George Fisher, Hilton |
|
Queensland, Qld, Australia |
Main commodities:
Zn Pb Ag
|
|
 |
|
 |
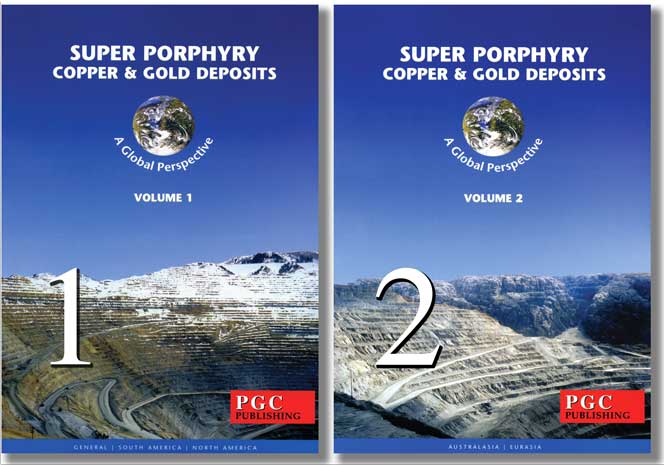 |
Super Porphyry Cu and Au

|
IOCG Deposits - 70 papers
|
All papers now Open Access.
Available as Full Text for direct download or on request. |
|
 |
The George Fisher South (previously Hilton or P49) and George Fisher North (L72) are located approximately 20 km north of the city of Mt Isa, in north-western Queensland, Australia and 975 km west of Townsville (#Location: George Fisher North - 20° 32' 56"S, 139° 28' 6"E; George Fisher South - 20° 34' 4"S, 139° 28' 35"E).
Whilst lead-zinc mineralisation had been discovered at what is now Mount Isa in March 1923 by prospector John Campbell Miles, and mining commenced in 1931, the Hilton deposit was not discovered until 1947, when S R Carter, a Mount Isa Mines (MIM) geologist, recognised outcropping cerussite at surface. Diamond drilling to test this discovery was commenced in August 1948, with the first drill hole intersecting a narrow interval of zinc mineralisation. From then until 1957, a significant follow-up drilling program was undertaken, and by 1950, the Hilton ore reserves stood at 26 Mt. The drilling program was curtailed in 1957 due to a fall in metal prices and heavy capital expenditure at the main Mount Isa operation. In 1966, MIM consolidated its mining lease holdings by taking up all the ground between Hilton and the Mount Isa operations within a single Special Mining Lease and diamond drilling recommenced at Hilton. The Hilton reserve was subsequently increased to 37 Mt. MIM decided to develop Hilton in 1969 but market factors delayed start-up of the 1 Mt/y mine and concentrator until 1990. In 1981, similar mineralisation was located 2 km further north at Hilton North, later to be renamed George Fisher North. Mining commenced at George Fisher in 2000.
Continental to Regional Setting
The George Fisher and Mount Isa orebodies lie within the Leichhardt River Fault Trough of the Western Fold Belt, part of the Mount Isa Inlier (or Domain) of Northwest Queensland. The Mount Isa Inlier comprises three major elements from west to east: the Western Fold Belt, the Kalkadoon-Leichhardt Belt and the Eastern Fold Belt, which are predominantly north-south trending sedimentological and structural domains (Blake and Stewart, 1992; O'Dea et al., 1997). The Western Fold belt is bounded to the east by 1870 to 1850 Ma felsic volcanic and coeval granitoid rocks of the Kalkadoon-Leichhardt Belt, interpreted to represent a remnant of a magmatic arc related to the Palaeoproterozoic Barramundi Orogeny. To the NW, the Western Fold belt is separated from the McArthur Basin in the Northern Territory by the 1853±4 Ma Murphy Metamorphics basement exposed as the narrow ENE-WSW trending Murphy Inlier.
The Western Fold belt is further divided into the narrow Leichhardt River Fault Trough immediately to the west of the Kalkadoon-Leichhardt Belt, and the broader Lawn Hill Platform further to the west, each separated from its neighbour by a major north-south trending terrane boundary fault zone (Blenkinsop et al., 2008; Foster and Austin, 2008). In the northern half of the belt, this structure is the major Mount Gordon Fault, while to the south it is in part the Mount Isa Fault.
The 1300 x 60 to 200 km Mount Isa Inlier-McArthur Basin succession comprise Late Palaeo- to Mesoproterozoic sequences that are, in turn, part of the more extensive 1800 to 1580 Ma Northern Australian Platform cover, a 5 to 15 km thick volcano-sedimentary succession draped across the northern third of the continent. Deposition within the Mt Isa-McArthur basin system took place in three super-basins which represent three nested cycles of deposition and exhumation, specifically the Leichhardt (1798 to 1738 Ma), Calvert (1728 to 1680 Ma) and Isa (1667 to 1575 Ma) super-basins, terminated by the 1590 to 1500 Ma Isan Orogeny, which was followed by the younger Roper super-basin.
All of the major stratabound Zn-Pb-Ag deposits of northern Australia, including the George Fisher and Mount Isa deposits, are hosted by the Isa Super-basin in the McArthur Basin (e.g., McArthur River), Lawn Hill Platform (e.g., Century and Lady Loretta), Leichhardt River Fault Trough (e.g., George Fisher, Mount Isa Zinc) and Eastern Fold Belt (e.g., Dugald River).
A ~25 to 30 m.y. hiatus marked the end of deposition and inversion of the Calvert superbasin within the Mount Isa inlier. During this period the Calvert Super-basin sedimentary rocks were uplifted and incised. Towards the end of this hiatus, the linear, north-south trending, voluminous, lopolithic 1671±8 Ma Sybella Granite, a foliated coarse porphyritic biotite granite, was intruded along the boundary between the Leichhardt River Trough and the Lawn Hill platform immediately to the west of Mount Isa. The exposed and sub-outcropping batholith occupies an area of ~220 x 15 to 30 km and defines the Sybella Domain of Withnall and Cranfield (2013). The northern extremity of this batholith passes into the NNE trending Mount Gordon Fault Zone which separates the Leichhardt River Trough from the Lawn Hill platform in the northern part of the Mt Isa Domain.
The depositional hiatus during Calvert super-basin inversion ended with renewed extension, development of the Isa Superbasin and recommencement of sedimentation from 1670 to 1590 Ma (Jackson et al., 2000). This superbasin is interpreted to have resulted from thermal subsidence of the lithosphere, the sag phase of Etheridge and Wall, 1994, and of Betts and Lister, 2001. This subsidence was most intense to the west and NW of the Leichhardt River Domain, where an extensive, thick blanket of carbonaceous shale, stromatolitic dolostone, and turbiditic sandstone and siltstone were deposited on the Lawn Hill platform the ~10 km thick McNamara Group (Krassay et al., 2000; Southgate et al., 2000). A similar sequence was deposited in the McArthur basin to the north, the 5.5 km thick McArthur Group, which hosts the McArthur River deposit in the Northern Territory (Rawlings, 1999). Within the narrower (40 to 90 km wide) Leichhardt River Trough, the Isa Superbasin sequence is thinner and and spans a narrower temporal range, represented by the up to 7.5 km thick, ~1670 to ~1647 Ma Mt Isa Group, which hosts the Mount Isa, George Fisher South (previously Hilton) and George Fisher North deposits close to its western margin, immediately adjacent to the regional-scale Mount Isa Fault. The Lady Loretta deposits are located within a similar sequence in the lower sections of the overlying McNamara Group on the eastern margin of the Lawn Hill platform. Century is located further to the north, also within the Lawn Hill Platform, adjacent to the major Termite Range Fault, which may merge with the Mount Isa Fault to the SE.
