Prominent Hill |
|
South Australia, SA, Australia |
Main commodities:
Cu Au
|
|
 |
|
 |
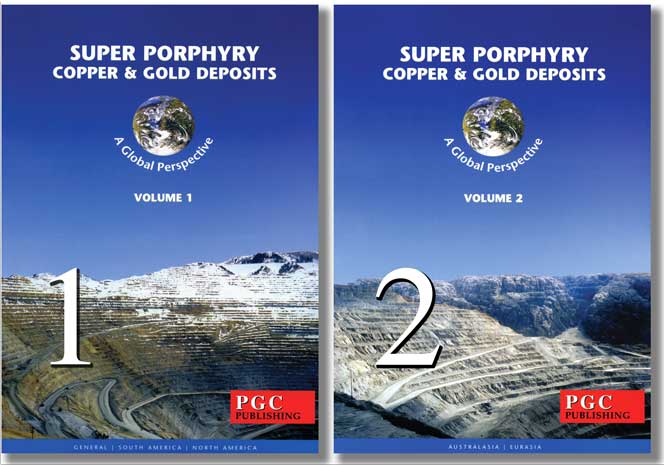 |
Super Porphyry Cu and Au

|
IOCG Deposits - 70 papers
|
All papers now Open Access.
Available as Full Text for direct download or on request. |
|
 |
The Prominent Hill iron oxide copper-gold (IOCG) deposit is located approximately 150 km north-west of Olympic Dam and 650 km NNW of Adelaide in northern South Australia (#Location: 29° 43' 14"S, 135° 34' 38"E).
Prominent Hill, Carrapateena, Olympic Dam, Moonta-Wallaroo and Hillside, and all of the other significant known IOCG mineralised systems of the Gawler craton, are hosted within Palaeo- to Mesoproterozoic rocks and are distributed along the eastern edge of the currently preserved craton, to define the Olympic IOCG Province.
Discovery and History
The Prominent Hill IOCG deposit was discovered through diamond drilling of a gravity anomaly in 2001 (Carter et al., 2003) and was brought into production in 2009.
Copper-gold mineralisation associated with magnetite alteration had been discovered in the Mount Woods region by earlier explorers in the 1980s (e.g., the Manxman, Joes Dam prospects), although none were considered economic (Skirrow et al., 2002). Minotaur Exploration Ltd. explored the area based on the model that hematite-dominated iron oxide systems were also likely to be present near the magnetite systems, either at shallower structural levels, or in laterally adjacent positions. Geophysical modelling of gravity and magnetic anomalies contributed significantly to the discovery (Hart and Freeman, 2003), but because of the cover, extensive drilling was required to delineate targets. After drilling other tagets in the Mount Woods area, the discovery hole, URN001, was drilled in October 2001 to test a discrete gravity anomaly, located to the south of an intense magnetic anomaly. This vertical hole passed through 108 m of barren cover sediments before intersecting a mineralised massive hematite-supported breccia. The hematite breccia averaged 1.94% Cu and 0.66 g/t Au over 107 m, from 200 m depth. Deepening of URN001 intersected a further 152 m averaging 1.20% Cu and 0.61 g/t Au from 429 m, also hosted in a haematite breccia. This same interval averaged 0.57% REE and 495 ppm U. See the Prominent Hill section below for detail of what the gravity and magnetic anomalies reflected and their relationship to mineralisation.
The drilling at Prominent Hill was funded by BHP Billiton that was earning a 51% interest in the existing Mt Woods copper-gold joint venture. The other partners in that joint venture with Minotaur, which was diluting to 19% share, were Newmont (23.94%), Sons of Gwalia (3.78%) and Sabatica (2.28%).
However, in 2003, BHP Billiton decided Prominent Hill would not reach BHP Billiton's significant size threshold and agreed to sell its 51% interest to Minotaur. This transaction saw Minotaur acquire 100% of the Mount Woods Joint Venture; and in recompense, Newmont gain a 13.9% interest in Minotaur's undiluted share capital along with Sons of Gwalia (2.2%) and Sabatica (1.3%). Minotaur then introduced Oxiana Limited as a partner with the right to earn a 65% interest in the new Prominent Hill Joint Venture. In 2004, Oxiana acquired Minotaurs share of the joint venture.
Oxiana board approval for the Prominent Hill operation to proceed was granted in 2006, and the company commenced production in February 2009.
In 2008, Oxiana Limited and Zinifex Limited merged and rebranded to become OZ Minerals Limited. In February 2009 a takeover offer by China Minmetals was recommended for acceptance by the OZ Minerals board to form MMG Limited. However, in March 2009 the Government of Australia blocked the sale, citing national security concerns about the proximity of the Prominent Hill Mine to the RAAF Woomera Range Complex. A revised deal that excluded Prominent Hill was approved in April 2009. This saw Prominent Hill remain as the only asset of OZ Minerals. In 2024, BHP Group Limited acquired 100% of OZ Minerals and the Prominent Hill deposit.
Cratonic to Domain-scale Setting
See the Gawler Craton and Olympic IOCGU Province record for a summary of the regional, cratonic setting of the Olympic IOCG Province.
