deposita v18 pp 71-88
|
Armstrong R A, Robb L J, Master S, Kruger F J, Mumba P A C C 1999 - New U-Pb age constraints on the Katangan Sequence, Central African Copperbelt: in Journal of African Earth Sciences, Elsevier, Amsterdam v28, no.4A pp 6-7
|
Armstrong, R.A., Master, S. and Robb, L.J., 2005 - Geochronology of the Nchanga Granite, and constraints on the maximum age of the Katanga Supergroup, Zambian Copperbelt: in J. of African Earth Sciences v.42, pp, 32-40,
|
Brandt R T, Burton C C J, Maree S C, Woakes M E 1961 - Mufulira: in Mendelsohn F (ed), Geology of the Northern Rhodesian Copperbelt McDonald, London pp 411-461
|
Bull S, Selley D, Broughton D, Hitzman M, Cailteux J, Large R and McGoldrick, 2011 - Sequence and carbon isotopic stratigraphy of the Neoproterozoic Roan Group strata of the Zambian copperbelt: in Precambrian Research v.190 pp. 70-89
|
Cailteux J L H, Kampunzu A B and Lerouge C, 2007 - The Neoproterozoic Mwashya-Kansuki sedimentary rock succession in the central African Copperbelt, its Cu-Co mineralisation, and regional correlations: in Gondwana Research v.11 pp. 414-431
|
Cailteux J L H, Kampunzu A B, Lerouge C, Kaputo A K and Milesi J P, 2005 - Genesis of sediment-hosted stratiform copper-cobalt deposits, Central African Copperbelt: in J. of African Earth Sciences v42 pp 134-158
|
Daly M C, Chakraborty S K, Kasolo P, Musiwa M, Mumba P. Naidu B, Namateba C, Ngambi O, Coward M P 1984 - The Lufilian Arc and Irumide Belt of Zambia, Results of a Geotraverse across their Intersection: in Journal of African Earth Sciences, Elsevier, Amsterdam v2, no.4 pp 311-318
|
Darnley A G 1960 - Petrology of Some Rhodesian Copperbelt Orebodies and Associated Rocks: in Transactions of the Institution of Mining and Metallurgy v69 pp 137-173
|
Davey, J., Roberts, S. and Wilkinson, J.J., 2020 - Copper- and cobalt-rich, ultrapotassic bittern brines responsible for the formation of the Nkana-Mindola deposits, Zambian Copperbelt: in Geology v.49, 5p. doi.org/10.1130/G48176.1
|
Ellis M W, Austen A L, Garlick W G, Gane P G, Cornwall F W 1961 - Exploration: in Mendelsohn F (ed), Geology of the Northern Rhodesian Copperbelt McDonald, London pp 166-212
|
Fleischer V D 1984 - Discovery, Geology and Genesis of Copper-cobalt Mineralisation at Chambishi Southeast Prospect, Zambia: in Precambrian Research v25, no.1-3 pp 119-133
|
Fleischer V D, Garlick W G, Haldane R 1976 - Geology of the Zambian Copperbelt - Mufulira Mine, Zambia (Exerpt on Mufulira only): in Wolf K H, (ed), Handbook of Strata-bound and Stratiform Deposits; II. Regional Studies and Specific Deposits Elsevier, Amsterdam v6, Cu, Zn, Pb and Ag Deposits pp 304-323
|
Fleischer V D, Garlick W G, Haldane R 1976 - Geology of the Zambian Copperbelt (Excerpt covering the geology only): in Wolf K H, (ed), Handbook of Strata-bound and Stratiform Deposits; II. Regional Studies and Specific Deposits Elsevier, Amsterdam v6, Cu, Zn, Pb and Ag Deposits pp 223-243
|
Fleischer V D, Garlick W G, Haldane R 1976 - Geology of the Zambian Copperbelt - (Excerpt on Genesis): in Wolf K H, (ed), Handbook of Strata-bound and Stratiform Deposits; II. Regional Studies and Specific Deposits Elsevier, Amsterdam v6, Cu, Zn, Pb and Ag Deposits pp 323-352
|
Fleischer V D, Garlick W G, Haldane R 1976 - Geology of the Zambian Copperbelt (Exerpt covering Chambishi): in Wolf K H, (ed), Handbook of Strata-bound and Stratiform Deposits; II. Regional Studies and Specific Deposits Elsevier, Amsterdam v6, Cu, Zn, Pb and Ag Deposits pp 249-256
|
Fleischer V D, Garlick W G, Haldane R 1976 - Geology of the Zambian Copperbelt (Exerpt covering Chibuluma and Chibuluma West): in Wolf K H, (ed), Handbook of Strata-bound and Stratiform Deposits; II. Regional Studies and Specific Deposits Elsevier, Amsterdam v6, Cu, Zn, Pb and Ag Deposits pp 298-304