The regional structure of the Western Fold Belt of the Mount Isa Domain is dominated by faults and shear zones striking north-south (e.g. Mt Isa Fault), NW to NNW (e.g., Riversleigh and Termite Range faults) and NNE (e.g., Mount Gordon Fault), with subsidiary east-west, NW–SE or NE–SW structures.
Subsequent to the 1870 to 1850 Barramundi Orogeny, the rocks of the Mount Isa Domain were subjected to at least three phases of extensional faulting and rifting, and at least as many episodes of intervening inter- and post-rift compressional deformation and basin inversion spanning the three superbasin cycles. These events culminated in the most intense episode, the 1590 to 1500 Ma Isan Orogeny that terminated deposition of the Mount Isa Group (e.g. Blake, 1987; O'Dea et al., 1997; Betts et al., 2006; Gibson et al., 2012). Thrusting during inversion of the superbasins is interpreted, in many cases, to have reactivated listric faults active during the preceding extensional events.
The intensity of deformation and metamorphism varies markedly across the Mount Isa Inlier/Domain, although, in contrast to the more strongly deformed Eastern Fold Belt, it rarely exceeds greenschist facies in the rocks of the Lawn Hill Platform and Leichhardt River Fault Trough (Gibson et al., 2016). Metamorphism was largely low-pressure sub-greenschist facies during the Isan Orogeny (Jacques et al., 1982; Rubenach 1992; Oliver 1995; Rubenach and Barker 1998), although higher metamorphic grades to upper amphibolite facies are exposed in the hanging wall of the Mt Isa Fault, adjacent to the Sybella Batholith (Betts et al., 2006).
During deposition in the Leichhardt Superbasin, the Eastern Creek Volcanic pile and the thicker syn-rift sedimentary rocks, accumulated in an elongate, fault-bounded basin which, following deformation, is preserved as a 50 to 80 km wide belt (Bain et al., 1992; Blake, 1987; Derrick, 1982; Eriksson et al., 1983; Gibson et al., 2012; Jackson et al., 2000; Scott et al., 2000), largely restricted to the area of the Leichhardt River Fault Trough. This basin was bounded by faults typically striking NNW and dipping steeply to both the east and west, with displacements varying from a few hundred metres to several kilometres. Variations in this pattern indicate this is not a single basin but a series of highly asymmetric, overlapping, half-grabens, each oriented north-south and up to 70 to 130 km long, controlled by pre-existing fabric in the underlying basement (Gibson et al., 2016). Rifting ceased by no later than ~1740 Ma, with intrusion of post-kinematic granites and gabbro, whilst the regional north-south trending Leichhardt Anticline developed between 1740 and 1710 Ma within the Leichhardt River Fault Trough during east-west shortening inversion. Compression was followed by an episode of thermally induced subsidence (Gibson et al., 2016).
The western margin of the northern half of the Leichhardt River Fault Trough, separating it from the Lawn Hill Platform, is defined by the major, up to 7 km wide Mount Gordon Fault Zone, along which, the most recent activity has been dextral displacement of up to 10 km cutting all other fault sets. Towards its southern extremity, the Mount Gordon Fault curves north-south and then SSE to merge with and become part of the north-south Mt Isa fault corridor. Further south, the latter swings to the SSW again to become the Rufus and Mount Annabele fault zones.
The intensity of deformation within the Leichhardt River Fault Trough increases and is more complex in the vicinity of the Mt Isa Fault Zone where at least five deformation events have been interpreted based on overprinting relationships (Betts et al., 2006), including faulting and folding and overprinting of different strands within the zone (Connors and Lister 1995), three of which are indicated by post crystallisation dating of metamorphic minerals from the Sybella Granite at 1610±13, 1544±12 and 1510±13 Ma (Bell 1986). These deformational events are detailed in the 'Structure' and 'District Scale Structure and Metamorphism' sections below.
The Lawn Hill Platform differs from the Leichhardt River Fault Trough in that much of the Calvert and Isa superbasin successions are preserved in contrast to the latter, which is more deeply eroded, with only remnants of these sequences remaining. In the northern Lawn Hill Platform, immediately west of the Century deposit, a series of major NW-SE to NNW-SSE trending, east dipping faults occur over a width of ±20 km, including, from SW to NE, the Riversleigh, Little Range and Termite Range fault zones. These structures appear to have controlled deposition as listric faults bounding asymmetric, SW thickening half grabens. They also accommodated subsequent basin inversion as SW vergent thrusts, mainly during the Isan Orogeny. Depositional thickening and thrust displacement is most intense across the Riversleigh Fault zone, influencing the Leichhardt, Calvert and Isa Superbasin sequences. These structures approach, but do not appear to intersect the Mount Gordon-Mount Isa fault zones. Other NNW-SSE structures on the Lawn Hill Platform include the possibly sinistral May Downs Fault further to the south, which curves south to follow the western margin of the Sybella Granite just to the west of Mount Isa. To the east of these structures, over the platform, there appears to be little variation in thickness of the Calvert Superbasin sequence across other faults, suggesting little structural activity within the interior of the superbasin during deposition (Gibson et al., 2016), although ENE-WSW, mainly Calvert-age structures have been variously attributed to NW-SE crustal extension on the Lawn Hill Platform (Betts et al., 1998, Southgate et al, 2006).
At least two composite, regional Isan Orogeny basin inversion events are recorded in the Western Fold Belt, including:
i). ~north-south shortening and inversion of pre-existing east-west rift related structures between 1595 and 1570 Ma to produce east-west oriented axial-planar foliations in the immediate hangingwall of inverted extensional faults in the Leichhardt River Fault Trough and ENE-WSW structures in the Lawn Hill Platform (O'Dea and Lister 1995; O'Dea et al., 1997; Lister et al. 1999);
ii). a major phase of ~east-west shortening that developed crustal-scale north-south trending, shallowly plunging folds, and north-south trending reverse faults and thrusts across the Leichhardt River Fault Trough, and NE-trending folds throughout the Lawn Hill Platform between 1570 and 1550 Ma (Betts and Lister, 2002; Betts et al., 2004; Blaikie et al., 2017). Between ~1550 and 1540 Ma, the structural regime changed to strike-slip and oblique faulting and regional wrenching (O'Dea et al., 1997; Lister et al., 1999). This late east-west shortening was accommodated along NW trending sinistral, and NE trending dextral strike-slip faults. During inversion, zones of anomalous strain and buttressing occurred near normal faults and granitic plutons (Betts et al., 2006).
The Mount Isa terrane is considered to have been cratonised after the Isan Orogeny and the architecture of the region has not changed since the Late Mesoproterozoic.
It has been suggested that the Mount Isa Fault Zone is a Palaeoproterozoic Barramundi Orogen terrane-bounding suture (e.g., Hobbs et al., 2000). However, geophysical and geochemical data studied by Bierlein and Betts (2004) showed that basement rocks on either side of the Mount Isa Fault have similar densities, which is consistent with geochemical observations and Sm-Nd data that suggest basement lithologies on either side of the structure are geochemically and isotopically indistinguishable from each other, and that the Mount Isa Fault is unlikely to represent a suture zone that separates different Palaeoproterozoic terranes. Their data also indicates the crustal blocks on both sides must have been in close proximity of each other since the Palaeoproterozoic, and that the Western Fold Belt was part of the ancestral North Australian Craton well before the Barramundi Orogeny.