Prominent Hill lies on the southern margin of the Mount Woods Domain (MWD), which is located within the Olympic IOCG Province in the northeast of the preserved Archaean to Mesoproterozoic Gawler Craton. The MWD comprises Palaeo- and Mesoproterozoic metamorphic and igneous rocks that have a well defined aeromagnetic signature. It is characterised by high magnetic and gravity signatures, caused by multiple iron-oxide and mafic rock sources, and its boundaries are sharp and structurally controlled. The MWD encloses a major regional ~75 x 50 km magnetic complex with an overall 'U'-shape, elongated in a NW-SE direction and open to the SE. Outcrop is sparse, with flat lying Phanerozoic cover reaching thicknesses of up to 400 m in places, although it is generally <200 m.
The MWD comprises at least two separate sedimentary successions that have been subjected to one or more amphibolite to granulite facies metamorphic events, three periods of deformation, and two (probably three) episodes of magmatism, as well as a pulse of Neoproterozoic mafic dyke intrusion. Nine basement sub-domains have been defined, within the southern half of the domain within a 40 km radius of Prominent Hill.
The core of the southern half of the MWD is occupied by an 'eye-shaped', east-west elongated, 25 x 10 km kernel of magnetically anomalous rocks, reflecting the lopolithic White Hill Igneous Complex, which is characterised by extremely high amplitude magnetic lineaments on its margins, more subtle concentric magnetic zoning in its centre, and a very pronounced and complex gravity signature. The complex (where drilled) comprises pyroxenite, norite and gabbro, with pronounced layering defined by plagioclase and pyroxene-rich layers, with interleaved disseminated to massive magnetite-ilmenite bands. The gabbros and pyroxenites are hydrous, alkaline and enriched in volatile components, and carry up to 8% modal apatite. The complex is divided by a north-south fault into the White Hill and Joe's Dam sub-domains to the east and west respectively, based on differences in the magnetic pattern. The White Hill Complex is not as well developed in the Joe's Dam Sub-domain. Where drilled, the latter comprises a suite of quartzo-feldspathic gneisses with magnetite rich gneiss intercalations, and rare bands of mafic rocks, supporting the interpretation that the White Hill Igneous Complex is not well represented to the west. The magnetite and hematite breccias and replacement bodies of the Joe's Dam sub-domain are hosted by psammitic, pelitic and gneissic metasediments of higher metamorphic grade than those in the marginal Neptune and Blue Duck sub-domains. The voluminous Hiltaba Suite magmatism (Hiltaba granites and mafic to ultramafic White Hill Igneous Complex) in the area are a potential cause of high temperature contact metamorphism of the pelites (Freeman and Tomkinson, 2010).
The lenses-shaped 20 x 5 km Kennedy's Dam Sub-domain forms much of the northern margin of the White Hill and Joe's Dam sub-domains, and is characterised by relatively
low magnetic susceptibility enclosing a few moderate to high amplitude, linear magnetic anomalies. Where drilled, it is composed of a sequence of quartz-feldspar-biotite-magnetite gneisses and 'granulites' with very minor amphibolite and calc-silicate units, intruded locally by coarse grained granite and pegmatite.
The Skylark Sub-domain bounds the Kennedy's Dam Sub-domain to the NW, and is characterised by large intrusions with low to moderate magnetic responses, surrounded by narrow aureoles of higher magnetic intensity related to either hornfelsing or magnetite metasomatism. Granite with actinolite±magnetite veins and pyroxenites, norites and diorites dated at 1587±4 Ma (U-Pb; Jagodzinski, 2005) have been encountered in drill holes, intruding a variety of quartz-feldspar-biotite gneisses, all with accessory magnetite. Metasediments away from the intrusions include quartz-rich meta-sandstone with plagioclase, opaque oxide (magnetite and hematite), schistose biotite, fluorite and tourmaline. Samples of cordierite-garnet-bearing pelite and magnetite psammite have maximum depositional ages of ~1750 Ma respectively ( U-Pb zircon; Jagodzinski et al. 2007), and were intruded by the syn-metamorphic Engenina Adamellite (~1691±25 Ma; Finlay 1993; Daly et al. 1998). Geophysical data suggest the domain may be underlain by a continuation of the White Hill Igneous Complex.
Tectonic and geophysical setting of iron oxide related mineralisation in the southern Mount Woods Domain (MWD) -
a). RTP aeromagnetic image of the southern MWD showing the sub-domains discussed herein. The sub-domains are based
on exploration drilling and geophysical data; b). The interpreted sub-domains of the southern MWD and location of prospects
discussed. The prospects correspond to magnetic and/or gravity anomalies. Modified after: Freeman and Tomkinson (2010); Schlegel et al. (2018).
The 50 x 5 km Taurus Sub-domain forms the NE margin the Kennedy's Dam Sub-domain, but persists further to the east, delineating the northern margins of the White Hill and then the Blue Duck sub-domains, and the southern margin of the Ware's Peak Sub-domain to the NE. This terrane, characterised by a series of short strike length curvilinear magnetic features by major discontinuities, is interpreted to represent rocks caught up between a major strike-slip shear couple.
The extensive Ware's Peak Sub-domain occupies a large area in the NE of the MWD and is characterised by a few high amplitude, strike continuous, but folded magnetic linears, and by large areas of diffuse magnetic signature suggesting moderately magnetic and eastward deepening younger cover. Drill holes testing prospects encountered a highly variable suite of magnetite, plagioclase and garnet bearing paragneisses (including graphite rich units), as well as feldspathic and quartz-poor to quartz-rich meta-igneous lithologies (including quartz diorite, monzodiorite, syenite, granite and pegmatite). Other lithologies include metasandstone, iron formation, calc-silicate, skarn, dolomite and marble units. Undeformed granite and gabbroic intrusions have also been identified in drilling. Metamorphic grades range from mid-amphibolite to granulite facies.
