Esquel - Galadriel-Julia Vein System |
|
Chubut, Argentina |
Main commodities:
Au Ag
|
|
 |
|
 |
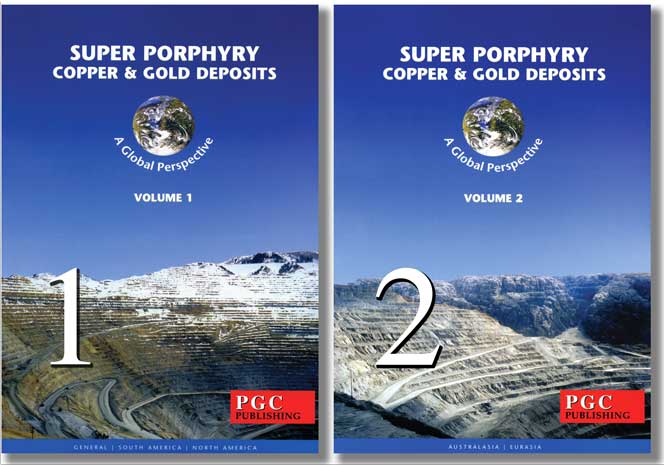 |
Super Porphyry Cu and Au

|
IOCG Deposits - 70 papers
|
All papers now Open Access.
Available as Full Text for direct download or on request. |
|
 |
The Esquel low sulphidation epithermal gold-silver vein deposit is located at the southeastern extremity of the Cordón de Esquel, a north-south trending range in the eastern foothills of the Patagonian Andes, ~12 km NE of the town of Esquel, Chubut Province, Argentina (#Location; 42° 53' 6"S, 71° 12' 40"W).
Several zones of spectacular limonitic staining are developed over barren pyritic rocks in the eastern part of the Esquel district, apparently unrelated the gold-bearing veins. These zones attracted several exploration companies, although the nearby vein outcrops were not apparently recognised until 1997. Subsequently, the exposed veins, which included the Galadriel vein containing the main resources, Minera El Desquite, a joint venture between London based Brancote Holdings plc and a group of private Argentinian investors, conducted an extensive reconnaissance geology and drainage geochemistry over the Cordón de Esquel. The program, which included BLEG (bulk leach extractable gold), panned concentrate and magnetic concentrate geochemical sampling, did not locate further veins. Chip sampling returned assays of up to 90 g/t Au from the exposed Galadriel vein, leading to drill testing of that vein, as well as the Galadriel Sur, Julia, Elena Norte, and Elena Sur vein from 1999 and from early 2000 to mid-2001. This testing defined a significant deposit in the Galadriel and Julia vein and smaller resources in the Elena Norte, Elena Sur, and Antonia veins. Step-out drilling below younger volcanic cover over the 800 m gap between the Galadriel and Julia vein located concealed major high grade vein connecting the two. The deposit was subsequently sold to Meridian Gold and then to Yamana Gold. Local opposition to mining has precluded it development.
Regional Setting
The deposit is hosted within a sequence of volcanic and volcano-sedimentary rocks that are the western equivalents of the continental scale Jurassic to Lower Cretaceous Chon Aike Large Igneous Province and are located in the overlap with the Andean Volcanic Arc. As such their composition is influenced by the arc magmatism and they have also been subjected to Late Cretaceous to Cenozoic deformation associated with the Andean Orogen. For detail of the setting and regional stratigraphy see the Southern Andes and Patagonia record.
Basement to the host sequence includes the moderately to intensely folded Permo-Carboniferous metasedimentary sequence of the Esquel Formation (Cuchi, 1980). The unconformably overlying Early Jurassic Piltriquitrón Formation, a fossiliferous shallow-marine sequence comprising organic-rich black shale and subordinate sandstone, conglomerate and limestone (Lizuaín, 1980), is interpreted to have been deposited in an extensional marine basin.