Regional to Deposit Scale Geology
The host sequence in the Mount Isa-George Fisher area is the Mount Isa Group, which unconformably overlies the Surprise Creek Formation of the Calvert Superbasin, and older rocks of the Leichhardt Superbasin, as follows:
Leichhardt Superbasin
• May Downs Gneiss - which is found ~5 km to the west of Mount Isa, between the overlying Mount Guide Quartzite and an intrusive contact with the 1671±8 Ma Sybella Granite to the west. It comprises plagioclase (microcline)-quartz gneiss and schist containing minor biotite, sillimanite and muscovite. It is reported to be younger than 1789±4 Ma and may be a more intensely metamorphosed equivalent of the Bottletree Formation (Australian Stratigraphic Units Database, viewed April, 2019).
• Bottletree Formation - up to 3000 m of porphyritic, rhyolitic to dacitic lava flows and ash-flow deposits, with interlayered greywacke and greywacke conglomerate, meta-arenite, quartzite, epidotic quartzite and grit. Sheared, schistose, amygdaloidal to massive metabasalt is common at or near the base and top of the formation. It unconformably overlies granites and volcanics rocks of the Kalkadoon-Leichhardt Belt, and is conformably followed by the Mount Guide Quartzite, the basal unit of the Haslingden Group;
• Mount Guide (and Leander) Quartzite - of the Guide Supersequence which comprises a >4000 m package of alluvial sheet, braided river and marginal lacustrine and marine deposits composed of a lower sequence of mainly greywacke type metasediments, including conglomerate and micaceous quartzite, and an upper suite of mostly ridge-forming quartz-rich sandstone metamorphosed to quartzite (Blake, 1987).
Deposition of the Bottletree Formation and Guide Supersequence was in an east-west extensional regime. The overlying sequences were deposited in a north-south directed extensional environment (Blaikie et al., 2017).
• Eastern Creek Volcanics - a 1790 to 1740 Ma, 6 to 15 km thick tholeiitic continental volcanic suite, principally composed of amygdaloidal to massive metabasalt, with interbedded clastic sedimentary rocks, deposited in an extensional setting. It has been subdivided into the Cromwell Metabasalt, Lena Quartzite and overlying Pickwick Metabasalt. These volcanic rocks were extruded under subaerial or shallow water conditions and are part of a larger bimodal igneous province represented in the Eastern Fold Belt by mafic and felsic volcanic rocks, including 1780 Ma rhyolites and ignimbrites of the Argylla Volcanics and the 1760 Ma Bulonga Volcanics (Neumann et al., 2009; Withnall and Hutton, 2013; Gibson et al., 2016);
• Myally Subgroup - formerly known as the Judenan Beds. This sequence constitutes the Myally Supersequence and is an up to 4000 m thick succession, from base to top, principally of quartzite, feldspathic quartzite to siltstone with associated pebbly to conglomeratic sandstone, mudstone, arkose, shale, quartzite, schist, dolomitic sandstone, oolitic and stromatolitic dolostone. Some felsic tuff occurs at the top, and metabasalt near the base of the sequence (Blake, 1987). This unit is the uppermost member of the Haslingden Group;
• Quilalar Formation - which is largely only exposed on the eastern margin of the Leichhardt River Fault Trough, distal to the Mount Isa area. It constitutes the Quilalar Supersequence and represents a transition to ~1750 to 1740 Ma post-rift sedimentation characterised by storm-, tide- and wave-dominated marine shelf and continental facies quartzite composed of feldspathic quartzite, orthoquartzite, conglomerate, arkosic grit, shale, siltstone, minor limestone and dolostone.
Calvert Superbasin
Bigie Supersequence
• Bigie Formation, a dominantly fluvial succession in a NW-SE directed extensional basin setting that formed as a SE thickening half graben between ~1710 and 1690 Ma. The sequence comprises up to 800 m of purple-brown hematitic sandstone, pebbly sandstone, tuff, conglomerate and red-brown siltstone.
• Fiery Creek Volcanics - a suite of bimodal volcanic rocks dated at 1708±2 Ma, comprising up to 750 m of rhyolite, agglomerate and amygdaloidal/vesicular altered basalt, interbedded with sandstone, conglomerate and siltstone.
Prize Supersequence
• Surprise Creek Formation - a 2500 m thick, generally upward fining sequence of alternating sandstone and quartzite sheets and wedge shaped siltstone horizons.
Isa Superbasin
Gun Supersequence - which is separated from the top of the Prize Supersequence by an unconformity and emplacement of the 1671±8 Ma Sybella Granite and 1678±2 Ma Carters Bore Rhyolite. It comprises, from the base to top:
Mount Isa Group which constitutes the Gun Supersequence in the Leichhardt River Fault Trough, and the lower sections of the Loretta Supersequence on the Lawn Hill Platform.
• Warrina Park Quartzite - a thin, discontinuous basal unit resting unconformably on rocks of the Calvert and Leichhardt superbasins. It comprises an up to 300 m thick, white conglomeratic orthoquartzite to blocky feldspathic sandstone with abundant ripple marks and cross-bedding;
• Mondarra Siltstone - which is generally 300 to 1000 m thick with a maximum of 1700 m. It is a thinly laminated, weakly micaceous, dolomitic siltstone with fine sandstone and black dolostone, overlain by dolomitic sandstone and micaceous slate.
• Breakaway Shale - up to 300 m of thinly laminated, siliceous siltstone/shale, locally chert, typically bleached at surface, but carbonaceous at depth. It is principally composed of quartz, albite, chlorite and muscovite with accessory carbon, microcline pyrite and tourmaline.
• Native Bee Siltstone - a carbonaceous, dolomitic shale and siltstone sequence up to 800 m thick, with rare, thin, vitrophyric tuff bands. Two main fancies have been recognised: i). a featureless carbonaceous shale; and ii). a rock type consisting of alternating white calcite and dark carbonaceous quartz-dolostone layers about 2 to 20 mm thick known locally as the 'zebra-shale'. It contains stromatolites, halite pseudomorphs, flat pebble conglomerates and cross-bedded channel deposits.
• Urquhart Shale - which has been dated at ~1655 Ma and has a maximum thickness of 1050 m. Where unmineralised, it comprises a carbonaceous, dolomitic shale and siltstone, closely resembling the Native Bee Siltstone. It contains numerous potassium rich marker beds, some of which have been positively identified as tuffs. Five facies have been recognised within the unit at George Fisher, each intercalated as bands ranging from a few mm to 25 m in thickness (after Chapman, 2004):
- Medium-bedded stylolitic mudstone - occurring as units with sharp boundaries that range from 5 to 15 m in thickness, composed of individual planar, laminated to massive beds that are 5 to 10 cm thick. This facies occurs as sheet-like bodies with consistent widths across the deposit. The mineralogy comprises quartz, calcite, and/or ferroan dolomite with accessory white mica, K feldspar and carbonaceous matter;
- Banded mudstone - occurring as units that range from 2 to 4 m in thickness with gradational boundaries. It is composed of discontinuous 10 to 100 m long lenses to sheets that extend across the deposit. Beds are more commonly massive than laminated or graded, and are 2 to 5 cm thick. The mineralogy is the same as for the medium-bedded stylolitic mudstone. White, layer parallel carbonate bands are common.
- Carbonaceous Siltstone - occurring as units with gradational boundaries that range from 2 to 50 cm in thickness, composed of mm to sub-millimetre scale alternating light grey and dark wavy laminations with intermittent millimetre thick planar laminations. The light grey laminations are predominantly composed of ferroan dolomite and quartz. The dark laminations have a similar composition, but in addition, contain abundant carbonaceous material. These bands are interleaved with banded mudstones.