The curvilinear, lensoid 2 to 6 km wide and >60 km long Blue Duck Sub-domain extends along the southern margin of the Joe's Dam, White Hill, Taurus and Ware's Peak sub-domains. In the west, it forms the southernmost sub-domain of the MWD. It is thickest in the centre, where it is immediately to the north of the Neptune Sub-domain that contains the Prominent Hill deposit near the contact between the two terranes, and thins on either extremity. In the east, its southern margin is marked by the regional ENE-trending Bulgunnia Fault. It is characterised by narrow, medium amplitude magnetic linears which are continuous for several kilometres or more. Drilling at a number of locations (mostly aimed at magnetic targets), has encountered metamorphosed calcsilicate units with associated magnetite, schistose, recrystallised K feldspar-biotite-chlorite-quartz-scapolite clastic metasediments, iron-rich, pelitic carbonate rock, marble, calc-silicate, magnetite-pyroxene-quartz rock and possible meta-evaporites. These rocks have been tentatively correlated with the 1760 to 1740 Ma Wallaroo Group found elsewhere in the Olympic IOCG Province (Freeman and Tomkinson, 2010).
The elongate Neptune Sub-domain forms the southern boundary of the MWD over an interval of ~30 km, where it fringes part of the southern Blue Duck Sub-domain, separated by the major, south vergent, Southern Overthrust. It has an overall 'V' shape, turning on its eastern extremity from an east-west to WSW trend, along the northern side of the regional Bulgunnia Fault. It includes the , which are up to 4.5 km thick (tentatively correlated on lithological grounds with the ~1590 Ma Gawler Range Volcanics) and the Prominent Hill Mine Sequence. Compared to terrains further to the north, it is characterised by relatively low amplitude linear aeromagnetic anomalies and contains a sequence of lower greenschist facies, relatively undeformed, mafic to felsic volcanic rocks (basalt-andesite-dacite-rhyolite), hematite-cemented quartz conglomerate, sandstone, argillite and dolostone. This sequence coarsens southwards from argillaceous and calcareous rocks into coarse grained siliciclastic rocks. The volcanic component in the structural footwall to the Prominent Hill deposit are basaltic to andesitic in composition, commonly porphyritic and amygdaloidal. Several kilometres to the west, felsic volcanic rocks (dacite to rhyolite) become much more voluminous with 'redrock' hematite dusting of alkali feldspar, compared to the predominantly sericite-chlorite-earthy hematite-leucoxene-carbonate alteration within the basalts and andesites of the mine sequence. Fragmental lithologies are intercalated with the coherent footwall volcanic rocks at Prominent Hill, and include agglomerate, felsic tuff or ignimbrite (Belperio et al., 2006) and volcanic clast conglomerate. They are also intercalated with mafic to intermediate volcanic rocks and hematitic, quartz-feldspar conglomerate and interbedded coarse grained sandstone. The east-west-trending Hanging wall Fault at Prominent Hill has been inferred to separate the hematite-stable sedimentary rocks of the copper-gold mineralised host sequence of the Neptune Sub-domain in the south, from a magnetite-stable hanging wall sequence of chloritic pelite and pelitic carbonate rock of the Blue Duck Sub-domain to the north. Proterozoic basement is overlain by 90 to 150 m of flat-lying Permo-Carboniferous sandstone and diamictite and Cretaceous sandstone and black claystone.
The Danae Hill Sub-domain forms the southern margin of the MWD to the southeast of the regional Bulgunnia Fault. It is characterised by a similar magnetic signature to the Neptune Volcanics, with low to moderate amplitude aeromagnetic linears. Drilling has identified a suite of altered, low-grade metamorphosed, sheared and brecciated basalts, with lesser metasediments and acid volcanic rocks, which collectively suggest a bimodal volcanic suite. These volcanics have a markedly different trace element signature similar to that of the Neptune Volcanics and are inferred to be of late Neoarchaean to Palaeoproterozoic in age.
The Christie Domain, is mainly found to the immediate south of the of, but also includes the MWD Neptune/Blue Duck and Danae sub-domains, and is predominantly composed by latest Archaean to earliest Palaeoproterozoic metasedimentary protoliths, metamorphosed in the earliest Palaeoproterozoic at ~2450 Ma during the Sleaford Orogeny to become the Christie Gneiss of the Mulgathing Complex. The MWD and has been overthrust onto the Christies Domain from the NE, with a major north dipping thrust, the Southern Overthrust marking the boundary (Betts et al., 2003).
In summary, the MWD is predominantly composed of ~1760 to ~1740 Ma Palaeoproterozoic meta-sedimentary and meta-volcanic rocks, tentatively correlated with the Wallaro Group seen elsewhere in the Olympic IOCG Province, including banded iron formations (possibly equivalent to the Middleback Range BIFs further to the south near Whyalla). These successions, which may include inliers of older late Neoarchaean to lower Palaeoproterozoic metamorphic rocks, underwent peak metamorphism at ~1736±14 Ma to amphibolite and granulite facies. They are bounded on the southern margin of the domain, across the south-vergent Southern Overthrust, by younger, low- to mid-greenschist facies, ~1590 Gawler Range Volcanics equivalents and intercalated volcanic and sedimentay breccias of the Neptune Sub-domain, hosting the Prominent Hill ore deposit. All of these rocks have been intruded by the ~1691±25 Ma Engenina Adamellite, the extensive Hiltaba Suite 1584±18 Ma Balta Granite and mafic to ultramafic rocks that include the 1562 ±14 Ma (U-Pb zircon; Allen et al., 2016) White Hill Igneous Complex and similar 1587±3 Ma (U-Pb zircon; Jagodzinski, 2005) leucogabbro near Peculiar Knob.
