Mount Isa, Mt Isa, Zinc |
|
Queensland, Qld, Australia |
Main commodities:
Zn Pb Ag
|
|
 |
|
 |
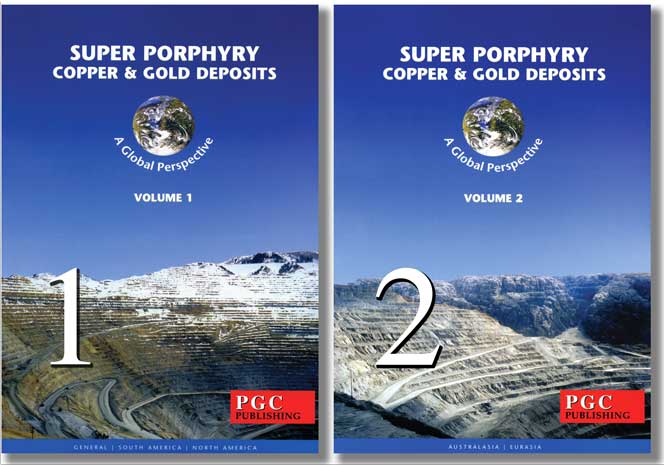 |
Super Porphyry Cu and Au

|
IOCG Deposits - 70 papers
|
All papers now Open Access.
Available as Full Text for direct download or on request. |
|
 |
The Mount Isa zinc-lead-silver orebodies are located within north-west Queensland, Australia ~975 km west of the Pacific Ocean port of Townsville (#Location: 20° 42' 58"S, 139° 28' 33"E).
Lead-zinc mineralisation was discovered at what is now Mount Isa in March 1923 by prospector John Campbell Miles. Mount Isa Mines Ltd (MIM) was formed in 1924 to develop the minerals discovered by Miles. The railway to Townsville was extended to Mount Isa in 1929, acting as a catalyst for the expansion of activity in the Mount Isa district. The US company ASARCO aided in the financing, proving and development of the deposit, which after a 5 year surface diamond drilling program of 11 000 m had reserves of 32 Mt of high grade ore by 1930. Mining commenced in 1931, employing the most modern mechanised mining equipment, although treatment problems and poor recoveries meant that the mine was not profitable until the 1936-1937 financial year, and in 1947, MIM paid its first dividend. The Hilton and later defined George Fisher deposits, 20 km north along strike from Mount Isa, were discovered when outcropping cerussite was recognised by S R Carter in 1947. However, production did not commenced at Hilton until 1987 and 2000 at George Fisher.
The potential for copper at Mount Isa was only explored during the 1940 and 1950s, although 15 m of 17% Cu was intersected in a hole targeting Zn-Pb mineralisation in 1927. In 1930 another intersection of 8.8 m at 8.5% Cu cut part of the 650 orebody. These intersections were largely ignored until a need for Cu during World War II, when copper mining commenced in 1943, but was closed down in 1944. Exploration following World War II culminated in the discovery of the 1100 orebody in 1954, with a drill hole intersection of 202 m @ 2.2% Cu (Perkins,1990). The subsequent re-commencement of Cu mining resulted in a doubling of mine production, an increase in the profitability of the operation and allowed MIM to thrive.
By 1960 ASARCO held 53% of MIM's shares, but gradually sold down its interest until finally exiting in 1996. Xstrata Limited purchased Mount Isa Mines in 2003 and split the operation into two separate streams, a copper and a zinc-lead-silver operation.
The Black Star open pit zinc mine began production in 2004. In December 2005, after 75 years of almost continuous operation, the underground Zn-Pb-Ag mining at Mount Isa Zinc had ceased, whilst the Black Star open pit was closed in 2016 after exhaustion of available reserves.
In 2013, Xstrata merged with Glencore to become Glencore Xstrata plc, which in May 2014, became Glencore plc, that wholly owns MIM.
In the mine area, the Zn-Pb ore and pyritic lenses were expressed at surface as silicified and ferruginised gossans that are 1 to 5 m high, occurring as strike parallel ribs along low hills that rise to 5 to 25 m above the alluvial flats of the adjacent parallel Leichhardt River. Overbank sands deposited from local ephemeral creeks thinly mantle the valley floors and coalesce with equally thin (<3 m) aprons of colluvium shed from the gossanous hills. The ferruginous and siliceous gossans are remnants of middle to upper saprolite that developed locally from the intense acid leaching of sulphide lodes. Manganese oxide coatings, rich in Pb, Zn and Ba have been dated at 15 to 21 Ma, within a wider band of Tertiary age Mn oxide minerals in the district. These reflect supergene enrichment, leaching and gossan development over the deposit probably during the same wet periods of the Miocene that formed the widespread silcrete and laterite that survives as eroded relicts on isolated mesas and plateaux (Conaghan et al., 2003).
Geological Setting
The regional, district and deposit scale setting, geology and structure of the Mount Isa - George Fisher district are described in the record for the George Fisher deposit. George Fisher is 20 km to the north of Mount Isa in the same host sequence and, like Mount Isa, is located immediately to the east of the multi-stage Paroo-Mount Isa Fault complex.
As at George Fisher, the Mt Isa Zn-Pb-Ag orebodies are hosted within the Late Palaeoproterozoic (1655 Ma) Urquhart Shale of the Mt Isa Group, which lies within the Leichhardt River Fault Trough, and was deposited in the Isa Superbasin in the Western Fold Belt of the Mt Isa Inlier. The Leichhardt River Fault Trough lies immediately to the east of the Lawn Hill Platform that embraces the Century and Lady Loretta sediment hosted Zn-Pb-Ag deposits, some 120 and 250 km to the NNW respectively. The Mt Isa Group interfingers with, but underlies the bulk of the McNamara Group which is the host to Century and Lady Loretta.