- Pyritic Siltstone - occurring as units with gradational boundaries that range from 1 to 5 m in thickness, composed of mm to sub-millimetre scale alternating light grey and dark wavy laminations with intermittent millimetre thick planar laminations. The thickest intervals occur as sheets that are continuous across the deposit. However, at gradational boundaries with banded mudstone, individual laminations may be discontinuous. The light laminations have the same composition as those of the carbonaceous siltstone, while the darker laminations are composed of fine grained spheroidal pyrite and minor carbonaceous material. White, layer parallel carbonate bands and nodular carbonate are common to abundant. Pyritic Siltstone beds tend to be interbedded with banded mudstone to form units from a few to >100 m in thickness and constitute >80% of the central and upper sections of the Urquhart Shale.
- Tuffaceous Marker Beds - occurring as 0.5 to 10 cm thick beds that are continuous across the deposit. They have sharp contacts and are composed of quartz and K feldspar with accessory chlorite and white mica.
A different suite of constituent facies of the Urquhart Shale have been mapped at Mount Isa, although the variations are largely nomenclatural.
• Spear Siltstone - composed of rhythmically laminated siliceous dolomitic siltstone and siliceous dolostone up to 160 m thick. It contains stromatolites, halite pseudomorphs, flat pebble conglomerates and cross-bedded channel deposits.
• Kennedy Siltstone - which is very similar to the underlying Spear siltstone, differing only in its course bedded to massive character over much of the sequence which has a maximum thickness on 320 m. Sedimentary brecciation is common to both units.
• Magazine Shale - a carbonaceous siliceous shale unit that is the uppermost formation of the Mount Isa Group. It's upper section, along its entire strike length, is truncated by the Paroo Fault, with a maximum preserved thickness of 220 m. Outcrops have a characteristic reddish colour due to contained iron oxides.
Structure
A succession of deformation events has been recognised within the Leichhardt River Fault Trough, best reflected in the vicinity of the Mount Isa Fault, although not all are evident in the broader Western Fold Belt. These are as follows:
• D1, as defined by Bell (1983) is a pre- to early Isan Orogeny subhorizontal, north-south shortening that occurred between ~1610 and 1590 Ma. It produced local thrusting, east-west trending folds, variably developed bedding-parallel carbonaceous seams (S1), and a north-south trending mineral lineation at the Mount Isa mine (Bell, 1991), but is not recognised at the George Fisher deposit (Chapman, 2004). It is generally characterised by east-west trending, near vertical foliation parallel to bedding, with a north-south trending mineral elongation (Bell and Hickey, 1998).
• D2 which produced the bulk of the regional folds with steeply dipping north-south trending axial planes. It was caused by a east-west shortening event during the Isan Orogeny at ~1544 Ma (Page and Bell, 1986), which at George Fisher was responsible for upright, tight folds with steeply dipping, subvertical, north-south striking axial planes and an associated S2 foliation. It is also defined by concentrations of carbonaceous material. It accompanied folding of and reverse displacement on the Paroo and Hanging Wall faults and dyke intrusion (Chapman, 2004). S2 is the dominant regional schistosity, commonly with a steeply south pitching L2/2 stretching lineation (Bell and Hickey, 1998). The Mount Isa and George Fisher deposits are located on the western limb of a regional D2 anticline (Davis, 2004).
• D3, previously known as D2.5 of Bell and Hickey 1996; Perkins 1996; Bell and Hickey 1998; Chapman 2004 and others. This deformation was first recognised after D2 and D3 had been established in the literature, and hence was designated as D2.5 by Bell and Hickey (1996). However, subsequent authors (e.g., Davis, 2004) referred to it as D3 and then referred to the the subsequent D3 event as D4, etc. (c.f., Wilde et al., 2006; Long, 2010). This deformation, which is evident at both Mount Isa and George Fisher, was the result of NE-SW to ENE-WSW compression (McLellan et al., 2014). It rotated D2 structures (specifically S2) into gently dipping microfolds (or crenulations) to macroscopic folds with subhorizontal axial planes and east vergence, but no recorded foliation. These folds may either die out along their axial planes into mineralised layers, or curve to merge into S3 (Perkins, 1996).
• D4 of Davis (2004) and subsequent authors, (previously D3 of earlier authors as listed above), which is another shortening event, but reflects SE-NW shortening and took place at ~1510 Ma. It resulted in further development of folds with steeply dipping, subvertical axial planes and gently plunging axes that trend NW-SE to NNW-SSE and have an axial planar schistosity, S4 (Page and Bell, 1986; O'Dea et al., 1997; Bell and Hickey, 1998). These folds are more localised than those of D2. The largest D4 structure in the Mount Isa deposit area is the Mount Isa Fold, a syncline-anticline pair with a wavelength of 200 to 400 m, amplitude of 130 m, and a near vertical common short limb. Other D4 folds have wavelengths no wider than 10 to 20 m.
• Post D4, described by Chapman (2004) as Post D3, which is interpreted to have produced local NW-SE trending folds; brittle NNW-SSE splays from the Mount Isa-Paroo faults, including the Transmitter and Gidyea Creek faults between George Fisher and Mount Isa; and reactivation of the Mount Isa and Paroo fault zone. This deformation is assumed to encompasses the D5 and/or D6 listed below, but may well represent the late stages of D4.
• D5, which was previously described by Bell and Hickey (1998) as D4, is only manifested in the Mount Isa District as flat lying kinks, mainly to the west of the Mount Isa Fault in more schistose rock types. These kinks may be distinguished from D3 crenulations in that the latter have not been refolded by D4. The sense of shearing is generally east-vergent (Bell and Hickey, 1998).
• D6, is only very weakly and sparsely developed, forming crenulations or poorly developed crenulation cleavage. Where observed to the west of the Mount Isa Fault, it is associated with local retrogression. While not significant in the Western Fold Belt, it is more important in the Eastern Succession, being related to gold mineralisation at Starra (Bell and Hickey, 1998).
District Scale Structure and Metamorphism
In the Mount Isa-George Fisher area, the Mount Isa Group rocks occurs as a west-dipping, north-south striking sequence on the western limb of a regional
D2 anticline. The stratigraphic package is ~4000 m thick, decreasing north of the Transmitter fault (~2 km south of Hilton) where sections of the group have been removed by faulting (Valenta, 1994). The formations of the Upper Mount Isa Group are truncated to the west by the Paroo and Mount Isa faults and are locally fault-bounded against the lower Mount Isa Group to the east across the Barkly shear zone. The latter structure roughly separates the Urquhart Shales and Native Bee Siltstone.