The presence of Hiltaba-aged zircon, interpreted as metamorphic (Holm, OZCHRON), in quartzites and felsic gneisses suggests that metamorphic grade in the MWD at ~1590 to ~1580 Ma was significantly higher than is typical elsewhere in the Gawler Craton during that period. This, in turn, suggests that the MWD was at deeper crustal levels than the adjacent Olympic, Christie and Wilgena Domains.
These differences in crustal level may be explained by Tiddy et al. (2020) who have interpreted the regional structural architecture of the Mount Woods Domain (MWD) to represent a partially preserved metamorphic core complex that developed during the ~1600 to 1580 Ma Hiltaba Event. The lower plate of the complex is preserved in the central to northern parts of the MWD, bounded to the SW, east and NE by the curved Skylark Fault Zone, and to the north and NW by Cairn Shear Zone and the Panorama Fault Zon to the west. The rocks of this plate comprise the Mount Woods Metamorphics which have a maximum detrital zircon depositional age of ~1860 Ma and were polydeformed and metamorphosed to upper amphibolite to granulite facies during the ~1740 to 1690 Ma Kimban Orogeny. These metamorphic rocks cover a WNW-ESE elongated area of ~60 x 30 km. To the south, the upper plate is composed of a younger succession, which includes the Skylark Metasediments that were deposited at ~1750 Ma. Subsequently, sedimentary and volcanic successions of the Gawler Range Volcanics were deposited into half grabens that evolved during brittle normal faulting of the upper plate caused by the updoming that resulted from the rise of the lower plate. This upper plate brittle deformation is underlain by the Skylark Shear Zone which represents the basal detachment fault separating the upper and lower plate of the core complex. The geometry of normal faulting in the upper plate is consistent with NE-SW extension. Both plates were intruded by ~1795 to 1575 Ma Hiltaba Suite granitic and mafic plutons. These intrusions and half graben hosted Gawler Range Volcanics include the White Hill intrusions and sequences of the Joe's Dam, Kennedy and Blue Duck domains to the SE of the Skylark Fault Zone. Extensive modification of the core complex occurred during the ~1570 to 1540 Ma Kararan Orogeny which is characterised by shear zone formation. Exhumation of the complex was associated with activity along syn-Kararan Orogeny faults, as indicated by 40Ar/39Ar biotite cooling ages that show that rock packages in the central MWD cooled to below ~300 ±50°C at ~1560 Ma, which was ~20 m.y. before equivalent cooling in the western and eastern Mount Woods Domain. This assumes all three packages were metamorphosed at the same time. The western MWD part of the upper plate had reached peak metamorphic temperatures of ~750°C at ~1590 Ma (Forbes et al., 2011). Exhumation had commenced along the Southern Overthrust at ~1592 to 1582 Ma (Forbes et al., 2012), and was likely further accommodated by Kararan-aged faults. Exhumation continued until ~1540 Ma, likely accommodated by the Southern Overthrust and possibly by continued activity along the Skylark and Panorama Fault Zones (Tiddy et al., 2020). These observation suggest the Hiltaba Suite intrusives and Gawler Range Volcanics were emplaced under a NE-SW directed extensional regime, rather than NW-SE directed transpression.
Regional Alteration and Mineralisation
Two main iron oxide alteration styles are abundant in the southern Mount Woods and northern Christie domains:
• Magnetite-dominant alteration which generally comprises repeated episodes of magnetite ±phlogopite and/or magnetite-K feldspar-pyrite ±chalcopyrite ±pyrrhotite ±apatite ±titanite ±monazite which overprint both metasedimentary and volcanic rocks, and occurred both during and after brecciation. These alteration assemblages are locally accompanied by low grade Cu, mainly due to minor associated chalcopyrite (Schlegel et al., 2015).
Hydrothermal replacement, veins and breccia-fills of (already brecciated) pelitic and calcareous metasediments and gneisses are abundant along the southern and eastern margins of the White Hill Igneous Complex (Freeman and Tomkinson, 2010; Schlegel and Heinrich, 2015). Proximal to the same igneous complex (see images above for location), the metasedimentary rocks are altered to an assemblage that includes magnetite (with minor hematite) ±biotite/phlogopite ±pyrite ±pyrrhotite ±chalcopyrite ±diopside ±actinolite ±talc ±scapolite (Schlegel et al., 2017). A number of large magnetite accumulations with anomalous copper, gold and uranium associated with this alteration are found adjacent to this intrusive complex, and within 10 km to the west in the Joe's Dam sub-domain, often with associated mafic to ultramafic intrusions - see the Manxman - Joes Dam record for a detailed description. These occurrences are interpreted to have developed by contact metamorphism and the release of magmatic-hydrothermal fluid from the White Hill Igneous Complex (Betts et al., 2003; Freeman and Tomkinson, 2010; Schlegel and Heinrich, 2015). On the basis of textural and paragenetic observations (Schlegel and Heinrich, 2015), this regional magnetite-'skarn' alteration and mineralisation may partly pre-date the economic Cu-Au ore formation at Prominent Hill. Further south and distal from the intrusion, weakly magnetite or hematite altered amphibolite facies calcareous and pelitic (meta-) sediments contain abundant pyrite. The fluids involved in this regional alteration may well have resulted from mingling of the mafic to ultramafic, mantle derived igneous complex and felsic gneisses and/or Balta granites of the Joe's Dam sub-domain to initiate exsolution and expulsion of Fe-rich hypersaline (Na-Ca-K) fluids responsible for the regional alteration and mineralisation observed in the southern Mount Woods and northern Christie domains (e.g., Chen et al., 2010; Velasco and Tornos, 2009).