In the Mt Isa mine area, the Mt Isa Group strikes north-south and has a persistent westerly dip of 60 to 65°. It is up to 4000 m in thickness and comprises a sequence of alternating units of dolomitic shale and dolomitic siltstone, with relatively minor conglomerate and sandstone at the base. This succession, which was deposited within the Isa Superbasin, unconformably overlies both clastic sedimentary rocks of the Calvert Superbasin and the thick mafic volcanic rocks and quartzites of the older Leichhardt Superbasin which includes the 6 to 15 km thick Eastern Creek Volcanics.
The host Urquhart Shale, which occurs towards the top of the Mount Isa Group, is a carbonaceous, pyritic, dolomitic siltstone ~1000 m thick. At Mount Isa, the Urquhart Shale is characterised by a well-laminated sequence of current-deposited siltstones and mudstones (Neudert, 1983, 1988), hosting both the lead-zinc and copper orebodies. At Mount Isa, Neudert (1983) and Painter et al. (1999) recognised the following interbedded facies within the upper sections of the unit:
• Rhythmite facies - which comprises the bulk of the Urquhart Shale and hosts all on the known Zn-Pb-Ag and fine-grained pyrite mineralisation. The beds of this facies typically comprise rhythmically alternating black to charcoal coloured carbonaceous laminated siltstone, and grey and black mudstone. The basal laminated siltstone in each rhythmic cycle typically hosts concentrations of fine grained pyrite, sphalerite and galena which often obliterate graded bedding textures. Locally abundant current-produced sedimentary structures that include cross laminations, graded bedding and flutes are observed throughout the facies. It can be subdivided into mudstone, muddy rhythmite and silty rhythmite sub-facies. Various pseudomorphs, including carbonate nodules and carbonate crusts which are commonly associated with mudstones and laminated siltstones in the lower third of the Urquhart Shale are interpreted to represent evaporite textures. These pseudomorphs are apparently after gypsum, anhydrite and glauberite, but not halite. Halite has only been encountered above the Urquhart Shale.
• Carbonate-cemented siltstone facies - which is composed of siltstone to fine sandstone with local cross bedding and flutes, that are cemented by calcite or ferroan dolomite. It is distinguished by a light grey bleached appearance and contains no evaporitic textures, base metals or fine grained pyrite, but does carry sporadic sub- to euhedral pyrite crystals. In zones of more intense cementation, sedimentary structures and textures are obliterated, and stylolites filled with carbonaceous material are developed. Where cementation is patchy, local concentrations or nodules are evident, with textures indicative of differential compaction, suggesting cementation occurred prior to significant compaction (Neudert, 1983).
• Cross-laminite facies - which forms a 5 to 11 m thick marker bed towards the centre of the Urquhart Shale. It is composed of light grey, fine sandstones, with irregular course carbonaceous Iaminae that frequently contain fine pyrite, interbedded with pyrite bearing mudstone and siltstone similar to the rhythmite facies. The fine sandstones are composed of dolomitic peloids and abundant detrital quartz and K feldspar. The cement in the sandstones is typically composed of ferroan dolomite to ankerite with similar pre-compaction characteristics to the carbonate cemented siltstone facies. Evaporatic textures are also absent (Painter et al., 1999).
These facies, other than the cross-laminite, are not as readily distinguished in the lower third of the Urquhart Shale, which Neudert (1983) grouped into a non-channelled and a nodular facies. The only well developed marker in this interval is a 0.5 m thick flat-pebble conglomerate (Painter et al., 1999). Neudert (1984) regarded the nodules of the nodular dolomite facies as being after evaporitic sulphate. These nodules are much more abundant in the lower third of the Urquhart Shale. Thin tuffaceous marker beds, mostly composed of grey-coloured potassium-altered layers of volcanic material, are present throughout the sequence and allow correlation between drill hole intersections and exposures in underground workings.
Sub-units within the Urquhart Shale are well defined, tabular, and can be traced throughout the deposit area. A package of alternating carbonate cemented siltstone and rhythmite facies in the middle of the Urquhart Shale hosts to the highest grade Zn-Pb-Ag orebodies. Fine grained pyritic bands, known as 'pyite ribs' typically extend over lateral distances of several kilometres, only carrying low grade Zn-Pb-Ag mineralisation (Painter et al., 1999).
The Urquhart Shale is overlain by the Spear and Kennedy 'siltstones', a thicker-bedded sequence of dolomitic siltstones and sandstones, followed by a shaley unit, which is truncated to the west by the Paroo Fault (Perkins, 1996).
Mineralisation at the Mt Isa mine is distributed over much of the thickness of the Urquhart Shale, although the bulk of the ore is within the upper 650 m, confined to a strike length of ~2000 m and down dip extent of ~1200 m.
The Mt Isa mineralised system is developed immediately east of, and adjacent to, the major north-south trending, multistage, Mount Isa-Paroo Fault Complex which juxtaposes the host Mount Isa Group against the older Eastern Creek Volcanics to the west and at depth.
The Paroo Fault is interpreted to have originally formed as a listric extensional fault during deposition of the Kennedy Siltstone and Magazine Shale (Long, 2010), but may be a reactivated predecessor structure that bounded a half graben during Leichhardt Superbasin deposition (Lister, 2002; Betts et al., 2003). The related Mount Isa Fault was active by D2. Whilst both the Paroo and main Mount Isa faults were reactivated by brittle deformation during D2 and D3 to D4, Long (2010) and McLellan et al. (2014) propose that the Paroo Fault was folded during these deformations, while also undergoing reverse shearing. Long (2010) suggests this folding was influenced by uplift of the Sybella batholith which acted as a buttress during D2 shortening (Betts and Lister, 2002). The main Mount Isa and Paroo Faults are broadly parallel at surface to the west of the Mount Isa deposit, northward to beyond George Fisher. Over this interval, the Paroo Fault is in the footwall, and immediately east, of the steeply west dipping Mount Isa Fault. Down dip at Mount Isa however, the Paroo Fault diverges eastward from the Mount Isa Fault where it takes the form of gently north plunging, fault dislocated synform and then an antiform, before dipping steeply east to depth. As a result, the 60 to 65°W dipping Mount Isa Group was truncated at depth by the flat lying section of the folded Paroo Fault and juxtaposed with the underlying similarly steeply dipping Eastern Creek Volcanics and Guide Quartzite at 1655±4 Ma (Southgate et al., 2000; Valenta, 1994; Perkins et al., 1999). To the south of the Mount Isa deposit, this fold is evident where it intersects the surface, and the Paroo Fault diverges sharply from the main Mount Isa Fault juxtaposing the same two sequences. Deformation related to reactivation of these two faults is the major manifestation of brittle D4 fracture in deposits of the Mount Isa district (Chapman, 2004). D2 and D3 shearing and reverse displacement along the folded Paroo Fault is regarded as being important in producing zones of dilation exploited by mineralising fluids (Long, 2010).