The Paroo fault is interpreted to have originally formed as a listric extensional fault during deposition of the Kennedy Siltstone and Magazine Shale (Long, 2010), but may be a reactivated predecessor structure that bounded a half graben during Leichhardt Superbasin deposition (Lister, 2002; Betts et al., 2003). The related Mount Isa Fault was active by D2. Whilst both the Paroo and main Mount Isa faults were reactivated by brittle deformation during D2 and D3 to D4, Long (2010) and McLellan et al. (2014) propose that the Paroo Fault was folded during these deformations, while also undergoing reverse shearing. Long (2010) suggests this folding was influenced by uplift of the Sybella batholith which acted as a buttress during D2 shortening (Betts and Lister, 2002). The main Mount Isa and Paroo Faults are broadly parallel at surface to the west of the Mount Isa deposit, northward to beyond George Fisher. Over this interval, the Paroo Fault is in the footwall, and immediately east, of the steeply west dipping Mount Isa Fault. Down dip at Mount Isa however, the Paroo Fault diverges eastward from the Mount Isa Fault where it takes the form of gently north plunging, fault dislocated synform and then an antiform, before dipping steeply east to depth. As a result, the 60 to 65°W dipping Mount Isa Group was truncated at depth by the flat lying section of the folded Paroo Fault and juxtaposed with the underlying similarly steeply dipping Eastern Creek Volcanics and Guide Quartzite at 1655±4 Ma (Southgate et al., 2000; Valenta, 1994; Perkins et al., 1999). To the south of the Mount Isa deposit, this fold is evident where it intersects the surface, and the Paroo Fault diverges sharply from the main Mount Isa Fault juxtaposing the same two sequences. Brittle deformation related to reactivation of these two faults is the major manifestation of D4 brittle deformation in deposits of the Mount Isa district (Chapman, 2004). D2 and D3 shearing and reverse displacement along the folded Paroo Fault is regarded as being important in producing zones of dilation exploited by mineralising fluids (Long, 2010).
Peak metamorphism apparently occurred between D2 and D3 to the west of the Mount Isa and Paroo faults, where metamorphic grades are significantly higher, increasing from biotite to sillimanite-K feldspar grade westward toward the Sybella batholith (Connors and Page, 1995). Metamorphic temperatures within the Mount Isa Group declined northward from ~350 to 300°C at Mount Isa (Rubenach, 1992) to ~200°C at George Fisher (Chapman,1999, 2001). The age of the peak of metamorphism has been estimated at 1550 to 1570 Ma (SHRIMP U-Pb of zircons in folded pegmatite - 1554 ± 10 Ma; Connors and Page, 1995; chemical Th-U-total Pb isochron method or CHIME of monazite - 1570 Ma with no errors quoted; Hand and Rubatto, 2002; and Pb-Pb step-leach dating of tourmaline from quartz-tourmaline veins -1528±51 and 1577±48 Ma; Duncan et al., 2006).
Deposit Scale Structure
At George Fisher North, at the deposit and ore lens scale, mudstone and siltstone units generally dip at 30 to 80° west, with small domains extending for ~50 to 100 m down-dip characterised by near-vertical to slightly overturned bedding. These and other observations are interpreted to imply the presence of open folds with both steep and gently dipping axial planes (Chapman, 2004).
Small, centimetre to tens of centimetre scale tight to open folds are evident in some of the pyritic siltstone units, particularly where these units are mineralised with sphalerite and galena. These small scale folds have a wide range of fold profiles and orientations, with refolded folds and several cleavages evident. The distribution and geometry of these folds have no obvious parasitic relationship to the larger scale open folds detailed above and contrast to the more simple structure of the deposit at the larger scale. Also, in contrast to the Zn-Pb mineralised pyritic units, the largely barren mudstone beds lack similar small scale structures (Chapman, 2004).
At the mineralised unit scale, Chapman (2004) recognises four foliations at George Fisher, denoted as GFS1 to 4 inclusive. The earliest observed cleavage is usually the bedding-parallel GFS1, characterised by anastomosing penetrative seams of carbonaceous material in mudstones and a slaty cleavage in pyritic siltstones. The earliest folds are tight to isoclinal, east-vergent and north-south striking with 0.5 to 2 m amplitudes, and an axial plane cleavage, GFS2, developed in their hinges. These structures are refolded by younger GFF3 recumbent, and upright GFF4 folds with tight to isoclinal profiles around north-striking axes. These structures commonly produce complex interference patterns at 0.5 to 2 m scales. Chapman (2004) noted that it was not clear whether these small scale folds and multiple GFS1 to GFS4 cleavages were developed during a single continuous deformation or as successive, discrete events comparable to the regional D1 to D4.
Mineralisation
The George Fisher mines are some 20 km to the north along strike from the Mt Isa orebodies, and are located immediately to the east of the major, north-south trending Mt Isa fault system. Both George Fisher South (Hilton) and North are hosted by similar facies in the upper sections of the same host Urquhart Shale which also embrace the Mt Isa Zn-Pb ores, although the orebodies are generally thinner and more disrupted by faulting than the similar ores at Mt Isa. Little copper is known in association with the zinc lead ores at both Hilton and George Fisher.
Economic grade mineralisation at George Fisher occurs within a north-south striking, west dipping stratigraphic package that is 350 m thick, has a strike length of 1200 m, and extends down dip for >1000 m. It is obliquely truncated by the NNW-SSE trending Spring Creek and Gidyea Creek faults to the north and south respectively, and to the west at depth by the Hanging Wall Fault.
The George Fisher North deposit comprises 11 mineralised stratigraphic intervals that occur as stacked stratabound lenses, hosted by the Pyritic Siltstone and interbedded Pyritic Siltstone and Banded Mudstone units of the Urquhart Shale as described above. These units are separated by thicker bands of barren Banded Mudstone.
Mineralised intervals are denoted, from the stratigraphic base upward, as 1, 2 and A to I inclusive, with internal subdivisions denoted by a numerical subscript. The C and D orebodies contain almost half of the known George Fisher resource at grades of around 10.7% Zn, 5.9% Pb and 111g/t Ag. Each of the mineralised interval has distinctive bedding characteristics and tuffaceous marker beds, enabling detailed stratigraphic correlations throughout the ore-bearing
sequence (Johnston et al., 1998, Tolman et al., 2002).
Each of these mineralised intervals is segmented along strike by NE-SW to NNE-SSW striking faults which Chapman (2004) sugget are post-D4, but may be late D4. These faults contain pods of coarse calcite ±dolomite ±quartz ±sphalerite ±fluorite ±pyrite, localised in jogs, linked by graphitic fault gouge.
The George Fisher South (Hilton) deposit has 7 to 10 stacked ore lenses within a 250 m stratigraphic interval, which have been complicated by intense shortening associated with the Isan Orogeny. The two deposits are separated by a 2 km strike length of intensely faulted, barren shale, mudstone and siltstone.
These Pyritic Siltstone and interbedded Pyritic Siltstone and Banded Mudstone units carry 10 to >50% fine-grained diagenetic pyrite, distributed over a thickness of 800 m, enveloping both the George Fisher North and South deposits and extending over a strike length of >10 km.
The George Fisher North deposit has undergone a long and complex history of hydrothermal activity. The the earliest base metal sulphide-bearing assemblages of the deposit were preceded by four distinct gangue-forming events that included:
i). early dolomite, ankerite and ferroan dolomite cement replacing primary sedimentary grains and rock matrix, such that the rock is enriched in Fe and Mn relative to background Urquhart Shale;
ii). nodular and banded calcite that predates stylolite formation, manifested as as pervasive bleaching of barren mudstone while nodular calcite is rhythmically interwoven with the fine-grained diagenetic pyrite in finely laminated siltstone and finely banded mudstone;
iii). widely developed, fine-grained pyrite precipitated within some siltstone units and along carbonaceous stylolite surfaces;
iv). development of celsian, hyalophane, K feldspar, calcite ±ferroan dolomite ±quartz veins and alteration halos.
The subsequently introduced economic sulphide mineralization predominantly comprises sphalerite and galena with traces of native silver, tetrahedrite and chalcopyrite. Some of the individual ore lenses can be distinguished on the basis of their metal and gangue mineral assemblages. Alteration that is spatially associated with the late, weak, syntectonic Cu mineralisation includes pyrrhotite, siderite, ferroan ankerite, biotite, chlorite, muscovite and magnetite.
