Bendigo |
|
Victoria, Vic, Australia |
Main commodities:
Au
|
|
 |
|
 |
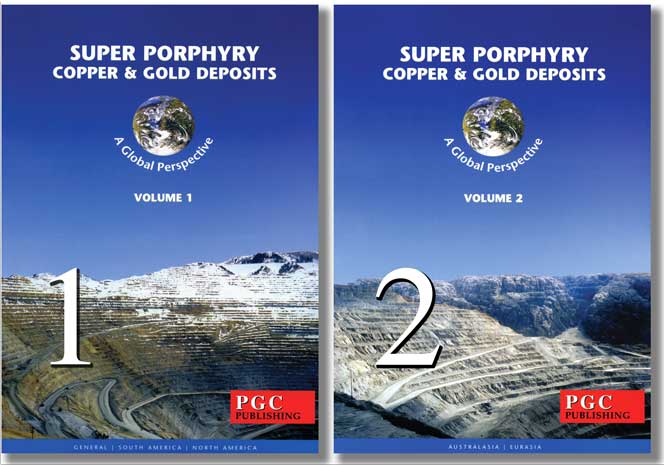 |
Super Porphyry Cu and Au

|
IOCG Deposits - 70 papers
|
All papers now Open Access.
Available as Full Text for direct download or on request. |
|
 |
The Bendigo Goldfield is located ~130 km NNW of Melbourne, in Victoria, south-eastern Australia (#Location: 36° 47' 41"S, 144° 15' 38"E [Bendigo Mining]; 36° 45' 53"S, 144° 16' 13"E [Central Deborah]).
Historically, the Bendigo Goldfield is the second largest gold producer in Australia after the Golden Mile at Kalgoorlie, with a cumulative output of 539.9 t of primary gold (Woodall, 1990) from an 9 x 5 km area. This was supplemented by another ~120 t of alluvial/deep lead gold. Of the 6380 t of gold produced in Australia up to 1987, some 2450 t originated from Victoria (Woodall, 1990), 60% of which was from alluvial/deep lead workings. By 2015, Australian production had climbed to 13 601 t of Au, little of which has come from Victoria.
Alluvial gold was discovered at Bendigo in October 1851 and by the end of the same year had yielded just over 6 tonnes of gold and the more than 15 tonnes in the following year. The first quartz reef mine at Specimen Hill on the Garden Gully Line, commenced operation in 1853, followed by mining on the Hustlers Reef in 1854 and the New Chum line in 1855. The annual production from the gold field exceeded 12 t per annum until 1859, reducing as the alluvial resources declined and quartz reef mining dominated. Annual output fluctuated between 7.5 and 5.5 t of Au, affected by the opening of new and the closure of older mines until the outbreak of World War 1 which brought a sharp decline to only 2 t in 1916. The downward trend continued with slight fluctuations, but by 1927 had reached a low of only 0.4 t of Au. From 1930 when the Deborah line became prominent, and the price of gold increased, production had increased to just over 2 t in 1941, but declined again until 1954 when the Central Deborah mine, the last significant operation, closed (Thomas, 1953). During its history, ~5000 exploratory and production shafts were sunk in the gold field, at least 140 of which were >300 m in depth, while 67 were >600 m, and 11 >1000 m deep.
A remaining inferred resource was estimated in 2006 to be 23.5 Mt @ 14.5 g/t Au for 340 t Au (Bendigo Mining, 2006). In 2018, underground exploration was concentrated on the Nell Gwynne Line (see figure below; GBM Gold Limited Annual Report, 2018).
Regional Setting
The Bendigo Goldfield lies within the Bendigo tectonic zone (Bendigo Zone) of the Palaeozoic Lachlan Fold Belt of eastern Australia, separated by major east vergent thrust faults from the Melbourne and Stawell zones to the east and west respectively. The core of the Bendigo Zone is interpreted to overlie Late Neoproterozoic to Cambrian oceanic crust separating Proterozoic basement below the two adjacent zones.
The Melbourne Zone overlies the interpreted Proterozoic Selwyn Block, which geophysical data indicates is a northerly extension of the Tyennan and Rocky Cape blocks in Tasmania, which collectively form what Cayley (2011) refers to as the VanDieland Microcontinent. The eastern margin of the Melbourne Zone and underlying Selwyn Block, is defined by the steeply east dipping, west vergent Governeor Fault, which separates them from the Tabberabberan Zone to the east. The Melbourne Zone, and the underlying VanDieland Microcontinent, both taper northward towards the Murray River, and are absent in southern central NSW where the Bendigo and Tabberabberan Zones are contiguous. The Stawell Zone is the western-most terrane of the Lachlan Fold Belt, and has a transitional western margin across the west vergent Moyston Fault with rocks of the late Lower Cambrian to Early Ordovician Delamerian Orogen. The latter include attenuated Proterozoic to Cambrian basement rocks.