The Mount Isa Cu and Zn-Pb-Ag orebodies are situated in the brecciated Urquhart Shale in the hanging wall of the Paroo Fault, and in shear zones that splay upward from that structure to parallel the stratigraphy up-dip, to then follow sheared bedding planes. There is a distinct zonation of mineralisation within this system, with the Mount Isa copper orebodies mostly concentrated adjacent, and parallel, to the Paroo fault. These orebodies split into fingers that follow sheared splays upward, declining in intensity before pinching-out, sometimes as much as almost a km up-dip. The Zn-Pb-Ag orebodies, which have a strong stratabound control, overlap the Cu mineralisation, but are generally more distal to the Paroo Fault. They extending from as little as a few hundred metres from the fault, and progress from Pb- to Zn-rich distal from the same structure, persisting to more than a kilometre beyond the outer limits of Cu mineralisation. The southern and down-dip extremities the Mount Isa Zn-Pb-Ag mineralisation interfinger with, and are generally replaced by, lobes of silica-dolomite, the local term for breccia and bedding-replacive, multipulse vein and breccia replacement by masses of dolomite and quartz that envelope to the Mount Isa Cu orebodies.
Mineralisation
The Mount Isa Zn-Pb-Ag deposit is composed of a series of orebodies which are ovoid disc-shaped sheets that parallel stratigraphy, with long axes of up to 2 km. The intermediate axes of these orebodies are between 250 and 1000 m, decreasing from west to east across the deposit. Examples include 5 Orebody which has a strike length of ~2000 m, width of up to 35 m and a vertical extent of ~1000 m, whilst 12 Orebody further to the east and lower in the sequence has respective dimensions of ~1500 x ~10 x ~1000 m. These orebodies are composed of individual sulphide bands of galena and sphalerite, with fine pyrite and pyrrhotite which closely follow bedding and are typically ~40 cm, but range from 1 mm to 1 m in thickness and may extend for up to several hundred metres along strike and down-dip. These sulphide bands are interlayered with unmineralised siltstones that are from millimetres to tens of metres in thickness. The bedding/banding within these mineralised packages may be planar or intensely folded (Davis, 2004).
The individual orebodies are bedding-parallel at a mesoscopic scale, although correlation of tuffaceous marker beds demonstrate that concentration of mineralisation transgresses stratigraphy towards the hanging wall in an up-dip and northerly direction (Lilly et al., 2017).
The sulphide bands within these orebodies display textures consistent with replacement of metasomatic alteration minerals, mostly dolomite, and occur in a number of styles, including i). finely laminated stratabound pyritic bands; ii). generally sphalerite rich stratabound disseminated layers with variable galena, pyrrhotite and pyrite; iii). folded galena-rich bands, veins and breccias sub-parallel to bedding; iv). distinctly cross-cutting replacement zones, veins and breccias of sulphides of all types (Perkins, 1996).
Where these bands and zones of sulphides are grouped together in sufficient density to provide economic grade and thicknesses they constitute an orebody. Some 30 such stacked 'orebodies' are defined over the upper 650 m of the Urquhart Shale, ranging from <10 to >50 m in thickness (Lilly et al., 2017).
The Zn-Pb-Ag orebodies partially surround and extend up-dip and to the north of crudely stratabound marginal lobes of silica-dolomite alteration and Cu mineralisation. The boundaries of these Zn-Pb-Ag orebodies are economic grade shells enclosing packages of both sulphides and barren rock as described above. However, they generally have relatively abrupt hanging and footwalls and form natural tabular bands of sulphide-rich stratigraphy that collectively define a distinctive en echelon pattern in both plan and cross-section. The envelope enclosing these orebodies plunges at from 23 to 60° toward an azimuth of 323 to 348°, parallel to fold hinges and to the limits of silica-dolomite alteration halos that surround the copper orebodies. The more westerly orebodies (Black Star Numbers 1 to 5) occur at higher stratigraphic levels and in more northerly positions, whilst the narrower Racecourse orebodies (5/60 to 14/30) occupying progressively deeper and more southerly sites. Further to the south, the less well-developed Rio Grande Zn-Pb-Ag orebodies occur as a halo to the silica-dolomite alteration and the major 1100 copper orebody.
Folding with amplitudes of up to ~10 m affect the orebodies, although larger folds, such as the Mount Isa Fold on the northern margin of the higher grade orebodies, are up to 200 m across. These structures generally plunge at 30 to 50°N. The larger of these folds and associated faults can be traced for hundreds of metres both horizontally and vertically. Where these structures fold the orebodies, there is considerable enrichment of sulphides, particularly galena, within the fold hinges. This folding is commonly very disharmonic.
Pyrite is the earliest sulphide at Mount Isa, occurring as: i). coarse-grained euhedra, directly related to hydrothermal events that emplaced both the Cu and Pb-Zn mineralisation, and is present as irregular disseminations and less commonly as conformable zones in the forms described above (Croxford 1962; Gulson et al.,1983; Perkins 1984) and ii). finely laminated stratabound bands of fine grained pyrite, as also described above, that occurs in a variety of forms, many of which can occur together. One of the most common variants is found as either single euhedral to subhedral 'brassy' crystals, or aggregates, that are generally 20 to 200 µm across, ranging from sparsely (~5%) disseminated, to mono-minerallic pyrite bands, the latter often terminated by fine fractures and veins. The second main variety consists of a complex array of brown, finely zoned, bedding parallel pyrite crystals that vary from 2 to 20 µm in size, and are found at a density ranging from <0.1 to 60% of a bed. Combinations of these types of pyrite may vary from ovoid, to worm like or interlocking lath like brassy pyrite grains and aggregates varying from 1 to 20 mm across within continuous trains of fine grained wavy or anastomosing pyrite. Where individual pyrite grains are closely packed, the anastomosing pattern encloses small augen of carbonaceous siltstone that have long axes of 0.3 to 3 mm (Perkins, 1996).