Four distinct styles of strata-bound Zn-Pb mineralisation are distinguished at George Fisher, based on the dominant sulphide minerals, grain size, structural setting and paragenesis (from Chapman, 2004):
• Layer-parallel disseminated sphalerite, which is conformable to bedding at a mm to cm scale, and stratabound at all other levels. The sphalerite is very fine grained, with µm to mm widths, and is subhedral to anhedral with a honey to light brown and red-brown colour. It is disseminated in mm to cm thick diffuse bands subparallel to host rock layering, and is very variable, comprising between 5 and 45% of a particular band. Individual sphalerite grains and aggregates mimic, or are finer grained than the host rock. However, at a mining scale, mineralisation of this style alone is not economic.
Where developed within siltstone, disseminated sphalerite is dispersed within quartz-calcite-ferroan dolomite laminations, but is rare in pyritic and carbonaceous bands. Where it is found within nodular carbonate layers, it occurs as isolated interstitial grains, infill after rhombohedral calcite, and as mm sized crystal aggregates with irregular grain contacts with carbonates. In mudstone, sphalerite of this type, locally with traces of galena, occurs as grains that are interstitial to carbonate and quartz and are variably intergrown with bitumen.
Sulphides occur as irregular 'flecks', aggregates, fine disseminations and concentrations that follow and mimic the sedimentary beds, including crossbeds. Some tuffaceous marker beds and white carbonate bands also contain disseminated sphalerite with characteristics that are similar to the mudstone hosted sphalerite.
These observations indicate disseminated sphalerite mineralisation was precipitated in open space within older nodular carbonate material (which itself transgresses stratigraphy at a district scale) and occurs as interstitial pore fillings in mudstones with bitumen. As such, zones of disseminated sphalerite are also discordant to bedding, as well as being discontinuous at the deposit scale, and replace layers that define current produced bedding at finer scales.
• Layer-parallel vein-hosted sphalerite, occurring as fine to medium grained, typically red-brown, semi-massive crystalline sphalerite in discrete layer-parallel bands that are interpreted to be veins. It is coarser grained than sphalerite in the disseminated layer-parallel style, and occurs interstitial to, and surrounds, gangue minerals that have preserved euhedral to subhedral outlines typical of material deposited in open fractures. These veins vary from 1 to 30 mm in thickness, averaging <10 mm, and either occur alone or in stacks of 5 to 10 veins over a width of 10 to 30 cm. They are usually found rhythmically intercalated with pyritic siltstones and at contacts between thin-banded pyritic siltstone and mudstone units. They have planar-parallel or folded margins, and variably sharp to diffuse boundaries. The latter occur where they are bounded by sedimentary layers with abundant disseminated sphalerite. These veins, which comprise 30 to 95 vol.% interstitial sphalerite also carry various combinations of paragenetically associated quartz, calcite ±K feldspar ±hyalophane [(K,Ba)Al(Si,Al)Si2O8] ±celsian [Ba(Al2Si2O8)] ±hydrophlogopite ±ferroan dolomite.
Silicate and carbonate vein filling minerals have significantly coarser grain sizes and are readily distinguishable from those of the wall-rocks. Some of the quartz and carbonate have relict euhedral crystal outlines, whilst all other associated minerals, except hydrophlogopite, have brecciated and corroded grain contacts with sphalerite. These relationships imply sphalerite and hydrophlogopite were deposited after carbonate, quartz and feldspar during a discrete but closely related episode of open-space vein fill. These textures are further interpreted to suggest earlier precipitated silicate and carbonate vein infill was brecciated on a small scale, dissolved and/or replaced by sphalerite during later vein opening and mineral precipitation. Layer-parallel disseminated, sphalerite and hydrophlogopite in the adjacent wall rocks are interpreted to be an alteration selvage to the veins (Chapman, 2004). Crenulation of sphalerite vein margins by GFS2 and intensification of the GFS2 cleavage along vein margins are taken to imply formation of sphalerite veins prior to GFD2.
• Breccia-hosted sphalerite, is characterised by a finer grain size and greater internal structural complexity compared to the vein-hosted sphalerite, although both have an overall strata-bound character. The breccia matrix is composed of very fine grained sphalerite with minor pyrrhotite and galena, enclosing 5 to 50 vol.% clasts. The clasts include irregular, mm to cm size subrounded fragments of folded and boudinaged mudstone and pyritic siltstone, sugary ferroan dolomite vein fragments, and aggregates of calcite ±ferroan dolomite ±K feldspar ±hyalophane ±quartz, similar to the infill in the layer-parallel sphalerite veins.
This brecciated mineralisation postdates sugary ferroan dolomite veining, which crosscuts both vein-hosted and layer-parallel disseminated sphalerite, but occur as clasts in the breccia. Textural relationships suggest breccia formation was synchronous with, or postdated GFF2 fold development.
Breccia-hosted sphalerite is interpreted to be the product of mechanical deformation of pre-existing vein-hosted sphalerite, resulting from strain partitioning in sphalerite veins due to rheological differences between sulphides, mudstone layers and ferroan dolomite veins at cm to tens of cm scales (Chapman, 2004).
• Vein and breccia hosted galena, comprises:
i). Discordant vein hosted galena composed of fine to coarse grained galena ±pyrite ±sphalerite ±pyrrhotite ±carbonate ±chlorite ±quartz. Subhedral galena is interstitial to euhedral pyrite, forming irregularly interlocking crystal aggregates with sphalerite and pyrrhotite. Veins generally have planar to irregular margins, are a few mm thick, and are discontinuous with tapering extremities. They typically form orthogonal networks perpendicular to bedding and are structurally continuous with galena breccias.
ii). Breccia hosted galena occurs as the following variants:
- Coarse-grained galena-rich breccias with a matrix principally composed of ~40 to 70% galena and accessory pyrite, enclosing fragments that vary from a few mm to a few cm in size to larger blocky to subrounded clasts that predominantly comprise mudstone and pyritic siltstone as well as layer-parallel, disseminated and vein-hosted sphalerite mineralisation. Additionally there are microscopic clasts of wall rocks and irregular, subrounded monominerallic silicate, carbonate or sphalerite. These breccias vary from 1 to 100 cm, but are mostly 2 to 10 cm thick, occurring as concordant bands with planar, parallel and discordant margins.
- Fine-grained galena-rich breccias, where the contained galena has a dull lustre and occurs as a fine grained 40 to 75% matrix enclosing microscopic to cm sized clasts similar to those of the coarse-grained galena breccias described above. Clasts usually have oval to spheroidal to elongate shapes and are rounded, sometimes with a bedding-parallel preferred orientation. These breccias occur as conformable bands with planar-parallel margins and range from 1 to 15 cm in thickness.
- Mixed sulphide-rich breccias comprise ~70% matrix composed of variable amounts of fine-grained galena, sphalerite, pyrrhotite and pyrite. These sulphides are irregularly distributed, producing mineralogically distinct bands to wavy and flamelike domains within the breccia matrix at a mm to cm scale. Clasts range from sub-mm to a few cm in size, and are typically well rounded to ovoid to irregular in shape, and include fragments of variably folded host rock, layer parallel, disseminated and vein hosted sphalerite mineralisation, as well as monomineralic calcite, ferroan dolomite and quartz. These breccias are typically 3 to 10 cm thick and have sharp to wavy margins, subparallel to bedding.
There is a consistent timing relationship of galena rich mineralisation compared to all zinc mineralisation styles on a deposit scale, indicating it represent the youngest ore-forming event at George Fisher. The discordant galena veins are structurally continuous with the breccias, indicating the two are coeval. The veins commonly occur in NW-SE to NE-SW trending conjugate sets that crosscut GFF2 and GFF3 folds, with an orientation compatible with their formation during GFF4 fold development.