Each of the key tectonic elements affecting the Bendigo Zone is discussed below.
The Stawell Zone is bounded by the Moyston and Avoca faults to the west and east respectively, with the latter dipping steeply west to intersect the former at a depth of ~22 km. Data from deep seismic traverses is interpreted to indicate the sequence above these faults is composed of Cambrian metaturbidite rocks of the 505 to 490 Ma Moornambool Metamorphic Complex, interlayered with lesser mafic volcanic rocks, and underlain by a thick imbricated suite of mafic volcanic rocks with interbedded sedimentary rocks, similar to those of the Glenelg Zone. The Stawell gold deposits occur toward the western margin of this zone.
The Bendigo Zone, lies between the west dipping Avoca and Heathcote thrust faults, and is composed of a thick imbricated pile of Cambrian to Ordovician (490 to 440 Ma) quartz-mica turbiditic rocks> These were deposited into a deep marine environment along the eastern edge of the Australian Craton, and are composed of detritus eroded from the exposed Delamerian orogen to the west. These rocks overlie a thicker suite of similarly imbricated Cambrian tholeiitic volcanic rocks. The latter, as interpreted from deep seismic reflection data, are underlain by Proterozoic rocks of the continental margin below the Moyston thrust fault on its western margin, and by similarly aged rocks of the Selwyn Block below the Heathcote thrust fault on its eastern margin. In the central section, the Cambrian mafic volcanic rocks are indicated to directly overlie the the Moho. The Selwyn Block, which geophysical data suggest is the northern tip of VanDieland, is unconformably overlain by sporadic accumulations of volcanic rocks, which include the Cambrian calc‐alkaline Licola and Jamieson Volcanics, that are equivalent to the Mount Read Volcanics in Tasmania. This Proterozoic terrane is interpreted to be an exotic microcontinent created during the break-up of Rodinia, and lies on the trailing edge of the section of Rodinia (probably North America) that separated from the Australian craton and migrated eastward in the late Neoproterozoic. By 450 Ma, the sequence that had been accumulating in the Bendigo Zone began to be deformed as the Selwyn Block moved westward, with the shortening accommodated by thrusting on the Moyston, Avoca, Heathcote and numerous other faults, and extensive imbrication of the sequence within the Stawell and Bendigo zones. As these two zones were elevated by deformation, they shed detritus eastward to form the condensed Ordovician to Devonian cover sequence of the Murrindindi Supergroup in the Melbourne Zone which is underlain by the Selwyn Block (Cayley et al., 2011). Deposition ceased during the Middle Devonian Tabberabberan deformation (Cayley et al., 2002).
Click here to expand
The Stawell and Bendigo Zones are both broadly composed of a lower crustal zone of interlayered and tectonically interleaved metavolcanic and metasedimentary rocks and an upper crustal zone of tightly folded metasedimentary rocks. The lower sequences in the Stawell Zone were accreted to the Australian craton during the Cambrian, with preserved oceanic crustal basement, whilst much of the Early Cambrian oceanic crust beneath the Bendigo Zone also remaining as a crustal-scale imbricate thrust stack. Deep seismic reflection data have shown that thin-skinned deformation typifies of the Melbourne Zone, whilst the Stawell and Bendigo Zones have a thick-skinned structural style (Cayley et al., 2011).
Compressional deformation across the eastern Delamerian and western Lachlan Orogen was generally progressive, based on the geometry of east-verging thrusts and isotopic dating, with more intensive activity represented by the following four orogenic events and six deformation stages (Cayley et al., 2011; Miller et al., 2005; Gray et al., 2003; Foster et al., 1998). Isotopic dating and stratigraphic evidence suggests that these periods of more intense activity are punctuated by protracted intervals of relative tectonic quiescence across the whole of the Stawell and Bendigo zones, e.g., between 490 and 455 Ma and from 420 and 390 Ma (Cayley et al., 2011):
• Delamerian (~515 to 490 Ma), which is manifested within the Kanmantoo Trough, Glenelg and Grampians-Stavely zones and the Stawell Corridor on the western margin of the Stawell Zone, but not in the remainder of the latter or the Bendigo and Melbourne zones. It is split into two parts, the first being the major D1a phase of crustal shortening, thickening and fault imbrication, mainly between 505 and 500 Ma, which ended a protracted period of extension and crustal attenuation, culminating in the inversion of the Adelaide Rift and Kanmantoo Trough sequences in the west (Preiss, 1995, 2000; Jenkins et al., 2002). D1a also led to the cessation of ~510 to 502 Ma Cambrian volcanism. It corresponded to a phase of sinistral transpression and the development of a series of thrust packages that overthrust the Grampians-Stavely Zone by rocks of both the adjacent Glenelg and Stawell zones. This was followed by the much less intense D1b, a phase of sinistral transtension that produced WNW trending extensional structures, and associated ~502 to 498 Ma, post-orogenic magmatism including intrusion of granites and porphyries (Cayley et al., 2018).