Neudert (1983) noted and antithetic relationship between the amount of ferroan dolomite present as detrital grains or micritic pre-compaction cements in the carbonate cemented siltstone and across laminate facies, and the amount of fine-grained pyrite. This was interpreted to suggest the ferroan dolomite was source of iron in the pyrite which was released in solution during replacement of the dolomite (Neudert 1983; Painter et al., 1999). However, Painter (2003) showed that dolomite within the Urquhart Shale That are within ~1 km of economic Zn-Pb-Ag mineralisation are elevated in Fe compared to more distal dolomite. The same author also showed an increase in the occurrence of manganiferous dolomite cements associated with mineralisation.
A detailed microscopic study of the fine-grained stratabound pyrite indicates that it has overprinted carbonaceous stylo-laminae and locally carbonaceous cleavage (Perkins, 1998). Perkins (1998) also observed microstructural evidence he interpreted to show precipitation of fine grained pyrite was late replacive, overprinting both the S2 cleavage sub-parallel to bedding and cross-cutting S3 cleavage which is axial planar to the mesoscopic folds in the deposit. The resulting pyrite-bearing shale is more abundant to the north, and may have had a rheological influence on the distribution and localisation of later alteration and ore-bearing fluids. Pyrrhotite has been developed at the expense of fine-grained pyrite to the south and down-dip towards the Cu orebodies, where it becomes the dominant iron sulphide in much of the Pb-Zn-Ag mineralisation, and has associated bleaching and fine-grained phyllosilicate growth. Pyrrhotite commonly occurs as either disseminated lath-like, or bladed aggregates which are arranged irregularly along, and randomly oriented to bedding and as more or less continuous bands along bedding. Perkins (1998) interprets the structural constraints on mineralisation and timing criteria to place the pyrrhotite and layer-parallel pyrite as having formed late in the last major phase of deformation affecting the Mount Isa deposit area.
Ore textures suggest galena and sphalerite are replacive, predominantly of bedding-parallel, coarsely crystalline dolomite (Grondijis and Schouten, 1937; Perkins, 1997). The bulk of the galena occurs in near-bedding parallel breccia veins that contain variable sphalerite, whilst silver, occurring as freibergite, is closely associated with galena, but cannot be distinguished other than in polished section (Perkins, 1996). Most galena is found as either subhedral aggregates when associated with coarse-grained gangue minerals, or as connected wisps and lenses where associated with finer-grained dolomitic siltstones. Cross-cutting variants are generally coarser-grained. Much of the sphalerite occurs as fine-grained aggregates in minimally altered feldspathic siltstone, but where associated with coarse-grained gangue minerals occurs as almost continuous aggregates, and is invariably accompanied by galena. The relationship of galena and sphalerite and their boundaries changes according to the volumetric proportion of galena. Where galena is <20% of the total sulphide volume, it occurs as strings of fine blebs between sphalerite aggregates, or as cusp-shaped forms within them. As the galena proportion increases, the sphalerite becomes more rounded. At high quantities of galena, sphalerite appears as subrounded inclusions or lenses.
Galena and friebergite rich ore is concentrated towards the silica-dolomite alteration down-dip and to the south, whilst sphalerite increases up-dip and to the north (Mathias and Clark, 1975; Davis, 2004). Ore textures indicate galena and sphalerite are replacive, predominantly after bedding-parallel, coarsely crystalline dolomite alteration (Grondijis and Schouten, 1937; Perkins, 1997). Higher ore grades can occur in folded zones, as detailed above (McDonald, 1970; Davis, 2004). This had previously been attributed to remobilisation, but are regarded by Perkins (1997) to be due to preferential bedding- and fracture-controlled dolomite replacement at these sites. Chalcopyrite is a very minor phase in galena-rich bands, although it occurs as inclusions within Pb-Zn sulphides, most commonly in the southern and deeper parts of the mine where the Zn-Pb-Ag orebodies locally interfinger with and are overprinted by the silica-dolomite alteration in the transition to the Cu orebodies (Andrew, 1989).
Stratigraphic sampling of the orebody by Perkins (1998) indicated progressive development of an incipient to pervasive alteration down-dip and laterally towards the south that overprinted the well-laminated host carbonaceous siltstones and mudstones. At the periphery, alteration comprises bleaching by the destruction of carbonaceous seams and enhanced growth of dolomite and K feldspar. Further in the same direction, alteration changes to white mica (sericite) and albite-bearing 'buff alteration', commonly also containing siderite, biotite, magnetite and stilpnomelane, through a chloritic zone, ultimately grading into a massive 'silica-dolomite' assemblage (Perkins, 1998; Swager, Perkins and Knights, 1987). The progression from an abrupt pyrite to pyrrhotite transition, and a zonation from sphalerite to galena to chalcopyrite accompanied this alteration train. Perkins (1998) has interpreted these relationships to indicate the lead-zinc-silver mineralisation at Mount Isa developed cogenetically with the large-scale Cu orebodies by late syndeformational replacement processes.
A systematically outward diminishing halo of enriched stratabound Zn-Pb-Ag and pyrite can be laterally traced within the Urquhart Shale for >10 km north of the limit of economic mineralisation and to a lesser distance to the south. This halo, the outer sections of which are characterised by fine-grained pyrite, strongly crosscuts stratigraphy, with a maximum development around the centre of the deposit, progressing to higher stratigraphic levels to the north (Perkins, 1998; Painter, 2003).