|
Fleischer V D, Garlick W G, Haldane R 1976 - Geology of the Zambian Copperbelt (Exerpt covering Nkana): in Wolf K H, (ed), Handbook of Strata-bound and Stratiform Deposits; II. Regional Studies and Specific Deposits Elsevier, Amsterdam v6, Cu, Zn, Pb and Ag Deposits pp 275-285
|
Fleischer V D, Garlick W G, Haldane R 1976 - Geology of the Zambian Copperbelt (Excerpt covering Konkola and Musoshi): in Wolf K H, (ed), Handbook of Strata-bound and Stratiform Deposits; II. Regional Studies and Specific Deposits Elsevier, Amsterdam v6, Cu, Zn, Pb and Ag Deposits pp 244-249
|
Fleischer V D, Garlick W G, Haldane R 1976 - Geology of the Zambian Copperbelt (Exerpt covering Roan Antelope and Baluba): in Wolf K H, (ed), Handbook of Strata-bound and Stratiform Deposits; II. Regional Studies and Specific Deposits Elsevier, Amsterdam v6, Cu, Zn, Pb and Ag Deposits pp 285-297
|
Fleischer V D, Garlick W G,and Haldane R 1976 - Geology of the Zambian Copperbelt (Exerpt by Garlick and Haldane covering Nchanga): in Wolf K H, (ed), Handbook of Strata-bound and Stratiform Deposits; II. Regional Studies and Specific Deposits Elsevier, Amsterdam v6, Cu, Zn, Pb and Ag Deposits pp 256-274
|
Fleischer, V.D., Garlick. W.G. and Haldane, R., 1976 - Geology of the Zambian Copperbelt: in Wolf, K,H,, (ed,.), Handbook of Strata-bound and Stratiform Deposits; II. Regional Studies and Specific Deposits, Elsevier, Amsterdam, v.6, Cu, Zn, Pb and Ag Deposits, Chapter 6, pp. 223-352.
|
Garlick W G 1961 - Chambishi: in Mendelsohn F (ed), Geology of the Northern Rhodesian Copperbelt McDonald, London pp 281-297
|
Greyling L N, Robb L J, Master S, Boiron M C and Yao Y, 2005 - The nature of early basinal fluids in the Zambian Copperbelt: A case study from the Chambishi deposit: in J. of African Earth Sciences v.42, pp. 159-172,
|
Gunning H C 1961 - The Early Years: in Mendelsohn F (ed), Geology of the Northern Rhodesian Copperbelt McDonald, London pp 3-10
|
Halley, S.W., Wood, D., Stoltze, A., Godfroid, J., Goswell, H. and Jack, D., 2016 - Using Multielement Geochemistry to Map Multiple Components of a Mineral System: Case Study from a Sediment-Hosted Cu-Ni Camp, NW Province, Zambia: in SEG Newsletter, No. 104, January, 2016, pp. 1, 15-21.
|
Haynes D W 1986 - Stratiform Copper deposits hosted by low-energy sediments: II. Nature of source rocks and composition of metal-transporting water: in Econ. Geol. v81 pp 266-280
|
Haynes D W 1986 - Stratiform Copper deposits hosted by low-energy sediments: I. Timing of Sulfide precipitation - an hypothesis: in Econ. Geol. v81 pp 250-265
|
Hitzman M W 1998 - Petrographic Studies of Ore Shale from the Zambian Copperbelt, Implications for Models of Ore Formation: in Geological Society of America, 1998 Annual Meeting Geological Society of America - Abstracts with Programs v30, No.7 pp 19-20
|
Hitzman M W, Kirkham R, Broughton D, Thorson J and Selley D, 2005 - The sediment-hosted stratiform copper ore system: in Hedenquist J W, Thompson J F H, Goldfarb R J and Richards J P (Eds.), 2005 Economic Geology 100th Anniversary Volume, Society of Economic Geologists, Denver, pp. 609-642
|
Hitzman, M.W., Selley, D. and Bull, S., 2010 - Formation of Sedimentary Rock-Hosted Stratiform Copper Deposits through Earth History : in Econ. Geol. v.105, pp. 627-639.
|
Hitzman, M.W., Broughton, D., Selley, D., Woodhead, J., Wood, D. and Bull, S., 2012 - The Central African Copperbelt: Diverse Stratigraphic, Structural, and Temporal Settings in the Worlds Largest Sedimentary Copper District: in Hedenquist, J.W., Harris, M. and Camus, F., 2012 Geology and Genesis of Major Copper Deposits and Districts of the World - A tribute to Richard H Sillitoe Society of Economic Geologists Special Publication 16, pp. 487-514.