This sedimentary sequence is unconformably succeeded in the southern Cordón de Esque by subaerial andesitic and basaltic flows and pyroclastic and volcaniclastic rocks, along with lesser volumes of more felsic components, and have been subjected to lower greenschist-facies metamorphism. This volcanic sequence is no younger than earliest Late Jurassic. This volcanic suite may be compositionally and chronologically correlated with the mid-Jurassic Lago La Plata group found farther south in the Patagonian Andes (Haller and Lapido, 1980, 1982; Ramos, 1983) and with the broadly equivalent Lonco Trapial Group to the east (Nullo, 1983; Page and Page, 1993; Ramos and Aguirre-Urreta, 2000). Both of these volcanic units may constitute the eastern extremities of the Middle to Late Jurassic Ibáñez Formation, which is composed of an andesite- and basaltic andesite-dominated succession in eastern Chile (Baker et al., 1981). The Lago La Plata, Lonco Trapial, and Ibáñez sequences are all bimodal in composition, with the volumetrically subordinate anatectic rhyolitic rocks (Baker et al., 1981; Gust et al., 1985; Page and Page, 1993; Féraud et al., 1999; Riley et al., 2001).
Several small isolated plutons of equigranular granitoid cut the Jurassic rocks in the Cordón de Esquel, interpreted to belong to the eastern fringe of the tonalite- and granodiorite- dominated Patagonian batholith to the west, and were probably emplaced during the mid-Cretaceous (Ramos, 1983; Pankhurst et al., 1992).
All of these rocks are overlain by subaerial Tertiary volcanic and sedimentary rocks, with an Oligocene volcanic sequence capping the highest topography (Rapela et al., 1988).
The Esquel vein cluster is bounded to the south by the WNW striking Willimanco-Nahuel Pan fault system. This structure underwent multiple pulses of activity from at least the Jurassic onward, culminating in development of a prominent fault-controlled Neogene-Quaternary graben. This fault system is part of a prominent lineament that parallels the Ancud-Río Chubut lineament 70 km to the north. Both lineaments continue westward to the Pacific coast (Sillitoe et al., 2002).
Deposit Geology
The mineralised veins at Esquel are confined to a 5 x 2 km, north-south aligned corridor, hosted within a volcanic sequence, but thin markedly downwards to the underlying Early Jurassic organic-rich black shale unit. The host volcanic rocks are gently folded, subaerial two-pyroxene andesite and pyroxene- and olivine-bearing basaltic-andesite flows, with minor clastic and volcaniclastic sedimentary intercalations. One of these beds, a thin shale, locally contains silicified logs. A series of minor intrusions, mainly stocks, domes, sills and dykes cut the volcanic hosts. The various dykes and other intrusions follow the same fault system that controls veining. Porphyritic dacite stocks along the eastern margin of the veined corridor are pyritic and sericitised, and are believed to be related to the more widespread pyritisation that produced the colour anomalies mentioned previously. Garnetiferous rhyolite domes and related breccias occur immediately east and west of the vein corridor, and predate vein formation, apparently unrelated to the gold mineralisation. They are assumed to be peraluminous in composition, similar to other isolated Jurassic garnetiferous intrusive rocks elsewhere in Patagonia (e.g., Suárez et al., 1990). Other dykes include flow-banded biotite rhyolite along the eastern side of the zone of veining, as well as and andesite and basaltic-andesite in the remainder of the corridor, including a set closely associated with the Galadriel-Julia vein. The latter andesite and basaltic-andesite dykes both cut and are cut by the gold-bearing veins, implying they are syn-mineral. In contrast, the felsic dykes are all apparently pre-mineral, although they share the same structural control. The west-central sections of the vein corridor are capped by a gently dipping, ~1 km2 andesite porphyry sill that is up to 70 m thick, overlying rather than truncating the Galadriel-Julia vein. This sill steepens to the west to become a north-striking feeder dyke, and is unaltered, other than for zeolite (chabazite) veinlets, and is believed to be post-mineral.