Individual bedding-parallel Pb-Zn-Ag sulphide orebodies are abruptly terminated at a range of scales, which to the north are generally at slight flexures within 20 m of the anticlinal hinge of the Mount lsa Fold (Perkins, 1997; Davis, 2004). For example, on the 11 level, grades in the 7 orebody on the western long limb of the fold diminish abruptly from 7.0 m @ 3.7% Pb, 8.2% Zn over a 45 m interval to 0.73% Pb, 0.24% Zn at the anticlinal hinge (Perkins, 1996). The Mount lsa Fold (described above) is an 'S' shaped anticline-syncline pair offset along their common limb by the axial plane parallel Urquhart Shear. This structure marks the northern limit of higher grade Zn-Pb-Ag mineralisation, although lower grade, Zn>Pb mineralisation redevelops to the north of the structure. Flexures and termination of mineralisation are also locally in close proximity to east-dipping D2 fractures and veins (Lilly et al., 2017). Davis (2004) showed that the extremities of the orebodies correlate with F4 folds, whilst high-grade shoots are centred on F4 hinges and short limbs that contain older F2 folds. Contours of Pb/Zn ratios throughout the orebodies are parallel to F4 hinges and silica-dolomite alteration fronts. F2 folds, which are preserved in the hinge zons and short limbs of F4 folds, are interpreted to have behaved as structural heterogeneities during D4, which caused the dilation that led to metal deposition. F4 folds closest to the copper orebodies contain the highest grade Zn-Pb-Ag ore shoots (Davis, 2004).
Perkins (1996) concluded that both bedding-parallel and cross-cutting structures controlling the localisation of mineralisation existed prior to the precipitation of sulphides. Fine-grained pyrite is locally overprinted by all other economic sulphides (Grondijis and Schouten, 1937). Sphalerite is overprinted by galena, and both are overprinted by chalcopyrite, which everywhere is paragenetically the youngest sulphide.
δ34S values range from 0 to 32‰ for all fine-grained pyrite,
irrespective of whether it occurs in the copper or lead-zinc orebodies (Solomon,1965; Smith et al., 1978; Andrew et al., 1989; Eldridge,
1991, pers.comm. reported in Perkins, 1998). By comparison, sulphides directly associated with the Cu and Zn-Pb mineralisation generally accepted to be of hydrothermal origin involving exotic sulphur, also show a broad spread of δ34S, ranging from 11 to 27‰ (Perkins, 1996), which includes sulphides from the Cu orebodies ranging from 8 to 25‰ (Long, 2010). Sulphides from basement rocks distal to mineralisation yield δ34S values of from 3 to 7‰, whilst those from the altered Eastern Creek Volcanics and quartzites of the Haslingden Group below the Paroo Fault, where juxtaposed with the Cu orebody within the silica-dolomite altered Urquhart Shale, yield values in the range 12.2 to 23.2‰ (Long, 2010). Painter et al. (1999) interpret the δ34S values for fine grained pyrite to be the result of high temperature (up to 180°C; Machel, 2001) thermochemical sulphate reduction of evaporitic sulphate (gypsum, anhydrite and barite) within the lower Urquhart Shale (e.g., Neudert, 1984) to produce authigenic carbonate and liberate H2S to react with organic material and iron to form pyrite. However, Perkins (1997) argues that while pseudomorphs recognised by Neudert (1984) were after evaporitic sulphates, the volume of pyrite deposited required more sulphur than would have been available, and that when the pyrite was deposited, the sulphates had already been replaced by other phases. Perkins (1997) concluded that the sulphur for pyrite deposition was introduced in an oxidised form simultaneously with the iron, and reduced by the carbonaceous seams and carbon lined cleavages. Similarly, he concluded that the occurrence of galena, sphalerite and pyrrhotite, and their late timing, also indicate that sulphur was introduced with the metals.
However, Wilde et al., (2006) describe a large body of coarse-grained, granoblastic and massive, commonly hematite stained, anhydrite, dolomite and barite that is transgressively developed within the Kennedy Siltstone. It dips vertically, parallel to the immediately adjacent Paroo Fault and is located near the northern end of the Cu ore system, adjacent to the Zn-Pb-Ag orebody 5. This 'anhydrite' body, which is up to 70 m thick and has an inferred strike length in excess of 500 m. It does not outcrop, although breccia bodies at surface in the Kennedy Siltstone may represent the residuum after the solution and removal of anhydrite. The contact between the anhydrite body and Kennedy Siltstone comprises an ~2 m thick breccia zone, in which hydrothermally altered siltstone fragments are set in an anhydrite-dolomite-barite matrix. The main body anhydrite-dolomite-barite contains coarse 5 to 10% pyrite throughout. Chalcopyrite is usually intimately associated with pyrite at contacts with anhydrite and Cu averages ~0.16 wt.% over the width of the body, with intervals of up to 1.1 wt.% over 2 m. The geometry, mineral relationships and textures observed led Wilde et al., (2006) to suggest chalcopyrite and anhydrite were coprecipitated, and that the anhydrite is hydrothermal, rather than recrystallised in situ evaporitic in origin. This body confirms that a substantial volume of sulphate was available as a sulphur source for thermochemical sulphate reduction at the time of sulphide formation in both the Cu and Zn-Pb-Ag orebodies, but that this was not necessarily derived from earlier deposited evaporitic sulphate.