At the Joe's Dam South East prospect, calcareous beds within a sequence of interbedded limestone-pelite and its brecciated equivalents are pervasively replaced by a magnetite ±pyrite ±quartz assemblage (Betts et al., 2003). This alteration took place in stages, separated by brecciation and renewed replacement of the calcareous breccia matrix by magnetite and pyrite. The prospect lies immediately adjacent to the margin of the White Hill Igneous Complex. Magmatic magnetite banding is found in gabbros on the margin of the igneous complex, whilst miarolitic cavities in gabbro, also on the margins of the intrusion, contain K feldspar-phlogopite-magnetite-quartz ±pyrite ±pyrrhotite. Within the sedimentary wall rock, K feldspar-, quartz- and pyrite-bearing pegmatitic veins cut the deformed interbedded limestone-pelite unit which also contain pyrite in calcareous layers that grade into dominantly magnetite-pyrite ±phlogopite-altered limestone interbedded with pelite. The magnetite alteration grades outward into magnetite-poor but pyrite-rich brecciated calcareous beds within the limestone-pelite sequence (Schlegel et al., 2015; 2017).
• Hematite-dominant alteration overprints undeformed albite- and magnetite-K feldspar altered porphyritic to aphyric basaltic andesitic and andesitic extrusives of the 'Neptune Volcanics', interpreted to be equivalents of Gawler Range Volcanics (Carter et al., 2003; Harris et al., 2013) at the Neptune and Triton prospects, 5 to 6 km SE of Prominent Hill. At Triton, these mafic lavas are variously altered to hematite-chlorite-sericite ±quartz ±kaolinite ±fluorite ±apatite ±barite ±leucoxene, surrounding intense hematite-quartz-apatite-barite alteration (Schlegel and Heinrich, 2015). Early crystallised amphibole is replaced by chlorite, and is overgrown by euhedral plagioclase phenocrysts, that in turn, are progressively altered to sericite and chlorite. The transition to the inner hematite-quartz alteration resulted in pseudobrecciation and near total replacement and destruction of mineral textures in the original protolith. Kaolinite is intergrown with sericite and replaces plagioclase crystals or fills volcanic amygdales. Intense hematite-quartz ±apatite ±barite alteration resulted in replacement of feldspars and sericite-kaolinite-filled amygdales, whilst late calcite fills the remaining pores. Local patches of chalcopyrite and minor chalcocite are found in intensely altered autobrecciated intervals between volcanic flows, and in veins containing hematite, euhedral quartz, K feldspar and pyrite. Veins with finely intergrown quartz-hematite-chalcopyrite-pyrite dominate and K feldspar or sericite occur as vein selvages or in the matrix of local breccias. At ~250 m depths, these veins become increasingly brecciated to form a fracture network that hosts Cu mineralised clasts set in a matrix with two generations of chalcopyrite-pyrite that has associated fluorite, barite and dolomite, indicating that mineralisation is related to small, multiple brecciation events. Degassing of volatiles from the lavas at Triton resulted in vesicular flow tops filled with quartz-hematite-magnetite-fluorite-chalcopyrite and late dolomite (Schlegel et al., 2017).
At Neptune, mafic lavas of the 'Neptune Volcanics' are altered to a magnetite ±K feldspar, chlorite ±fluorite ±apatite ±leucoxene ±barite ±carbonate ±pyrite ±chalcopyrite assemblage that are pervasively altered by magnetite-quartz-pyrite-chalcopyrite ±K feldspar ±calcite ±fluorite. Potassic feldspar is commonly reddened by very fine grained hematite. Four main generations of secondary iron oxides are distinguished at Neptune: i). fine granular magnetite, enriched in basaltic protoliths; ii). coarse granular magnetite, mostly associated with late hydrothermal 'mineralisation', some partly converted to hematite; iii). 'fresh', randomly clustered, bladed hematite; and iv). magnetite, partly to completely replacing earlier, randomly clustered, bladed hematite (mushketovite). Broad intervals of strong magnetite alteration contain anomalous to low grade copper mineralisation with minor zones of higher grade restricted to narrow, matrix-supported, magnetite rich hydrothermal breccias.
PROMINENT HILL DEPOSIT
The Prominent Hill deposit was discovered under ~100 m of cover, and is reflected by a discrete gravity anomaly, the target of the discovery drillhole (Carter et al. 2003). This anomaly corresponds to the hydrothermal iron altered (magnetite-deficient) hematite matrix breccias that host the copper-gold-silver-uranium-cerium-lanthanum ore deposit within the Neptune Sub-domain, and to corresponding palaeotopographic highs. The peak of the gravity anomaly coincides with a mass of massive, barren 'steely' hematite-silica flooded volcanics (on the eastern end of the main ore zone), flanked to the west by a ~2 km long mineralised hematite matrix-supported breccia. The overall gravity anomaly has an east pointing "V" form, with a 1 km long northern arm trending WNW-ESE, and the southern 2 km long limb trending WSW-ENE. The southern limb reflects the main mineralised hematite breccia, with the peak of the anomaly near the hinge, and has no magnetic expression (Belperio et al., 2006).