• Benambran (~455 to 440 Ma), which comprised a sequence of east-west shortening events, D2, that resulted in uplift and termination of deposition in the Bendigo Zone. It produced a pattern of tight, closely spaced, meridional folds with near-vertical hinges, and high-angle, east vergent, regularly spaced thrusts deforming the deep-marine Lower Palaeozoic turbiditic metasedimentary rocks of the Bendigo and Stawell zones. The Moyston Fault was subjected to major reactivation during D2. The Heathcote Fault Zone marks both the eastern margin of the Bendigo Zone, and the eastern limit of more intense Benambran deformation within the western Lachlan Orogen. Faulting within the Bendigo Zone is generally west dipping with throws of only a few kilometres. While important in the Bendigo and Stawell zones, this event was only of minor significance in the Glenelg and Grampians-Stavely zones. In contrast, the Melbourne Zone remained undeformed in a marine depositional setting throughout the Benambran Orogeny (Cayley et al., 2011).
• Bindian (~420 to 400 Ma), reflected by both D3 and D4, which are apparently absent from the Melbourne Zone, but are evident in the Tabberabberan Zone and further east, and in the Stawell Corridor of the western Stawell Zone, and in the Grampians-Stavely Zone. In the eastern Tabberabbera Zone and further east, widely developed, steep NE to east-west trending crenulation cleavages overprint Benambran foliation, considered to have formed from north-south shortening (Morand et al., 2005; Willman et al., 2005). The Moyston Fault underwent major reactivation during the Bindian orogenies (Cayley and Taylor 2001; Miller et al., 2006). Within the Grampians-Stavely Zone, D3 deformation took place between ~420 and 400 Ma and involved minor sinistral transpression producing low-angle to bedding-parallel faults and large open folds. In the same area, D4, at ~400 Ma, took the form of major dextral transpression, reworking of and drag folding of D1 to D3 structures, producing low-angle extensional, transtensional and strike-slip faults (Cayley et al., 2018).
• Tabberabberan (~390 Ma) or D5 orogenic events were most intense in the east, decreasing westward. During this event, the Tabberabbera Zone overthrust the eastern margin of the Selwyn Block and Melbourne Zone along the Governor Fault. The Melbourne Zone is characterised by widespread development of mainly north to NW trending upright, open to tight folds with increasing deformation eastwards, including multiple folding and foliation development, towards the Governor Fault (Gray, 1995; Murphy and Gray, 1992; VandenBerg et al., 1995, 2006). Within the Bendigo and Stawell zones, Tabberabberan deformation is restricted to a thrust fault in the Stawell gold mine, where NE–SW compression is indicated (Miller et al., 2006; Phillips et al., 2012), and reactivation of the Heathcote Fault Zone along the eastern margin of the Bendigo Zone (VandenBerg et al., 2000). Further west in the Grampians-Stavely Zone, minor sinistral transpression is indicated producing subvertical joint arrays and minor sinistral strike-slip reactivation of existing faults (Cayley et al., 2018).
The mineralised vein systems of the Bendigo-Ballarat region formed at ~445 Ma during regional deformation, followed by less widespread events at 410 and 370 Ma (Phillips et al., 2012).
During the Ordovician to Early Silurian (490 to 439 Ma), subduction was active along the intra-oceanic Macquarie Volcanic Arc to the east of the Australian craton and the Selwyn Block.
Geology and Mineralisation
The Bendigo goldfield encompasses Cambrian to Carboniferous age rocks developed under a compressional regime imposed upon a sequence of marine and non-marine sedimentation, igneous intrusion and regional deformation and metamorphism.