Alteration of original carbonate-rich metasediments of the Urquhart Shale resulted in coarse bedding controlled recrystallisation occurring as variably continuous bands of dolomite parallel to bedding, but with cross-cutting channelways. This dolomitisation led to a decrease in volume and the progressive obliteration of bedding to almost massive coarse-grained dolomite aggregates adjacent to the Paroo Fault, extending, with declining intensity through the zone of Cu mineralisation into the hosts to the Zn-Pb-Ag orebodies. Subsequent to dolomite and silica crystallisation, the system appears to have undergone further brittle fracturing ± ductile shearing, overprinting the silica-dolomite and metasediment. This fracturing was most intense adjacent to the Paroo Fault, producing an intensely shattered crackle network parallel to the fault. Alteration away from the intense fracturing followed splays on the hanging wall of the Paroo Fault to where these structures converged with bedding. Dolomitisation decreased upwards and outwards into less intense, layer controlled brittle fractures in the finely bedded Urquhart Shale units, accompanied by minor related shearing. Fluid inclusions in quartz and dolomite from the dolomitic breccia revealed three fluids:
i). An early CaCl2-rich with ~25% salinity, interpreted to have been derived from the Eastern Creek Volcanics;
ii). Later low-salinity, with 10 to 20 mole.% CO2 fluid suggested to have originated from the Mount Isa Group sedimentary sequence. These fluids indicate temperatures of emplacement of between 350 and 270°C and pressures of 1500 to 700 bars (Heinrich et al., 1989).
iii). Subsequent, retrograde siliceous alteration superimposed on the dolomitic breccia contains NaCl-rich inclusions, declining with time, from 10 to 20, to 4 to 9% NaCl bracketing Cu mineralisation with homogenisation temperatures of 220 to 140°C (Heinrich et al., 1989; Kendrick et al., 2006). This latter fluid has C, O and H isotope values indicating an origin as an oxidised, evolved basinal brine, or an evaporite-derived metamorphic fluid. On the basis of noble gas ratios, together with halogen geochemistry, Kendrick et al., 2006) concluded that the Cu mineralisation was the result of the mixing of the two brine types at temperatures of between 200 and 250°C (Heinrich et al., 1989; Waring, 1990). On the basis of statistical interpretation of δ34S data for mineral pairs, Painter et al. (1999) estimated deposit-scale equilibrium between sphalerite and galena was indicated at temperature of 240 to 120°C.
Paragenetic studies and the commonality of sulphur isotopes suggest only one stage of ore-related sulphide is present within the Mount Isa Cu and Zn-Pb-Ag deposits and that they represent a single, zoned carbonate replacement system (e.g., Grondijs and Schouten, 1937; Perkins, 1997; Taylor, 2016; Lilly et al., 2017).
These and other lines of evidence led Perkins (1997) to interpret the Pb-Zn-Ag ores as having formed epigenetically during D4, late in the Isan deformation history, following lithification, peak metamorphism and the bulk of the ductile deformation, and began with shear-controlled carbonate alteration that was progressively replaced by sulphides. Extension on fractures at an angle to bedding was followed by dextral shearing during a phase of shortening that has produced buckles in thicker layers and tight folds in thin layers. The folded remnants are surrounded by gangue alteration and then by sulphides. The ore/alteration system is interpreted by Perkins (1996, 1997; Davis, 2004; Lilly et al., 2017) to have formed from hydrothermal solutions entering along the Paroo Fault ramp, which is underlain by the Eastern Creek Volcanics of Leichhardt Superbasin. These, most likely over-pressured fluids migrated outwards and upward to form an evolving alteration system during the formation of both the layer-parallel and cross-cutting steep cleavages, culminating in a relatively short-lived mineralising event that formed the zoned pyrite-zinc-lead-copper system.
Banded stratabound sulphides, predominantly brown sphalerite and lesser galena, within finely laminated Urquhart Shale from drill hole I722 ED1 at 7218 mN on the low grade zinc rich northern fringe of the Mount Isa Zinc deposit. Note the different populations of fine brown disseminated sphalerite bands and coarser sphalerite, galena and pyrite. Scale bar in cm with mm graduations. This and the images below are all from intervals of 1 to 2 m containing 10 to 12% Zn, 3 to 5% Pb, 50 to 80 g/t Ag. This 56°E dipping hole passed from barren Spear Siltstone into the Urquhart Shale at 170 m, ending at 300 m in the latter. The Urquhart Shale intersected within this hole averaged 3.2% Zn, 1.26% Pb, 25.7 g/t Ag over 130 m, including two higher grade intersections of 4.7% Zn, 1.7% Pb, 38.4 g/t Ag over 35.7 m and 3.9% Zn, 1.3% Pb, 28 g/t Ag over 36.8 m. Images by Mike Porter, April 2019, of core held at the Queensland Department of Natural Resources, Mines and Energy John Campbell Miles Drill Core Storage Facility at Mount Isa.
Banded and brecciated stratabound sulphides from drill hole I722 ED1.
Brecciated, stratabound and crosscutting sphalerite, galena and pyrite from drill hole I722 ED1.
Brecciated, stratabound and crosscutting sphalerite, galena and pyrite with calcite veining from drill hole I722 ED1.
Brecciated, stratabound and crosscutting sphalerite, galena and pyrite from drill hole I722 ED1 with strongly folded sediment band within the breccia ore. Note the brecciated sulphides passing into the crosscutting vein on the lower right, and the breccia band crosscutting bedding on both margins.
Reserves and Resources
Total economic Mineral Resources + past production at Mt Isa, mainly from the underground operations, amounted to more than 150 Mt @ 7% Zn, 6% Pb, 150 g/t Ag (Forrestal, 1990; McGoldrick and Large, 1998; Waring et al., 1998).
The underground reserves at the Mount Isa Zn-Pb deposit were exhausted in late 2005 and the mine placed on care and maintenance. Operations commenced at the Black Star open pit in 2004 to maintain supply to the existing concentrator.