An associated 750 m long magnetic anomaly coincides with the northern limb of the gravity feature. It is centred ~500 m to the north of the orebody, across the steeply north dipping Hanging wall Fault that separates the ore hosting rocks of the Neptune Sub-domain in the south, from the Blue Duck Sub-domain to the north. This magnetic body, which is within the Blue Duck Sub-domain (Hart and Freeman 2003), reflects a package of magnetite-chlorite-tremolite-phlogopite altered metasomatic 'skarn-like' altered calc-silicate/carbonate-rich metamorphic rocks, intercalated with highly altered, intermediate porphyritic intrusives and chlorite matrix tectonic breccias (Belperio et al., 2006). This package largely comprises a body of massive magnetite accompanied by phlogopite-chlorite-pyrite ±chalcopyrite ±actinolite-tremolite ±serpentine ±talc ±scapolite, known as the 'magnetite skarn' (Belperio et al., 2007; Freeman and Tomkinson, 2010; Schlegel and Heinrich, 2015).
The deposit is characterised by abundant, largely pre-mineralisation breccias, large volumes of hematite-white mica-carbonate altered rock, variation of the dominant Cu mineral species (native copper/ chalcocite/bornite/chalcopyrite), and a distinctive geochemical association with marked enrichment of F, Ba, LREE and U (Belperio et al., 2007; Williams et al., 2017).
Geology
The Hanging wall Fault is a relatively brittle, sinistral structure that dips at 70 to 80°N in the deposit area, but flattens to 60 to 60° a little further west. It is represented by a chlorite breccia zone (Freeman and Tomkinson, 2010; Schlegel and Heinrich, 2015). To the immediate north of the main Prominent Hill deposit, it closely follows the major, south vergent Southern Overthrust. The latter is a domain boundary separating a mid- to upper-greenschist to locally amphibolite facies sequence of calcsilicate and phyllite to the north in the Blue Duck Sub-domain, correlated with the Palaeoproterozoic Wallaroo Group, from the less metamorphosed volcanosedimentary succession of the Neptune sub-domain to the south. To the west of the main Prominent Hill deposit, the Southern Overthrust diverges sharply northward whilst the Hanging wall Fault continues with an east-west trend. The Neptune sub-domain sequence hosts the Prominent Hill deposit in the footwall of the Hanging wall Fault/Southern Overthrust, within a 200 to 300 m thick, <1.75 Ga sequence of weakly to unmetamorphosed shallow marine grading to fluvial carbonate and siliciclastic sedimentary rocks, including conglomerates and sedimentary breccias. This sequence is overturned, dipping steeply to the north, subparallel to the Hanging wall Fault. The Main Host Sequence is structurally underlain, but stratigraphically overlain, by a younger, ~1.59 Ma sequence dominated by basalt, basaltic andesite and andesite, correlated with the Gawler Range Volcanics and then by a red bed sequence. All of these rocks are cut by two generations of mafic dykes (Freeman and Tomkinson, 2010; Williams et al., 2017).
The succession in the Prominent Hill deposit area is a follows, from oldest to youngest (after Freeman and Tomkinson, 2010 and Schlegel and Heinrich, 2015):
Hanging wall metamorphic sequence above the Southern Overthrust.
• Mostly unbrecciated metasedimentary rocks that include carbonate- and chloritic pelite/phyllite and K feldspar-biotite-chlorite-quartz-scapolite clastic metasedimentary rocks, limestone and dolostone, and inferred former evaporites (Freeman and Tomkinson, 2010). They are intruded by undeformed reddish granitoids, mainly quartz-diorite, tonalite and a felsic K feldspar-phyric porphyry which has fine-grained hematite dusting. The intrusions also include a ~1585 Ma dacite porphyry (Belperio et al., 2007). This sequence, which is interpreted to belong to the 1765±27 to 1741±1 Ma (GeoScience Australia) Wallaroo Group, is the host to the 'magnetite skarn'.