The earliest known rocks are Cambrian volcanics (oceanic crust emplaced during the breakup of Rodinia) and sediments, which are not locally exposed. These are overlain by the dominant lithologies exposed in the goldfield, namely Lower Ordovician marine sequence of turbiditic sandstones, siltstones and mudstones of the Castlemaine Supergroup, which is estimated to be in excess of 5 km in thickness. These turbidites are entirely derived from continental sources and comprise a mixture of quartz, mica, rutile, tourmaline and lithic fragments and contain graptolite assemblages.
During the Late Ordovician to Late Devonian the Bendigo-Ballarat zone underwent crustal shortening resulting in extensive folding and reverse faulting which have significantly increased the apparent thickness of the Castlemaine Supergroup. The Ordovician sediments have been intruded by the post-tectonic Upper Devonian Harcourt Granodiorite to the south of Bendigo, while Jurassic lamprophyre dykes also intruded the Ordovician sediments, with evidence of at least one diatreme eruption preserved in the area.
The Bendigo Goldfield lies in a 9 km wide, NNW trending block of monotonous, Lower Ordovician, lower greenschist facies turbidites, bounded by the regional, steeply west dipping Whitelaw and Sebastian thrust faults. These two faults have a strike-slip component and a displacement of at least 1500 m.
The dominant lithologies in the Bendigo area comprise a very uniform sequence of turbiditic sandstones, siltstones/slates and mudstones interbedded with hemipelagic mudstone and minor 'cone-in-cone' limestones. Deformation has only locally modified sedimentary structures, including graded bedding, which are perfectly preserved and provide evidence for the younging direction of beds. Graptolites, mainly within black mudstones, reveal the age of the sediments as the Lancefieldian to Castlemainian stages of the Lower Ordovician. The higher stratigraphic levels are characterised by more complex bed thickness variations often forming recognisable cycles 15 to 50 m thick. Sandstone beds are often grouped in 10 to 20 m thick packages which can be continuously traced along strikes lengths of up to several km, although rapid facies variations and lensing over short distances are also common.
The Lower Ordovician sediments of the Bendigo Goldfield are characterised by regular, close spaced folding with extensive local reverse faulting related to the compression event responsible for the folding. Within the goldfield, mineralisation occurs across at least 3.2 km of the stratigraphic succession.
The productive portion of the goldfield is contained within a 15 x 5 km zone, represented by a tightly folded synclinorium in the centre of the fault-bounded block. More than 20 near parallel anticlines have been mineralised within this zone, with 80% of the production being from quartz bodies in anticlinal crests. The anticlines strike at ~340° with axial planes dipping slightly to the east of vertical. The limbs of the anticlines typically dip at 65 to 75° to the east and west.
These folds have a regular frequency, are continuous along strike, and display a strong structural control on gold-quartz mineralisation. Wavelengths vary up to 300 m, with amplitudes of from 250 to 600 m. Individual folds have been continuously traced over a length of up to 25 km along strike and mining has traced individual anticlines to as deep as 1.6 km. Folds plunge at 5 to 10°S on the southern end of the field and 5 to 15°N on the northern end producing a broad domal structure in the centre of the goldfield. Local variations in fold plunge give rise to smaller dome and basin structures along the strike of individual anticlines. Chevron folding with interlimb angles of <40° are apparently restricted to the mineralised central portion of the goldfield with folding to the east and west being more open. Apart from the Whitelaw and Sebastian Faults, faulting is largely only responsible for small displacements, and have been classified into the following three styles, all of which may host quartz-gold mineralisation.
i). Bedding-plane faults - which are reverse dip-slip structures confined to single bedding planes, are characterised by 1 to 200 cm thick laminated quartz with associated black carbonaceous pug. Some of these banded veins, are not accompanied by pug and show only small displacements parallel to bedding.
ii). Break faults - which are reverse faults striking sub-parallel to bedding but truncate bedding in cross section. They form when bedding plane faults on one limb of a fold cross an anticlinal or synclinal axis and are then at a high angle to bedding on the adjacent limb until crossing the fold axis to be bedding parallel again. Where 'bedding plane faults' transition to 'break faults' their dip often flattens. During subsequent thrust displacement, they provide a locus of dilation. They have limited vertical continuity while extending along strike for several kilometres, and may be either east or west dipping. They repeat at regular intervals with depth and are the major structural control to mineralisation in the Bendigo Goldfield.
iii). Oblique (or cross) faults - which cut across the regional structural trend, and cross the reefs, displacing them horizontally.
Well-developed S1 cleavage formed during folding and is most strongly developed in fold hinges. In pelitic rocks the closely spaced (sub-millimetre scale) slaty cleavage is parallel to the fold axial surface, while in sandstones, a more widely spaced differentiated cleavage developed and is best represented at fold hinges as convergent fans. Cleavage lamellae are up to several centimetres apart, and are commonly refracted across sandstone-shales bedding contacts.