After extensive Ph.D. investigations of the George Fisher North ore deposit and hosts at all scales, Chapman (1999; 2001; 2004) concluded that the earliest sphalerite mineralisation is hosted by bedding parallel veins. Sphalerite in these veins occurs as infill with hydrophlogopite, and as an apparent alteration product of precursor carbonate-quartz-Ba-K feldspar vein fill. Layer-parallel, disseminated sphalerite occurs as infill after the replacement of carbonate in siltstones, mudstones and nodular carbonate layers and is interpreted to represent a halo to the veins. It was further observed that the earliest sphalerite mineralisation postdated the emplacement of nodular carbonate, which transgresses stratigraphy at a district scale, and occurred prior to district-wide folding marked by the onset of GFD2 at George Fisher. The emplacement of galena in late tectonic structural sites post-dated all sphalerite mineralisation and was emplaced during GFD4 deformation. There is an intimate deposit scale spatial association and grade variation between galena and sphalerite, suggesting a common origin for Zn and Pb and remobilisation in the late stages of deformation, supported by the observation of significant
textural reconstitution of ore during regional folding. Mineralisation styles indicative of sea-floor deposition were not observed, although their complete obliteration and remobilisation cannot be entirely excluded.
Cave et al. (2023) have studied the temporal and petrological relationship between dolerite dykes and the adjacent Zn-Pb-Ag mineralisation in the George Fisher and Hilton deposits. In drill core, the dolerite dykes occur as a light grey to grey-brown rocks that are commonly overprinted along their margins by Zn-Pb-Ag mineralisation. The results and conclusions of this study are as follows:
• Apatite U-Pb geochronology gave an intrusive age of 1619 ±22 Ma and 1611 ±21 Ma for the dolerite dykes at the George Fisher and
Hilton Zn-Pb-Ag deposits respectively. These are ages consistent with intrusion during the early stages of the 1.62 to 1.50 Ga Isan Orogeny.
• The dolerite dykes have been subjected to multiple stages of hydrothermal alteration/veining, including: i). quartz-albite-K feldspar veining/alteration; ii). dolomite alteration; iii). biotite-chlorite-sulphide alteration; and iv). paragenetically late dolomite, chlorite, and sulphide veining. These alteration assemblages have been correlated with those observed in the adjacent George Fisher and Hilton orebodies.
• U-Pb monazite geochronology of quartz-albite-K feldspar veining overprinting the dolerite dyke at Hilton produced an age of 1513 ±16 Ma, constraining the maximum age of alteration within the dolerite dykes.
• A weighted mean age of 1501 ±32 Ma was yielded by in-situ Lu-Hf geochronology of pre-ore calcite at the George Fisher Zn-Pb-Ag deposit, whilst a late calcite-sphalerite vein from the George Fisher Zn-Pb-Ag deposit produced a weighted mean age of 1289 ±26 Ma.
• The 1513 ±16 Ma age of quartz-albite-K feldspar veining in the Hilton dyke, combined with the Lu-Hf age of pre-mineralisation calcite from the George Fisher Zn-Pb-Ag deposit, have been interpreted to suggest an epigenetic syn-deformational metallogenic event is favoured for the emplacement of Zn-Pb-Ag mineralisation. Specifically, Cave et al. (2023) suggest these data indicate the dolerite dykes intruded during the early Isan Orogeny at ~1620 Ma, and experienced subsequent hydrothermal alteration during D3 of the Isan Orogeny, coeval with Zn-Pb-Ag mineralisation.
• Late faulting and the displacement of the Zn-Pb-Ag orebodies at the George Fisher and Hilton deposits are interpreted to be coeval with the age of a late calcite-sphalerite vein at 1289 ±26 Ma.
George Fisher North is concealed and is partially connected to George Fisher South (Hilton) on its northern margin. Both mines are underground operations and are owned and operated by Glencore's Mt Isa Zinc Division.
Sub-economic mineralisation follows the strike of the Urquhart Shale for 4 km to the north and south of the two economic orebodies.
The original resources were estimated as follows (Forrestal 1990, Valenta 1994, Chapman 2004):
George Fisher South (Hilton): 120 Mt @ 10.2% Zn, 5.5% Pb, 100 g/t Ag,
George Fisher North: 108 Mt @ 11.1% Zn, 5.4% Pb, 93 g/t Ag.
JORC compliant Ore Reserves and Mineral Resources (at June 2006) were as follows (X-Strata 2007):
George Fisher South underground
Proved reserves - 12.5 Mt @ 8.3% Zn, 5.7% Pb, 127 g/t Ag
Probable reserves - 5.9 Mt @ 7.8% Zn, 5.8% Pb, 126 g/t Ag
Measured resources - 25.3 Mt @ 9.7% Zn, 6.9% Pb, 150 g/t Ag
Indicated resources - 10.6 Mt @ 9.2% Zn, 6.6% Pb, 139 g/t Ag
Inferred resources - 10 Mt @ 10% Zn, 6% Pb, 100 g/t Ag
George Fisher North underground
Proved reserves - 11.3 Mt @ 8.9% Zn, 4.7% Pb, 91 g/t Ag
Probable reserves - 15.1 Mt @ 8.3% Zn, 3.9% Pb, 75 g/t Ag
Measured resources - 14.5 Mt @ 10.4% Zn, 5.2% Pb, 101 g/t Ag
Indicated resources - 27.9 Mt @ 9.5% Zn, 4.0% Pb, 74 g/t Ag
Inferred resources - 45 Mt @ 9% Zn, 4% Pb, 80 g/t Ag
Mine production in 2005 totalled 2.7 Mt @ 8.3% Zn, 5.0% Pb, 115 g/t Ag.
JORC compliant Ore Reserves and Mineral Resources (at 31 December, 2011) were as follows (X-Strata 2012):
George Fisher South underground
Proved + probable reserves - 19.6 Mt @ 6.5% Zn, 4.4% Pb, 96 g/t Ag
Measured + indicated resources - 49.8 Mt @ 8.6% Zn, 5.9% Pb, 125 g/t Ag
Inferred resources - 23 Mt @ 8% Zn, 5% Pb, 113 g/t Ag
George Fisher North underground
Proved + probable reserves - 61.8 Mt @ 7.5% Zn, 3.6% Pb, 62 g/t Ag
Measured +indicated resources - 106.2 Mt @ 8.6% Zn, 3.7% Pb, 63 g/t Ag
Inferred resources - 65 Mt @ 8% Zn, 4% Pb, 69 g/t Ag
Handlebar Hill open pit primary
Proved + probable reserves - 2.1 Mt @ 7.6% Zn, 3.4% Pb, 54 g/t Ag
Measured +indicated resources - 6.9 Mt @ 6.9% Zn, 2.3% Pb, 43 g/t Ag
Inferred resources - 1 Mt @ 5% Zn, 2% Pb, 30 g/t Ag
Handlebar Hill open pit oxide
Proved + probable reserves - 0.5 Mt @ 0.4% Zn, 8.5% Pb, 89 g/t Ag
Measured +indicated resources - 0.6 Mt @ 0.4% Zn, 7.8% Pb, 85 g/t Ag
Inferred resources - Nil
JORC compliant Ore Reserves and Mineral Resources (at 31 December, 2018) were as follows (Glencore Resources and Reserves report, 2018):
George Fisher South underground
Proved + probable reserves - 16.7 Mt @ 6.2% Zn, 4.4% Pb, 99 g/t Ag
Measured + indicated resources - 56 Mt @ 8.3% Zn, 5.0% Pb, 106 g/t Ag
Inferred resources - 27 Mt @ 8% Zn, 4% Pb, 87 g/t Ag
George Fisher North underground
Proved + probable reserves - 72 Mt @ 7.0% Zn, 3.1% Pb, 52 g/t Ag
Measured +indicated resources - 168 Mt @ 8.9% Zn, 3.5% Pb, 55 g/t Ag
Inferred resources - 53 Mt @ 9% Zn, 4% Pb, 57 g/t Ag
Handlebar Hill open pit primary
Proved + probable reserves - Nil
Measured +indicated resources - 5.2 Mt @ 6.6% Zn, 2.2% Pb, 37 g/t Ag
Inferred resources - 0.8 Mt @ 5% Zn, 2% Pb, 30 g/t Ag
Handlebar Hill open pit oxide
Proved + probable reserves - 0.5 Mt @ 0.4% Zn, 8.5% Pb, 89 g/t Ag
Measured +indicated resources - 0.6 Mt @ 0.4% Zn, 7.8% Pb, 85 g/t Ag
Inferred resources - Nil
NOTE: Mineral Resources are inclusive of Ore Reserves.