Veining, Mineralisation and Alteration
The corridor of veining is bounded by sets of steep, north-south trending, 3 to 5 m thick veins, specifically the Antonia and Antonia Norte veins to the west and Ungoliant, Pinnacles and Isengard veins to the east. The major NNE striking composite Galadriel-Julia vein obliquely transects the corridor between these bounding faults/veins. It is ~2.4 km long, dipping at 50 to 70°W, and has a maximum thickness that ranges from 15 to 35 m, although fault repetition produces local composite thicknesses of as much as 70 m. The vein has a sigmoidal shape in both plan and section, and steepens and horsetails at depth. Sigmoidal subsidiary veins, up to 5 m thick are developed in the hanging wall and, to a lesser degree, the footwall of the main vein.
A set of east- to NE trending, sinistral-oblique slip transfer faults displace or terminate the Galadriel-Julia and corridor-bounding veins. The mineralised veins display changes in width, geometry, and gold content across and between these transfer faults, which do not appear to themselves host mineralised veins. The Esquel vein system comprises a suite of en echelon braided shears and tension gashes controlled by the combined sinistral strike-slip and normal-oblique displacements on the east-west and NE-striking transfer structures. The sigmoidal shape of the Galadriel-Julia vein segments is interpreted to be the product of the oblique motion during vein formation and transfer fault displacement, with maximum opening of each segment between transfer fault pairs (Soechting, 2001). These observations suggest the Galadriel-Julia vein initially formed as a NNE-SSW connecting structure between the two main north-south dextral faults defining the Esquel vein corridor, and was modified by later pre- or syn-mineral, NE-SW transforms that produced enhanced dilation zones within the Galadriel-Julia fault zone, and later partially dismembered the vein system.
The composite Galadriel-Julia vein comprises three discrete, gently plunging ore shoots or segments, each separated by a transfer fault where the vein thins. These shoots are, from north to south, the Galadriel, Galadriel Sur and Julia, which together form a near continuous mineralised body that is >2.4 km long. The largest individual ore shoot/segment, Julia, is 1.1 km long.
The three vein segments/ore shoots represent different degrees of erosion, reflecting differential displacement across the transfer faults. The relative depth of exposure tends to decrease from north to south. The Beleriand veins, to the north of the northern transfer zone, are the most deeply eroded and relatively low grade, with an exposure level near the top of the basal Early Jurassic sedimentary sequence.
The Galadriel-Julia ore shoots have limited vertical extents of only 120 to 200 m measured down the dip of the vein. These three ore shoots are characterised by sinuous, subhorizontal central cores, which are both wider and higher in grade than the marginal zones. Galadriel Sur is the highest grade of the three and comprises a 120 x 25 m central core averaging 24 g/t Au that in turn surrounds an inner 5 to 8 m thick nucleus of >60 to 100 g/t Au. Together, the three high grade ore shoots are estimated to contain 0.38 Mt @ 31.2 g/t Au.
Post-mineral faulting, partially following the same overall oblique-slip structural regime responsible for vein formation, disrupted the sigmoidal veining and with other faults was the site of late dyke emplacement. This faulting produced local imbrication of the Galadriel-Julia vein system and increases in ore-zone thickness. Competence contrasts within the vein corridor were sites of high- to low-angle shearing that generated pyrobitumen-rich gouge and fault breccia, probably at least some of which occurred during Mid- to Late Cretaceous and/or Tertiary tectonic inversions. Postmineral east-vergent thrusting and dextral strike-slip along vein margins suggests NE-directed compression. This tectonic activity is evident in the hanging and footwalls of the Galadriel-Julia vein, particularly on the outer edge of the massively silicified hanging wall, as well as along the top and bottom of the main sill. Local crush zones and development of stylolitic sutures were produced by late deformation of vein chalcedony.