Known gold mineralisation is exclusively associated with quartz veining and occurs as:
• Free gold in quartz;
• In association with sulphides in quartz;
• In association with fragments or laminae of wall rock in quartz;
• Associated with sulphides in wall rock adjacent to quartz veins.
The mineralised quartz reefs are surrounded by a broad halo of weak hydrothermal alteration, comprising sericite, chlorite and carbonate with disseminated pyrite, while large crystals of arsenopyrite are commonly found within a few metres of the mineralised reef. Weak pervasive silicification is restricted to sandstones.
The veins range from massive and crystalline, locally containing vughs, to laminated, banded or 'crinkly' quartz, enclosing numerous angular blocks of sandstone and the slate. The laminations or banding is caused by the presence of a undigested relicts of black slate, whilst the 'crinkly' texture is caused by the presence of crenulated slate. In places and reefs are sheared and appreciated and re-cemented by quartz (Thomas, 1953).
The mineralised reefs are composed of 'bucky' or laminated quartz with small amounts of ankerite, sericite, calcite and rarely albite, as well as minor sulphides, mainly pyrite, arsenopyrite, pyrrhotite, chalcopyrite, sphalerite and galena, constituting between 0.5 and 2.5%. Arsenopyrite and pyrite tend to occur in the wall rocks and in slate inclusions whilst the other sulphides are chiefly found in the quartz veins. The sulphides contain a little fine-grained gold, whilst the presence of galena and sphalerite were regarded as favourable to the occurrence of gold. Approximately 5% of historic reef gold production has come from roasted sulphide concentrates. In places gold and sulphides are intimately intergrown. The bulk of the gold is free and visible, occurring as 100 µm to 2 mm grains, and occasionally as large nuggets. Many of the quartz reefs however, are barren (Thomas, 1953).
A feature of the Bendigo Goldfield is the irregular distribution of gold within the reefs. According to Thomas (1953), all of the reefs probably contain ~0.3 g/t Au, while many unpayable reefs carry ~1.5 g/t, and the levels of >5 g/t are only found locally. By contrast the shoots exploited contain as much as 100 g/t Au. Individual shoots vary from <100 to as much as 1.5 km in length, following the shallow flat to ~20° plunge of the mineralised folds.
Gold mineralisation was synchronous with the major period of regional compression during which the Ordovician sediments were detached from the underlying Cambrian oceanic crust. The resultant structural increase in crustal thickness of the Ordovician sequences is believed to have caused prograde regional metamorphism of the underlying Cambrian stratigraphy resulting in the formation and release of auriferous hydrothermal fluids. These fluids are postulated to have migrated up fault splays associated with major regional structures to be precipitated in nearby favourable low pressure dilation zones created when the hydraulic pressure of trapped fluids exceeded lithostatic pressure during local seismic events.
Two main styles of quartz are recognised, namely:
i). Laminated quartz which consists of multiple laminae or veinlets of quartz, separated by millimetre thick slivers of wall rock and/or sulphides typically developed in bedding parallel veins. The gold may be either fine or coarse grained, and is typically restricted to only one or a few laminae.
ii). Bucky quartz occurring as coarsely crystalline, non-laminated and sometimes vuggy quartz in non-concordant veins that cut across bedding. The gold, when present, is typically free and coarse grained (0.25 to 2.5 mm), except when associated with sulphides.
In addition, veins may contain a dolomitic carbonate (typically ankerite), albite and from 0.5 to 2.5% sulphides (pyrite, lesser arsenopyrite, galena, sphalerite, minor chalcopyrite and rare pyrrhotite).
Five broad mineralised quartz reefs have been recognised, as follow:
i). Saddle Reefs and Bedded Leg Reefs - saddle reefs, which occupy the dilational void of the fold hinge, usually have a small sectional area but are extensive along strike, forming in zones of high rock competency contrast. Bedding parallel legs consist of laminated quartz produced by multiple crack seal events.
ii). False Saddle and Neck Reefs - which are modified saddle reefs with extended necks either on one conjugate thrust fault, axial plane shear or bedding parallel 'leg' vein of the saddle continues upward beyond the saddle crest to form and inverted 'Y'. Alternatively, two bedding parallel veins may intersect to form a false saddle at an anticlinal crest.