|
Jordaan J 1961 - Nkana: in Mendelsohn F (ed), Geology of the Northern Rhodesian Copperbelt McDonald, London pp 297-328
|
Kampunzu A B and Cailteux J L H, 1999 - Tectonic Evolution of the Lufilian Arc (Central Africa Copper Belt) During Neoproterozoic Pan African Orogenesis: in Gondwana Research v.2 pp. 401-421
|
Key R M, Liyungu A K, Njamu F M, Somwe V, Banda J, Mosley P N and Armstrong R A, 2001 - The western arm of the Lufilian Arc in NW Zambia and its potential for copper mineralization: in J. of African Earth Sciences v.33 pp. 503-528.
|
Master S, Rainaud C, Armstrong R A, Phillips D and Robb L J, 2005 - Provenance ages of the Neoproterozoic Katanga Supergroup (Central African Copperbelt), with implications for basin evolution: in J. of African Earth Sciences v42 pp 41-60
|
Master S, Rainaud C, Armstrong R A, Philips D and Robb L J, 2005 - Provenance ages of the Neoproterozoic Katanga Supergroup (Central African Copperbelt), with implications for basin evolution: in J. of African Earth Sciences v.42 pp. 41-60
|
McGowan R 2001 - Towards a New Genetic Model for Precambrian Sediment-hosted Copper Deposits: in A brief overview of the Zambian Copperbelt - Web page extract, from: www.soc.soton.ac.uk/SOES/STAFF/ sr1/Ross/RMcGowan_HP.htm 5p
|
McKinnon D, Smit N J 1961 - Nchanga: in Mendelsohn F (ed), Geology of the Northern Rhodesian Copperbelt McDonald, London pp 234-275
|
Mendelsohn F 1989 - Central/Southern African Ore Shale Deposits: in Boyle R W, Brown A C, Jefferson C W, Jowett E C, Kirkham R V, (eds), Sediment-hosted Stratiform Copper Deposits Geological Association of Canada Special paper 36 pp 453-469
|
Mendelsohn F 1961 - Roan Antelope: in Mendelsohn F (ed), Geology of the Northern Rhodesian Copperbelt McDonald, London pp 351-405
|
Mendelsohn F, Garlick W G, Pienaar P J, Demesmaeker G 1961 - General Geology (Extract): in Mendelsohn F (Ed.), Geology of the Northern Rhodesian Copperbelt McDonald, London pp 17-56
|
Porada H and Berhorst V, 2000 - Towards a new understanding of the Neoproterozoic-Early Palaeozoic Lufilian and northern Zambezi Belts in Zambia and the Democratic Republic of Congo: in J. of African Earth Sciences v.30 pp. 727-771
|
Porada H and Druschel G, 2010 - Evidence for participation of microbial mats in the deposition of the siliciclastic ore formation in the Copperbelt of Zambia: in J. of African Earth Sciences v.58 pp. 427-444
|
Rainaud C, Master S, Armstrong R A and Robb L J, 2005 - Geochronology and nature of the Palaeoproterozoic basement in the Central African Copperbelt (Zambia and the Democratic Republic of Congo), with regional implications: in J. of African Earth Sciences v.42 pp. 1-31
|
Rainaud C, Master S, Armstrong R A and Robb L J, 2003 - A cryptic Mesoarchaean terrane in the basement to the Central African Copperbelt: in Journal of the Geological Society, London v.160 pp 11-14
|
Rainaud C, Master S, Armstrong R A, Phillips D and Robb L J, 2005 - Monazite U-Pb dating and 40Ar-39Ar thermochronology of metamorphic events in the Central African Copperbelt during the Pan-African Lufilian Orogeny: in J. of African Earth Sciences v.42 pp. 183-199
|
Richards J P, Cumming G L, Krstic D, Wagner P A, Spooner E T C 1988 - Pb isotope constraints on the age of Sulfide ore deposition and U-Pb age of late Uraninite veining at the Musoshi stratiform Copper deposit, central African Copper belt, Zaire: in Econ. Geol. v83 pp 724-741
|
Richards J P, Krogh T E, Spooner E T C 1988 - Fluid inclusion characteristics and U-Pb Rutile age of late hydrothermal alteration and veining at the Musoshi stratiform copper deposit, central African Copper belt, Zaire: in Econ. Geol. v83 pp 118-139
|
Selley D, Broughton D, Scott R, Hitzman M, Bull S, Large R, McGoldrick P, Croaker M and Pollington N, 2005 - A new look at the geology of the Zambian Copperbelt: in Economic Geology, 100 Anniversary Volume, Society of Economic Geologists, pp. 965-1000
|
Sillitoe R H, Perello J and Garcia A, 2010 - Sulfide-Bearing Veinlets Throughout the Stratiform Mineralization of the Central African Copperbelt: Temporal and Genetic Implications : in Econ. Geol. v105 pp 1361-1368
|
Sillitoe, R.H., Perello, J., Creaser, R.A., Wilton, J., Wilson, A.J. and Dawborn, T., 2017 - Age of the Zambian Copperbelt: in Mineralium Deposita v.52, pp. 1245-1268
|
Squire, R.J. and Keays, R.R., 2024 - The role of supermountain belts and climatic controls on the genesis of copper deposits in the Kupferschiefer and the Central African Copperbelt: in Mineralium Deposita v.59, pp. 717-732.