Chalcedony and minor quartz are the main gangue minerals in the Galadriel-Julia ore shoots and the more restricted ore shoots of the north-south veining. The chalcedony is either white, dark grey or locally black, and appears to represent multiple stages of vein filling and brecciation, although much of the dark-grey chalcedony predates the white variety. In the Galadriel ore shoot, the dark-grey chalcedony is more abundant in the footwall side vein, whereas in the Galadriel Sur and Julia ore shoots, it is preferentially developed in the upper and hanging-wall sections. However, clasts of both dark-grey and white chalcedony are cut and cemented by later chalcedony phases to form heterolithic breccias that also commonly contain dark clasts of silicified andesite. Both types of chalcedony show local crustiform banding, cockade texture, replacement of lamellar carbonate by translucent quartz, and numerous small vugs lined with prismatic quartz crystals. Minor illite is found as isolated flakes and discontinuous bands in colloform chalcedony. A small quantity of barren, late-stage quartz is crystalline, some of it amethystine. Locally, coarsely crystalline calcite, a fibrous pale-brown carbonate, and traces of rhodochrosite cut, and locally brecciate, the vein chalcedony and quartz.
Adularia (KAlSi3O8) occurs as aggregated or disseminated euhedral crystals in thin (hydrocarbon-impregnated) dark quartz and chalcedony bands in colloform chalcedony. The crystals are commonly euhedral, subrhombic and up to 6 mm long. It has been weakly overprinted by argillic alteration and corrosion due to quartz replacement, and was an initial precipitate along the walls of section of the Galadriel-Julia vein, particularly in the immediate hanging wall of the vein, where it also occurs as coarse plumose aggregates in chalcedony veinlets. An unaltered and unweathered coarse-grained sample of this adularia yielded a K-Ar minimum age of 161±4 Ma (SERNAGEOMIN, Chile, unpub. report, 2001), suggesting the gold mineralisation is of late Middle to early Late Jurassic (Callovian-Oxfordian) age.
The quartz, amethyst and white chalcedony content of the veins apparently increases with depth below the ore shoots. Pods of delicately banded, multicolored chalcedony and quartz/agate are also locally characteristic of the deeper parts of the Galadriel ore shoot. The more deeply eroded sections of the Beleriand (north of the Galadriel shoot) and Isengard (northern of the eastern bounding structures) veins in the north of the corridor are typified by coarsely banded crystalline quartz with abundant prismatic quartz layers that are partly amethystine and by adularia, although crustification and carbonate-replacement textures are absent. Final stage calcite is more abundant in deeper veins.
Pervasive impregnation by probably 1 to 5 vol.% pyrobitumen is responsible for the dark-gray colouration and opacity of more than half of the chalcedony, although it is also macroscopically abundant throughout the veins, occurring as a black vitreous substance with a conchoidal fracture and black streak. This coarser pyrobitumen widely occurs as crustiform bands, cavity fillings up to 3 cm across, and globular aggregates with quartz. The latter are found as clusters of mm to cm scale ovoidal to spheroidal quartz shells variably lined with pyrobitumen and infilled with crystalline quartz within chalcedony.
Only minor sulphide minerals, probably <3 vol.%, occur within the vein chalcedony, mostly comprising pyrite and marcasite accompanied by abundant rutile. Minor acicular arsenopyrite, the third most abundant sulphide mineral, is only locally visible to the naked eye. Traces of fine-grained tetrahedrite-tennantite, galena, chalcopyrite and sphalerite are locally present, whereas pyrrhotite, bornite, bournonite, and freibergite (argentiferous tetrahedrite), along with a variety of silver, gold, bismuth, lead and mercury tellurides (particularly hessite: Ag2Te), are only visible in polished section and electron-microprobes.
Macroscopic sheaves of coarse-grained acicular stibnite have been observed in chalcedony at the very top of the Galadriel-Julia vein system, suggesting enhanced antimony may characterise the upward levels of at least some veins. Supergene oxidation has largely unaffected the vein sulphides, except in the uppermost few metres, although microscopic traces of chalcocite and covellite are presumed to be products of incipiently developed supergene enrichment.
Gold is found in chalcedony, sulphides and pyrobitumen as both native metal and as electrum of variable fineness. Native gold and electrum are rarely recognisable in vein outcrop and drill core. They typically occur as rounded, angular and irregularly shaped, <120 µm grains, most of which are <15 µm, and are intergrown with pyrobitumen. There is a good correlation between Ag and Au within veins, with Ag/Au ratios generally between 1 and 2, except in a few places where late-stage ruby silver (some determined to be pyrargyrite) is visible. The dark-gray chalcedony is commonly, but not consistently, richer in gold than the white variety.