Remaining Ore Reserves and Mineral Resources at the end of 2003 (Xstrata, 2003) were:
Mount Isa Zn-Pb-Ag underground
Proved reserves - 2.8 Mt @ 6.3% Zn, 5.5% Pb, 150 g/t Ag
Probable reserves - 3.3 Mt @ 5.4% Zn, 6.3% Pb, 180 g/t Ag
Measured resources - 10.0 Mt @ 7.0% Zn, 6.4% Pb, 170 g/t Ag
Indicated resources - 5.8 Mt @ 4.7% Zn, 8.2% Pb, 210 g/t Ag
Inferred resources - 1.6 Mt @ 5.7% Zn, 7.7% Pb, 190 g/t Ag
Mount Isa Zn-Pb-Ag open pit
Indicated resources - 37 Mt @ 3.9% Zn, 3.3% Pb, 77 g/t Ag
Inferred resources - 250 Mt @ 4.4% Zn, 3.2% Pb, 74 g/t Ag
JORC compliant remaining Ore Reserves and Mineral Resources (at June 2006) were as follows (X-Strata 2007):
Black Star open pit
Proved reserves - 17.9 Mt @ 4.9% Zn, 2.6% Pb, 52 g/t Ag
Probable reserves - 14.4 Mt @ 5.4% Zn, 3.8% Pb, 72 g/t Ag
Measured resources - 27.2 Mt @ 5.4% Zn, 3.4% Pb, 65 g/t Ag
Indicated resources - 4.3 Mt @ 4.8% Zn, 3.0% Pb, 60 g/t Ag
Inferred resources - 3 Mt @ 5% Zn, 4% Pb, 80 g/t Ag
Mt Isa open pit
Measured resources - 87.3 Mt @ 4.1% Zn, 3.5% Pb, 81 g/t Ag
Indicated resources - 69.4 Mt @ 3.9% Zn, 3.3% Pb, 81 g/t Ag
Inferred resources - 180 Mt @ 4% Zn, 2% Pb, 50 g/t Ag
JORC compliant remaining Ore Reserves and Mineral Resources (at 31 December, 2011) were as follows (X-Strata 2012):
Black Star open pit
Proved + probable reserves - 30.1 Mt @ 4.9% Zn, 3.2% Pb, 63 g/t Ag
Measured + indicated resources - 30.1 Mt @ 5.1% Zn, 3.3% Pb, 66 g/t Ag
Inferred resources - 4 Mt @ 4% Zn, 3% Pb, 50 g/t Ag
Mt Isa open pit
Measured + indicated resources - 201.8 Mt @ 3.7% Zn, 3.0% Pb, 70 g/t Ag
Inferred resources - 150 Mt @ 4% Zn, 3% Pb, 60 g/t Ag
The Black Star open pit was closed in 2016 after mining ~40 Mt of ore.
JORC compliant remaining Mineral Resources (at 31 December, 2018) were as follows (Glencore 2018):
Black Star open pit
Measured + indicated resources - 7.9 Mt @ 4.7% Zn, 4.4% Pb, 74 g/t Ag
Inferred resources - 7.3 Mt @ 2% Zn, 1% Pb, 19 g/t Ag
Black Star underground
Measured + indicated resources - 66 Mt @ 5.6% Zn, 2.7% Pb, 49 g/t Ag
Inferred resources - 92 Mt @ 5% Zn, 3% Pb, 62 g/t Ag
Mount Isa open pit
Measured + indicated resources - 107 Mt @ 3.8% Zn, 3.8% Pb, 79 g/t Ag
Inferred resources - 40.3 Mt @ 4% Zn, 3% Pb, 62 g/t Ag
NOTE: Mineral Resources are inclusive of Ore Reserves.
Both the Mt Isa and Black Star open pit resources are approximately 85% primary sulphides, with the balance being mixed sulphide and secondary oxide/carbonate mineralisation.
The most recent source geological information used to prepare this decription was dated: 2017.
Record last updated: 19/4/2019
This description is a summary from published sources, the chief of which are listed below. © Copyright Porter GeoConsultancy Pty Ltd. Unauthorised copying, reproduction, storage or dissemination prohibited.
Mt Isa Zinc
|
|
Bell, T.H. and Hickey, K.A., 1998 - Multiple deformations with successive subvertical and subhorizontal axial planes in the Mount Isa region: their impact on geometric development and significance for mineralization and exploration: in Econ. Geol. v.93, pp. 1369-1389.
|
Betts P G, Giles D, Lister G S 2003 - Tectonic environment of shale-hosted massive sulfide Pb-Zn-Ag deposits of Proterozoic northeastern Australia: in Econ. Geol. v98 pp 557-576
|
Betts, P.G., Giles, D., Mark, G., Lister, G.S., Goleby, B.R. and Ailleres, L., 2006 - Synthesis of the Proterozoic evolution of the Mt Isa Inlier: in Australian J. of Earth Sciences v.53, pp. 187-211.
|
Blaikie, T.N., Betts, P.G., Armit, R.J. and Ailleres, L., 2017 - The ca. 1740-1710 Ma Leichhardt Event: Inversion of a continental rift and revision of the tectonic evolution of the North Australian Craton: in Precambrian Research v.292, pp. 75-92
|
Chapman L H, 2004 - Geology and Mineralization Styles of the George Fisher Zn-Pb-Ag Deposit, Mount Isa, Australia: in Econ. Geol. v99 pp 233-255
|
Conaghan, E.L., Hannan, K.W. and Tolman, J. 2003 - Mount Isa Cu and Pb-Zn-Ag deposits, NW Queensland Australia: in Regolith Expression of Australian Ore Systems - A compilation of geochemical case histories and conceptual models CRC LEME online, http://crcleme.org.au/RegExpOre/MtIsa.pdf, 3p.
|
Davis, T.P., 2004 - Mine-Scale Structural Controls on the Mount Isa Zn-Pb-Ag and Cu Orebodies: in Econ. Geol. v.99, pp. 543-559.
|
Derrick G M 1996 - The geophysical approach to metallogeny of the Mt Isa Inlier - what sort of orebody do you want: in Proc The AusIMM Annual Conference, Perth, 24-28 March, 1996 The AusIMM, Melbourne pp 349-366
|
Finlow-Bates T, 1979 - Cyclicity in the lead-zinc-silver-bearing sediments at Mount Isa mine, Queensland, Australia, and rates of sulfide accumulation: in Econ. Geol. v74 pp 1408-1419
|
Forrestal P J 1990 - Mount Isa and Hilton silver-lead-zinc deposits: in Hughes FE (Ed.), 1990 Geology of the Mineral Deposits of Australia & Papua New Guinea The AusIMM, Melbourne Mono 14, v1 pp 927-934
|
Gibson, G.M., Hutton, L.J. and Holzschuh, J., 2017 - Basin inversion and supercontinent assembly as drivers of sediment-hosted Pb-Zn mineralization in the Mount Isa region, northern Australia: in Journal of the Geological Society v.174, pp. 773-786.