- Southern Overthrust and in part Hanging wall Fault and unconformity
Main Host Sequence in the footwall of the Hanging wall Fault/Southern Overthrust, sandwiched between the hanging wall metamorphic and footwall volcanic sequences. These rocks have a maximum depositional age of ~1750 Ma (detrital zircon U-Pb; Bull et al., 2013). The host-rock package protoliths are provisionally interpreted by Bull et al. (2015) as consistent with deposition as poorly sorted, subwave base, clastic turbiditic, debris flow and hemipelagic sediments, with intercalated carbonate reefs, deposited in a shallow marginal marine grading to continental and perhaps mildly evaporitic environment in a magmatically and tectonically active basin (Schlegel and Heinrich, 2015). The succession comprises:
• Shallow marine sequence of interbedded and brecciated, argillaceous dolostone, argillite (breccias), calcareous matrix-bearing and coarse-grained siliciclastic, clast-supported breccias, medium- to fine-grained greywacke, chlorite- and muscovite-cemented subarkose, and laminated and massive limestone and dolostone, as well as quartz-feldspar conglomerate and volcanic-clast conglomerate south of the deposit (Belperio and Freeman, 2004; Belperio et al., 2007; Freeman and Tomkinson, 2010). This suite incorporates the following three units which are locally interbedded within it:
• Algal and micritic laminated and massive dolostone with stylolite-like veins filled with chlorite and hematite. It contains brecciated zones rich in hematite and Cu sulphides, and has carbonaceous dolomite and shale near the base of the unit;
• Poly- and mono-mictic pebble to cobble breccias and coarse-grained clastic to finely laminated argillaceous rock types, dominantly of sedimentary origin, with lesser lithic sandstone beds. The breccias and coarse clastics comprise hematite-rich greywacke-shale breccia with clasts of sandstone, greywacke, siltstone, chert, hematite and basalt in a matrix of crystalline earthy hematite. Rare clasts of retrogressed gneiss are also encountered. Brecciation is strata bound and mainly single stage (Freeman and Tomkinson, 2010), with little evidence of refracturing of previously consolidated breccia. Variable brecciation intensity formed clast- or matrix-supported breccia sheets. Sharp breccia contacts are evidenced by a sudden change of clast size or type. Brecciation predates the hematite-dominant replacement (Schlegel and Heinrich, 2015). This unit is interpreted to represent subaerial, high-energy, clastic sedimentary deposition on an alluvial to fluvial plain immediately below the unconformity separating the Gawler Range Volcanic equivalents and red beds from the stratigraphically underlying shallow marine sedimentary rock package (Bull et al., 2015).
• Andesite with minor dacite, which were probably precursors to the succeeding volcanic sequence;
• Fine grained redbed sandstone with minor red mudstone which occurs as an infaulted block, the stratigraphic position of which is unclear;
• Strongly metasomatised basalt, again occurring as a fault isolated block whose affinity has not been determined;
- Disconformity or unconformity
Footwall volcanic sequence of the 'Neptune Volcanics' (Freeman and Tomkinson, 2010), interpreted to be equivalent to the Gawler Range Volcanic sequence (Belperio et al., 2007; Freeman and Tomkinson, 2010; Bull et al., 2015; Williams et al., 2017). Reflection seismic data reveal that the volcano-sedimentary sequence has a maximum thickness of around 4.5 km and overlies Archaean rocks of the Mulgathing Complex (Harris et al., 2013) ~7 km south of Prominent Hill in a south-verging fold and thrust duplex system. Whilst at Prominent Hill this sequence is overturned, dipping steeply north, it overlies Archaean basement to the south above a contact with a much shallower northerly dip (Harris et al., 2013), inferring that, apart from it being overturned immediately to the south of the Southern Overthrust, the sequence is otherwise relatively shallow dipping. The volcanic rocks of this sequence have geochemical characteristics similar to the Gawler Range Volcanics from elsewhere in the Gawler Craton, including marked enrichment of the most incompatible elements, along with negative Nb-Ta, Zr-Hf and Ti anomalies. Prominent Hill basalts also show strong similarities with a marginal gabbroic facies of the large 1562±14 Ma White Hill Igneous Complex ~5 km to the NW (Williams et al., 2017). The sequence comprises:
• Basalt and basaltic andesite - massive plagioclase porphyritic, amygdaloidal and fragmental facies basalt, basaltic andesite and minor dacite. These coherent footwall volcanic rocks include intercalated fragmental lithologies such as agglomerate, felsic tuff and ignimbrite, and volcanic clast conglomerate (Belperio et al., 2006) that had variably brecciated textures prior to the pervasive hematite replacement and subsequent Cu-(Fe) sulphide mineralisation. The hematite replacement involved extensive alteration to form an assemblage that includes sericite, hematite, chlorite, carbonate and leucoxene altered titanomagnetite.
• Felsic volcanic rocks become much more common several kilometres west of Prominent Hill, with dacite to rhyolite compositions and a distinctive reddish colouration due to hematite dusting of alkali feldspar. This contrasts with the predominantly sericite-chlorite-earthy hematite-leucoxene-carbonate alteration of the basalts and andesites in the deposit area.
- Unconformity or fault
Red-bed sequence with interlayered volcanic rocks - coarse to very coarse grained quartz-rich sandstone and conglomerate with graded and cross-bedding and scours, representing high-energy, oxidized clastic sediments deposited in a subaerial setting, probably an alluvial and/or fluvial plain (Bull et al., 2015). The sedimentary sequence is interrupted by interbedded sericite-chlorite altered amygdaloidal basalt, poorly sorted hematite-chlorite-sericite altered volcanic clast conglomerate and hematitic/sericitic volcanic siltstones. Conglomerates and K feldspar sandstones in the upper section of the red beds are hematite cemented with clasts of vein quartz, ironstone, rhyolite and altered basalt (Freeman and Tomkinson, 2010).
Mafic sills and dykes. The hematite-altered sedimentary host rocks are cut by:
• mostly unbrecciated sericite-chlorite-hematite-silica altered basaltic-andesitic, andesitic and dioritic sills and dykes, some of which have chalcopyrite in the sericite-rich matrix and contain sparse amygdules filled with fluorite, barite and siderite. Chilled margins are not abundant;
• dolerite dykes that post date mineralisation, cut all hanging wall rocks and have been tentatively equated with the ~820 Ma Gairdner Dolerite dyke swarm (Freeman and Tomkinson, 2010).