iii). Fault Reefs - which are strike parallel fault mineralisation occurring as fissure veins, breccia, spurs and massive quartz developed in 'break' faults discordant to bedding. Mineralisation may occur away from the anticlinal zone depending on the penetrative power of the fault in the discordant limb. West or east dipping thrust faults also significantly displace the anticline and may produce false saddles.
iv). Spur Reefs - stockwork or spur veins occur in stratabound zones and in close proximity to bedded or discordant faults and reefs, and are best developed in brittle hosts where veining is often orientated normal to bedding and thins away from the plane of movement. Mineralisation of this style can be caused by a combination of bedding plane slip and flexure in a sandy medium.
v). Cross-course Reefs - mineralisation within oblique faults occurs as fissure veining, breccia, spurs, and massive quartz and may form long, steeply plunging shoots, extending well out into the limbs of the folds. Their steep plunge reflects the line of intersection of the bedding oblique fault with either a receptive lithological unit or bedding parallel structure. These are relatively rare in the Bendigo Goldfield and have been responsible for only a small percentage of the total gold production.
The most important reefs: i). occur in close proximity to an anticline axis; ii). display small cross sectional area and limited depth extent; iii). have one long dimension which is parallel to the crest of the associated anticline and iv). occur in groups or 'clusters' suggesting interrelated structural controls.
The principal mineralised lines of lode, from east to west, and the [mines] that each produced more than 3 tonnes of gold are:
• Hustlers - [United Hunters and Redan] - 4.09 t of Au; [Hustlers Reef] - 5.29 t of Au; [Great Extended Hustlers] - 9.33 t of Au;
• Derby - no significant mines;
• Paddy's Gully - no significant mines;
• Garden Gully - [New Moon] - 7.37 t of Au; [South New Moon] - 7.96 t of Au; [Virginia] - 4.72 t of Au; [Great Northern] - 4.18 t of Au; [Johnson's Reef] - 10.22 t of Au; [Koch's Pioneer] - 4.24 t of Au; [Carlisle] - 5.50 t of Au; [Garden Gully United] - 13.82 t of Au; [Great Southern] - 3.22 t of Au;
• Deborah - [North Deborah] - 3.76 t of Au;
• Sheepshead - [Hercules] - 3.36 t of Au; [New Red White and Blue Consolidated] - 8.60 t of Au;
• New Chum - [Catherinee Reef United] - 7.27 t of Au; [United Devonshire] - 3.14 t of Au; [Hercules and Energetic] - 3.84 t of Au; [Lansell's 180] - 3.47 t of Au; [Shenandoah] - 3.65 t of Au; [New Chum Railway] - 3.50 t of Au;
• Nell Gwynne and its southern splay British and Foreign - largest [Central Nell Gwynne] - 1.92 t Au;
• Napoleon and its northwestern splay Maiden's Gully - no significant mines;
• Lancashire (west of Napoleon in the southern part of the field) - no significant mines;
• Carshalton (west of Lancashire) - no significant mines;
• Thistle (west of Carshalton in the southern part of the field) - no significant mines;
• Christmas (west of Thistle and ~1000 m west of Napoleon and Maidens Gully) - no significant mines;.
This summary is largely based on a description on the Bendigo Mining Limited website
The most recent source geological information used to prepare this decription was dated: 2007.
This description is a summary from published sources, the chief of which are listed below. © Copyright Porter GeoConsultancy Pty Ltd. Unauthorised copying, reproduction, storage or dissemination prohibited.
Bendigo Mining Central Deborah
|
|
|
Bierlein F P and Maher S 2001 - Orogenic disseminated gold in phanerozoic fold belts - examples from Victoria, Australia and elsewhere : in Ore Geology Reviews v18 pp 113-148
|
Bierlein F P, Christie A B, Smith P K, 2004 - A comparison of orogenic gold mineralisation in central Victoria (AUS), western South Island (NZ) and Nova Scotia (CAN): implications for variations in the endowment of Palaeozoic metamorphic terrains: in Ore Geology Reviews v25 pp 125-168
|
Bierlein F P, Fuller T, Stuwe K, Arne D C and Keays R R 1998 - Wallrock alteration associated with turbidite-hosted gold deposits. Examples from the Palaeozoic Lachlan Fold Belt in central Victoria, Australia: in Ore Geology Reviews v13 pp 345-380
|
Boucher, R.K., Rossiter, A.G., Fraser R.M. and Turnbull, D.G., 2015 - Review of the structural architecture of turbidite-hosted gold deposits, Victoria, Australia: in Trans. IMM (incorp. AusIMM Proc.), Section B, Appl. Earth Sc. v.124, pp. 136-146.