|
Gibson, G.M., Meixner, A.J., Withnall, I.W., Korsch, R.J., Hutton, L.J., Jones, L.E.A., Holzschuh, J., Costelloe, R.D., Henson, P.A. and Saygin, E., 2016 - Basin architecture and evolution in the Mount Isa mineral province, northern Australia: Constraints from deep seismic reflection profiling and implications for ore genesis: in Ore Geology Reviews v.76, pp. 414-441.
|
Gregory M J, Schaefer B F, Keays R R and Wilde A R, 2008 - Rhenium–osmium systematics of the Mount Isa copper orebody and the Eastern Creek Volcanics, Queensland, Australia: implications for ore genesis: in Mineralium Deposita v.43 pp. 553-573
|
Heinrich, C.A., Andrew, A.S., Wilkins, R.W.T. Patterson D J 1989 - A fluid inclusion and stable isotope study of synmetamorphic Copper ore formation at Mount Isa, Australia: in Econ. Geol. v.84, pp. 529-550.
|
Huston, D.L., Champion, D.C., Czarnota, K., Duan, J., Hutchens, M., Paradis, S., Hoggard, M., Ware, B., Gibson, G.M. Doublier, M.P., Kelley, K., McCafferty, A., Hayward, N., Richards, F., Tessalina, S. and Carr, G., 2023 - Zinc on the edge - isotopic and geophysical evidence that cratonic edges control world-class shale-hosted zinc-lead deposits: in Mineralium Deposita v.58, pp. 707-729.
|
Leach D L, Bradley D C, Huston D, Pisarevsky S A, Taylor R D and Gardoll S J, 2010 - Sediment-Hosted Lead-Zinc Deposits in Earth History : in Econ. Geol. v.105 pp. 593-625
|
Lilly, R., Taylor, D. and Spanswick, N. 2017 - Mount Isa Cu-Pb-Zn deposit including George Fisher: in Phillips, G.N., 2017 Australian Ore Deposits, The Australasian Institute of Mining and Metallurgy, Mono 32, pp. 473-478.
|
Mathias B V, Clark G J 1975 - Mount Isa copper and silver-lead-zinc orebodies - Isa and Hilton mines: in Knight C L, (Ed.), 1975 Economic Geology of Australia & Papua New Guinea The AusIMM, Melbourne Mono 5 pp 351-372
|
McGoldrick P J, Keays R R 1990 - Mount Isa Copper and Lead-Zinc-Silver ores: coincidence or cogenesis?: in Econ. Geol. v85 pp 641-650
|
McLellan, J.G., O Sullivan, R., Miller, B. and Taylor, D., 2014 - Geomechanical Modelling of the Mount Isa Copper Deposit - Predicting Mineralisation: in Ninth International Mining Geology Conference, Adelaide, S.A., 18-20 August, 2014, Mining geology through the value chain, The AusIMM and Australian Institute of Geoscientists, Proceedings, pp. 197-205.
|
Page R W 1981 - Depositional ages of the stratiform base metal deposits at Mount Isa and McArthur River, Australia, based on U-Pb Zircon dating of concordant tuff horizons: in Econ. Geol. v76 pp 648-658
|
Page R W, Conor C H H, Stevens B P J, Gibson G M, Preiss W V and Southgate P N 2005 - Correlation of Olary and Broken Hill Domanins, Curnamona Province, Australia: Possible Relationship to Mount Isa and Other North Australian Pb-Zn-Ag-Bearing Successions: in Econ. Geol. v100 pp 663-676
|
Painter, M.G.M., Golding, S.D., Hannan, K.W. and Neudert, M.K., 1999 - Sedimentologic, petrographic, and Sulfur isotope constraints on fine-grained pyrite formation at Mount Isa mine and environs, northwest Queensland, Australia: in Econ. Geol. v.94, pp. 883-912.
|
Perkins W G 1998 - Timing of formation of Proterozoic stratiform fine-grained Pyrite: Post-diagenetic cleavage replacement at Mount Isa?: in Econ. Geol. v93 pp 1153-1164
|
Perkins W G, Bell T H 1998 - Stratiform replacement lead-zinc deposits: a comparison between Mt Isa, Hilton and McArthur River: in Econ. Geol. v93 pp 1190-1212
|
Perkins, W.G., 1997 - Mount Isa lead-zinc orebodies: Replacement lodes in a zoned syndeformational copper-lead-zinc system?: in Ore Geology Reviews v.12, pp. 61-110.
|
Polito, P.A., Kyser, T.K., Southgate, P.N. and Jackson, M.J., 2006 - Sandstone Diagenesis in the Mount Isa Basin: An Isotopic and Fluid Inclusion Perspective in Relation to District-Wide Zn, Pb, and Cu Mineralization: in Econ. Geol. v.101, pp. 1159-1188.
|
Smith W D 1975 - Black Rock secondary copper orebody - Mount Isa, Q: in Knight C L, (Ed.), 1975 Economic Geology of Australia & Papua New Guinea The AusIMM, Melbourne Mono 5 pp 1033-1039
|
Williams P J 1998 - An introduction to the metallogeny of the McArthur River-Mt Isa-Cloncurry Minerals Province: in Econ. Geol. v93 pp 1120-1131
|
Porter GeoConsultancy Pty Ltd (PorterGeo) provides access to this database at no charge. It is largely based on scientific papers and reports in the public domain, and was current when the sources consulted were published. While PorterGeo endeavour to ensure the information was accurate at the time of compilation and subsequent updating, PorterGeo, its employees and servants: i). do not warrant, or make any representation regarding the use, or results of the use of the information contained herein as to its correctness, accuracy, currency, or otherwise; and ii). expressly disclaim all liability or responsibility to any person using the information or conclusions contained herein.
|
Top | Search Again | PGC Home | Terms & Conditions
|
|