|
Sutton S J and Maynard J B, 2005 - A fluid mixing model for copper mineralization at Konkola North, Zambian Copperbelt: in J. of African Earth Sciences v42 pp 95-118
|
Sweeney M A, Binda P L 1989 - The Role of Diagenesis in the Formation of the Konkola Cu-Co Orebody of the Zambian Copperbelt: in Boyle R W, Brown A C, Jefferson C W, Jowett E C, Kirkham R V, (eds), Sediment-hosted Stratiform Copper Deposits Geological Association of Canada Special paper 36 pp 499-518
|
Sweeney M A, Binda P L 1994 - Some Constraints on the Formation of the Zambian Copperbelt Deposits: in J. of African Earth Sciences v19 pp 303-313
|
Sweeney M A, Binda P L, Vaughan D J 1991 - Genesis of the Ores of the Zambian Copperbelt: in Ore Geology Reviews v6 pp 51-76
|
Sweeney M, Turner P, Vaughan D J 1986 - Stable isotope and geochemical studies of the role of early diagenesis in ore formation, Konkola Basin, Zambian Copper belt: in Econ. Geol. v81 pp 1838-1852
|
Unrug R 1989 - LANDSAT-based Structural Map of the Lufilian Fold Belt and the Kundelungu Aulacogen, Shaba (Zaire), Zambia and Angola, and the Regional Position of Cu, Co, U, Au, Zn and Pb Mineralisation: in Boyle R W, Brown A C, Jefferson C W, Jowett E C, Kirkham R V, (eds), Sediment-hosted Stratiform Copper Deposits Geological Association of Canada Special paper 36 pp 519-524
|
Unrug R 1988 - Mineralization Controls and Source of Metals in the Lufilian Fold Belt, Shaba (Zaire), Zambia and Angola: in Econ. Geol. v83 pp 1247-1258
|
Unrug R 1988 - Mineralization controls and source of metals in the Lufilian Fold Belt, Shaba (Zaire), Zambia, and Angola: in Econ. Geol. v83 pp 1247-1258
|
van Eden J G 1974 - Deposition and Diagenetic Environment Related to Sulfide Mineralization, Mufulira, Zambia: in Econ. Geol. v69 pp 59-79
|
Wendorff M, 2005 - Sedimentary genesis and lithostratigraphy of Neoproterozoic megabreccia from Mufulira, Copperbelt of Zambia: in J. of African Earth Sciences v42 pp 61-81
|
Winfield O 1961 - Chibuluma: in Mendelsohn F (ed), Geology of the Northern Rhodesian Copperbelt McDonald, London pp 328-342
|
Zientek M L, Hayes T S and Hammarstrom J M 2013 - Overview of a New Descriptive Model for Sediment- Hosted Stratabound Copper Deposits: in Zientek M L, Hammarstrom J M and Johnson K M, 2013 Descriptive Models, Grade-Tonnage Relations, and Databases for the Assessment of Sediment-Hosted Copper Deposits - With Emphasis on Deposits in the Central African Copperbelt, Democratic Republic of the Congo and Zambia USGS Scientific Investigations, Report 2010-5090-J pp. 2-16
|
Porter GeoConsultancy Pty Ltd (PorterGeo) provides access to this database at no charge. It is largely based on scientific papers and reports in the public domain, and was current when the sources consulted were published. While PorterGeo endeavour to ensure the information was accurate at the time of compilation and subsequent updating, PorterGeo, its employees and servants: i). do not warrant, or make any representation regarding the use, or results of the use of the information contained herein as to its correctness, accuracy, currency, or otherwise; and ii). expressly disclaim all liability or responsibility to any person using the information or conclusions contained herein.
|
Top | Search Again | PGC Home | Terms & Conditions
|
|