Mine production at George Fisher North and South for the period 1 January 2018 to 31 December 2018 totalled:
2.9 Mt @ 7.3% Zn, 3.9% Pb, 68g/t Ag.
The most recent source geological information used to prepare this decription was dated: 2007.
This description is a summary from published sources, the chief of which are listed below. © Copyright Porter GeoConsultancy Pty Ltd. Unauthorised copying, reproduction, storage or dissemination prohibited.
George Fisher North George Fisher South (Hilton)
|
|
Betts P G, Giles D, Lister G S 2003 - Tectonic environment of shale-hosted massive sulfide Pb-Zn-Ag deposits of Proterozoic northeastern Australia: in Econ. Geol. v98 pp 557-576
|
Betts, P.G., Giles, D., Mark, G., Lister, G.S., Goleby, B.R. and Ailleres, L., 2006 - Synthesis of the Proterozoic evolution of the Mt Isa Inlier: in Australian J. of Earth Sciences v.53, pp. 187-211.
|
Bojcevski, D., Link, L., Johnson, N.W., Landmark, V., Johnston, M., Mackenzie, J. and Young, M.F., 1998 - Metallurgical Characterisation of George Fisher Ore Textures and Implications for Ore Processing: in Mill to Mine Conference, 1998, Brisbane, Queensland, 11-14 October, 1998 AusIMM, Melboune, Proceedings pp. 29-41.
|
Cave, B., Lilly, R., Simpson, A. and McGee, L., 2023 - A revised model for the George Fisher and Hilton Zn-Pb-Ag deposits, NW Queensland: Insights from the geology, age and alteration of the local dolerite dykes: in Ore Geology Reviews v.154, 17p. doi.org/10.1016/j.oregeorev.2023.105311.
|
Chapman L H, 2004 - Geology and Mineralization Styles of the George Fisher Zn-Pb-Ag Deposit, Mount Isa, Australia: in Econ. Geol. v99 pp 233-255
|
Conaghan, E.L., Hannan, K.W. and Tolman, J. 2003 - Mount Isa Cu and Pb-Zn-Ag deposits, NW Queensland Australia: in Regolith Expression of Australian Ore Systems - A compilation of geochemical case histories and conceptual models CRC LEME online, http://crcleme.org.au/RegExpOre/MtIsa.pdf, 3p.
|
Forrestal P J 1990 - Mount Isa and Hilton silver-lead-zinc deposits: in Hughes FE (Ed.), 1990 Geology of the Mineral Deposits of Australia & Papua New Guinea The AusIMM, Melbourne Mono 14, v1 pp 927-934
|
Gibson, G.M., Hutton, L.J. and Holzschuh, J., 2017 - Basin inversion and supercontinent assembly as drivers of sediment-hosted Pb-Zn mineralization in the Mount Isa region, northern Australia: in Journal of the Geological Society v.174, pp. 773-786.
|
Huston, D.L., Champion, D.C., Czarnota, K., Duan, J., Hutchens, M., Paradis, S., Hoggard, M., Ware, B., Gibson, G.M. Doublier, M.P., Kelley, K., McCafferty, A., Hayward, N., Richards, F., Tessalina, S. and Carr, G., 2023 - Zinc on the edge - isotopic and geophysical evidence that cratonic edges control world-class shale-hosted zinc-lead deposits: in Mineralium Deposita v.58, pp. 707-729.
|
Lilly, R., Taylor, D. and Spanswick, N. 2017 - Mount Isa Cu-Pb-Zn deposit including George Fisher: in Phillips, G.N., 2017 Australian Ore Deposits, The Australasian Institute of Mining and Metallurgy, Mono 32, pp. 473-478.
|
Mathias B V, Clark G J 1975 - Mount Isa copper and silver-lead-zinc orebodies - Isa and Hilton mines: in Knight C L, (Ed.), 1975 Economic Geology of Australia & Papua New Guinea The AusIMM, Melbourne Mono 5 pp 351-372
|
Perkins W G, Bell T H 1998 - Stratiform replacement lead-zinc deposits: a comparison between Mt Isa, Hilton and McArthur River: in Econ. Geol. v93 pp 1190-1212
|
Polito, P.A., Kyser, T.K., Southgate, P.N. and Jackson, M.J., 2006 - Sandstone Diagenesis in the Mount Isa Basin: An Isotopic and Fluid Inclusion Perspective in Relation to District-Wide Zn, Pb, and Cu Mineralization: in Econ. Geol. v.101, pp. 1159-1188.
|
Rieger, P., Magnall, J.M., Gleeson, S.A., Lilly, R., Rocholl, A. and Kusebauch, C., 2020 - Sulfur Isotope Constraints on the Conditions of Pyrite Formation in the Paleoproterozoic Urquhart Shale Formation and George Fisher Zn-Pb-Ag Deposit, Northern Australia: in Econ. Geol. v.115, pp. 1003-1020.
|
Rieger, P., Magnall, J.M., Gleeson, S.A., Oelze, M., Wilke, F.D.H. and Lilly, R., 2022 - Differentiating between hydrothermal and diagenetic carbonate using rare earth element and yttrium (REE+Y) geochemistry: a case study from the Paleoproterozoic George Fisher massive sulfide Zn deposit, Mount Isa, Australia: in Mineralium Deposita v.57, pp. 187-206.
|
Valenta R 1994 - Syntectonic discordant Copper mineralization in the Hilton Mine, Mount Isa: in Econ. Geol. v 89 pp 1031-1052
|
Valenta R 1994 - Deformation of host rocks and stratiform mineralization in the Hilton mine area, Mt Isa: in Australian J. of Earth Sciences v41 pp 429-443
|
Valenta, R.K., 1989 - Vein geometry in the Hilton area, Mount Isa, Queensland: implications for fluid behaviour during deformation: in Tectonophysics v.158, pp. 191-207.
|
Porter GeoConsultancy Pty Ltd (PorterGeo) provides access to this database at no charge. It is largely based on scientific papers and reports in the public domain, and was current when the sources consulted were published. While PorterGeo endeavour to ensure the information was accurate at the time of compilation and subsequent updating, PorterGeo, its employees and servants: i). do not warrant, or make any representation regarding the use, or results of the use of the information contained herein as to its correctness, accuracy, currency, or otherwise; and ii). expressly disclaim all liability or responsibility to any person using the information or conclusions contained herein.
|
Top | Search Again | PGC Home | Terms & Conditions
|
|