|
Bull, S.W. and Large, R.R., 2014 - Setting the stage for the genesis of the giant Bendigo ore system: in Jenkin, G.R.T., Lusty, P.A.J., McDonald, I., Smith, M.P., Boyce, A.J. and Wilkinson, J.J., (eds.), 2014 Ore Deposits in an Evolving Earth, The Geological Society, London, Special Publications, 393, pp. 161-187.
|
Cayley, R.A., Korsch, R.J., Moore, D.H., Costelloe, R.D., Nakamura, A., Willman, C.E., Rawling, T.J., Morand, V.J., Skladzien, P.B. and O Shea, P.J., 2011 - Crustal architecture of central Victoria: results from the 2006 deep crustal reflection seismic survey: in Australian J. of Earth Sciences v.58, pp. 113-156.
|
Cox S F, Wall V J, Etheridge M A and Potter T F, 1991 - Deformational and metamorphic processes in the formation of mesothermal vein-hosted gold deposits - examples from the Lachlan Fold Belt in central Victoria, Australia : in Ore Geology Reviews v6 pp 391-423
|
Fergusson, C.L., 2017 - Mid to late Paleozoic shortening pulses in the Lachlan Orogen, southeastern Australia: a review: in Australian J. of Earth Sciences v.64, pp. 1-39.
|
Forde A and Bell T H 1994 - Late structural control of mesothermal vein-hosted gold deposits in central Victoria, Australia: Mineralization mechanisms and exploration potential : in Ore Geology Reviews v9 pp 33-59
|
Foster D A, Gray D R, Kwak T A P and Bucher M 1998 - Chronology and tectonic framework of turbidite-hosted gold deposits in the Western Lachlan Fold Belt, Victoria: 40Ar-39Ar results: in Ore Geology Reviews v13 pp 229-250
|
Goldberg I S, Abramson G Y, Haslam C O and Los V L, 2007 - Depletion and Enrichment Zones in the Bendigo Goldfield: A Possible Source of Gold and Implications for Exploration: in Econ. Geol. v102 pp 743-753
|
Hughes, M.J. and Phillips, G.N., 2015 - Mineralogical domains within gold provinces: in Trans. IMM (incorp. AusIMM Proc.), Section B, Appl. Earth Sc. v.124, pp. 191-204.
|
Jia Y, Kerrich R 2001 - Stable isotope ( O, H, S, C, and N) systematics of Quartz vein systems in the turbidite-hosted central and north Deborah Gold deposits of the Bendigo Gold Field, central Victoria, Australia: constraints on the origin of ore-forming fluids: in Econ. Geol. v96 pp 705-721
|
Jia Y, Li X, Kerrich R 2000 - A fluid inclusion study of Au-bearing Quartz vein systems in the central and north Deborah deposits of the Bendigo Gold field, central Victoria, Australia: in Econ. Geol. v95 pp 467-494
|
Kirkby, A.L., Musgrave, R.J., Czarnota, K., Doublier, M.P., Duan, J., Cayley, R.A. and Kyi, D., 2020 - Lithospheric architecture of a Phanerozoic orogen from magnetotellurics: AusLAMP in the Tasmanides, southeast Australia: in Tectonophysics v.793, 13p. doi.org/10.1016/j.tecto.2020.228560.
|
Large R R, Danyushevsky L, Hollit C, Maslennikov V, Meffre S, Gilbert S, Bull S, Scott R, Emsbo P, Thomas H, Singh R and Foster J, 2009 - Gold and Trace Element Zonation in Pyrite Using a Laser Imaging Technique: Implications for the Timing of Gold in Orogenic and Carlin-Style Sediment-Hosted Deposits: in Econ. Geol. v104 pp 635-668
|
Leader L D, Wilson C J L and Robinson J A, 2013 - Structural Constraints and Numerical Simulation of Strain Localization in the Bendigo Goldfield, Victoria, Australia : in Econ. Geol. v.108 pp. 279-307
|
Li X, Kwak T A P and Brown R W, 1998 - Wallrock alteration in the Bendigo gold ore field, Victoria, Australia: Uses in exploration: in Ore Geology Reviews v13 pp 381-406
|
Lisitsin, V.A. and Pitcairn, I.K., 2016 - Orogenic gold mineral systems of the Western Lachlan Orogen (Victoria) and the Hodgkinson Province (Queensland): Crustal metal sources and cryptic zones of regional fluid flow: in Ore Geology Reviews v.76, pp. 280-295.