Cu averages <250 ppm, Zn <150 ppm and Pb <150 ppm. Arsenic ranges from 150 to 1650 ppm and Sb from 40 to 200 ppm. The few reliable Hg analyses available are mostly <1 ppm.
Alteration typically occurs as selvages or haloes from tens of cm to tens of metres wide that tend to be proportional to the width of the enclosed vein, and is dominated by illite replacement of plagioclase. It grades outward from illite, through mixed-layer illite-smectite and smectite+chlorite assemblages to the pre-existing, district-wide, lower greenschist-facies metamorphic overprint that reached the zeolite and prehnite-pumpellyite facies (Vattuone et al., 2000). Locally, the immediately proximal alteration, particularly in the hanging wall of the Galadriel-Julia vein, comprises patchily developed, dark-grey to black pyrobitumen-impregnated massive silicification cut by multidirectional chalcedony veinlets. This style of alteration reaches a thickness of as much as 50 m in the hanging wall of the high-grade Galadriel Sur ore shoot, but thins dramatically upwards toward the vein termination. However, dark-grey silicification extends for a few meters, or locally as much as tens of metres above the tops of concealed veins. Pyrobitumen is frequently more abundant in the immediate altered wall rocks than in the veins. The vein-related silicification is fringed by an envelope of multidirectional calcite veinlets that extends several metres further outwards. Altered volcanic wall rocks are locally sulphidic, commonly containing more pyrite, marcasite and arsenopyrite than the vein material, as shown by the elevated As/Au and As/Ag ratios in the hanging- and footwalls of the Galadriel-Julia vein, especially in the latter.
Mineral Resources
Drilling to 2008 at Esquel had defined a total resource of 118 tonnes of gold and 217 t of silver, 89 percent of it in the measured and indicated categories (Pincock, Allen and Holt, unpub. report, 2001). Of this, 17.5 Mt @ 6.32 g/t Au for a total of 110 t of Au, is contained in the Galadriel-Julia vein. At a higher cutoff grade of 5 g/t Au, the Galadrial-Julia vein contains 7.75 Mt @ 10.87 g/t Au.
The information in this summary has largely been drawn from Sillitoe et al., 2002.
The most recent source geological information used to prepare this decription was dated: 2008.
This description is a summary from published sources, the chief of which are listed below. © Copyright Porter GeoConsultancy Pty Ltd. Unauthorised copying, reproduction, storage or dissemination prohibited.
Esquel
|
|
Blesa, A.P., 2003 - Geology and mineralization of the Esquel area, Patagonia, Argentina: in Colorado School of Mines, Golden, CO, USA., MSc Thesis, 211p.
|
Sillitoe, R.H., Cooper, C., Sale, M.J., Soechting, W., Echevarria, D. and Gallardo, J.L., 2002 - Discovery and Geology of the Esquel Low-Sulfidation Epithermal Gold Deposit, Patagonia, Argentina: in Society of Economic Geologists, Special Publication 9, pp. 227-240.
|
Soechting, W., Rubinstein, N. And Godeas, M., 2008 - Identification of ammonium-bearing minerals by shortwave infrared reflectance spectroscopy at the Esquel Gold Deposit, Argentina: in Econ. Geol. v.103, pp. 865-869.
|
Porter GeoConsultancy Pty Ltd (PorterGeo) provides access to this database at no charge. It is largely based on scientific papers and reports in the public domain, and was current when the sources consulted were published. While PorterGeo endeavour to ensure the information was accurate at the time of compilation and subsequent updating, PorterGeo, its employees and servants: i). do not warrant, or make any representation regarding the use, or results of the use of the information contained herein as to its correctness, accuracy, currency, or otherwise; and ii). expressly disclaim all liability or responsibility to any person using the information or conclusions contained herein.
|
Top | Search Again | PGC Home | Terms & Conditions
|
|