|
McDermott G J, Quigley P W 1998 - Williams United gold deposit, Bendigo: in Berkman D A, Mackenzie D H (Ed.s), 1998 Geology of Australian & Papua New Guinean Mineral Deposits The AusIMM, Melbourne Mono 22 pp 511-516
|
Phillips G N and Hughes M J, 1996 - The geology and gold deposits of the Victorian gold province : in Ore Geology Reviews v11 pp 255-302
|
Phillips, G.N. and Hughes, M.J., 1998 - Victorian gold province: in Berkman D A, Mackenzie D H (Ed.s), 1998 Geology of Australian & Papua New Guinean Mineral Deposits The AusIMM, Melbourne Mono 22 pp. 495-506.
|
Ramsay W R H, Bierlein F P, Arne D C and VandenBerg A H M, 1998 - Turbidite-hosted gold deposits of Central Victoria, Australia: their regional setting, mineralising styles, and some genetic constraints : in Ore Geology Reviews v13 pp 131-151
|
Schaubs P M, Wilson C J L 2002 - The relative roles of folding and faulting in controlling Gold mineralization along the Deborah Anticline, Bendigo, Victoria, Australia: in Econ. Geol. v97 pp 351-370
|
Schofield, A., (Ed.), Bailey, A., Bastrakov, E., Cairns, C., Cayley, R., Duncan, R., Huston, D., Lewis, C., McAlpine, S., Schofield, A., Skladzien, P., Taylor, D. and Thomas, M., 2018 - Regional geology and mineral systems of the Stavely Arc, western Victoria: in Geoscience Australia and Geological Survey of Victoria, Geoscience Australia, Record 2018/02, 225p. dx.doi.org/10.11636/Record.2018.002.
|
Sharpe E N, MacGeehan P J 1990 - Bendigo Goldfield: in Hughes FE (Ed.), 1990 Geology of the Mineral Deposits of Australia & Papua New Guinea The AusIMM, Melbourne Mono 14, v2 pp 1287-1296
|
Sibson R H and Scott J 1998 - Stress/fault controls on the containment and release of overpressured fluids: Examples from gold-quartz vein systems in Juneau, Alaska; Victoria, Australia and Otago, New Zealand: in Ore Geology Reviews v13 pp 293-306
|
Thomas D E 1953 - The Bendigo Goldfield: in Edwards A B (Ed), Geology of Australian Ore Deposits, 5th Empire Min & Met Cong, Aust & NZ, 1953 AusIMM, Melb. v1 pp 1011-1027
|
Thomas HV, Large RR, Bull SW, Maslennikov V, Berry RF, Fraser R, Froud S and Moye R, 2011 - Pyrite and Pyrrhotite Textures and Composition in Sediments, Laminated Quartz Veins, and Reefs at Bendigo Gold Mine, Australia: Insights for Ore Genesis : in Econ. Geol. v106 pp 1-31
|
Turnbull D G, McDermott G J 1998 - Deborah line of reef gold deposits, Bendigo: in Berkman D A, Mackenzie D H (Ed.s), 1998 Geology of Australian & Papua New Guinean Mineral Deposits The AusIMM, Melbourne Mono 22 pp 521-526
|
Willman C E, 2007 - Regional structural controls of gold mineralisation, Bendigo and Castlemaine goldfields, Central Victoria, Australia : in Mineralium Deposita v42 pp 449-463
|
Willman, C.E., Korsch, R.J., Moore, D.H., Cayley, R.A., Lisitisin, V.A., Rawling, T.J., Mrand, V.J. and OShea, P.J., 2010 - Crustal-Scale Fluid Pathways and Source Rocks in the Victorian Gold Province, Australia: Insights from Deep Seismic Reflection Profiles: in Econ. Geol. v.105, pp. 895-915.
|
Wilson C J L, Schaubs P M and Leader L D, 2013 - Mineral Precipitation in the Quartz Reefs of the Bendigo Gold Deposit, Victoria, Australia : in Econ. Geol. v.108 pp. 259-278
|
Porter GeoConsultancy Pty Ltd (PorterGeo) provides access to this database at no charge. It is largely based on scientific papers and reports in the public domain, and was current when the sources consulted were published. While PorterGeo endeavour to ensure the information was accurate at the time of compilation and subsequent updating, PorterGeo, its employees and servants: i). do not warrant, or make any representation regarding the use, or results of the use of the information contained herein as to its correctness, accuracy, currency, or otherwise; and ii). expressly disclaim all liability or responsibility to any person using the information or conclusions contained herein.
|
Top | Search Again | PGC Home | Terms & Conditions
|
|