- Permian unconformity
Phanerozoic cover sequence (after Freeman and Tomkinson, 2010)
• Early Permian Boorthanna Formation - diamictite overlain by rhythmically bedded coarse and fine-grained clastic rocks;
• Lower Cretaceous Cadna-owie Formation - sandstone, siltstone, calcareous sandstone, pebbly sandstone and some feldspathic intraformational conglomerate;
• Late-Early Cretaceous Bulldog Shale - predominantly grey mudstone.
Alteration and Mineralisation
The formation of both hematite-dominated alteration and economic Cu-(Fe) sulphide mineralisation (or at least some) took place during and after rotation of the brecciated host package. This interpretation is based on geopetal structures in the high-grade mineralised breccia, and further supported by the presence of pre-mineral dykes intruding the overturned sedimentary hosts (Schlegel and Heinrich, 2015; Williams et al., 2017). The following alteration, particularly that related to iron oxide, is observed:
• Magnetite skarn developed in the Palaeoproterozoic, probable Wallaroo Group equivalent, metasedimentary rocks of the hanging wall metamorphic sequence. These rocks are altered to a 'skarn' assemblage of massive magnetite with phlogopite-chlorite-pyrite ±chalcopyrite ±actinolite-tremolite ±serpentine ±talc ±scapolite (Freeman and Tomkinson, 2010). Pyrite is abundant, filling fractures and veins within massive magnetite and locally comprising up to 20 vol.% of the 'skarn' assemblage (Belperio and Freeman, 2004), although the Cu grade is typically <0.2%. This assemblage may represent either a single event or be the result of episodic Fe ±K ±Mg alteration, as observed elsewhere in the Mount Woods Domain (e.g., Joe's Dam South East prospect; Schlegel and Heinrich, 2015). In drill core martite (hematite pseudomorphs after magnetite skarn) occurs as zones separated by metre-scale layers of chlorite breccia, granitoid and local fault zones. Magnetite-quartz-calcite-fluorite-barite ±pyrite ±chalcopyrite veins cut all lithologies of the hanging wall metamorphic sequence. Rare calcite-quartz ±hematite ±fluorite ±chalcopyrite veins that have been correlated to the Cu mineralisation in the Main Host Sequence (Belperio et al., 2007), indicating the magnetite skarn predated the hematite-associated economic Cu mineralisation. Rocks composed of the skarn alteration assemblage have been intruded by a dacite porphyry with a crystallisation age of 1586 ±12 Ma (LA-ICP-MS U-Pb dating of zircon; Bowden et al., 2017).
• Hematite breccias in the Main Host Sequence are the result of pervasive hematite replacement of host carbonate and pre-existing siliciclastic breccias. This interpretation is supported by the comparison of textures seen in regional, hematite-barren, calcareous sedimentary rocks and those of hematite breccias from Prominent Hill (Schlegel and Heinrich, 2015). Pervasive hematite can be observed to have variably replaced calcareous and dolomitic lithologies. Although in some cases this replacement has progressed to almost complete obliteration of previous textures, in many cases pre-alteration and pre-mineralistion sedimentary textures and structures are partially retained, e.g., layering, brittle fracturing, angular and rounded clast outlines, boudinage and aligned clast fabrics (Schlegel and Heinrich, 2015). Replacement appears to have commonly increased porosity and probably enhanced permeability of the hematite breccias, compared to their protoliths. There is no hydrothermal magnetite associated with the hematite alteration south of the Hanging-wall Fault, with the only magnetic features reflecting post-mineral dykes (Williams et al., 2017).
The breccias contain a range of clast types, including both fragments of the host rock, as well as red-dusted exotic granitoid clasts. These breccia clasts have a frayed appearance, and in addition to the matrix, may also be partially to totally replaced by hematite. As well as hematite, hydrothermal alteration has produced assemblages including carbonates (siderite, ankerite and dolomite), sericite (phengite), chlorite, fluorite, barite, quartz, fluorapatite, REE minerals (including monazite), uraninite, coffinite and Cu-(Fe) sulphides. Some of these minerals may not be entirely of hydrothermal origin, e.g., chlorite, muscovite and sericite, which are seen to be original constituents of the matrix of unaltered protoliths. Never the less, much of these minerals are formed by hydrothermal alteration and can be seen to be the products of the breakdown of feldspars seen in the unaltered host rocks (Schlegel and Heinrich, 2015). Two end member hematite breccias have been recognised, with transitional varieties developed in brecciated and unbrecciated host rocks. The two hematite breccia types together constitute the Prominent Hill hematite breccia body:
i). hematite-quartz alteration, which is mostly in brecciated rocks, and is characterised by an assemblage of hematite-quartz ±fluorapatite ±barite ±fluorite ±REE minerals ±gold. This alteration style is referred to as 'steely hematite' by Belperio et al. (2007) and Freeman and Tomkinson (2010) and coincides with the gravity anomaly initially drilled that led to the discovery of the deposit. It occurs as a dense, hard rock that overprints earlier mineralised breccia, barren breccia and volcanic rocks. It comprises extremely fine (<10 µm) hematite intricately mixed with cryptocrystalline silica in altered volcanic rocks, whilst in calcareous siliciclastic protoliths it produced cryptocrystalline silica intergrown with hematite. In zones of more intense pervasive alteration, hematitic jasperoid resulted. It contains pervasively hematised and/or silicified angular cherty clasts. Late-stage veins containing barite and specular hematite cut the breccia. Where hematite-quartz is pervasive, both brecciated and unbrecciated host rocks were variably altered, first causing depletion in elements such as Na, K, Mn, Mg and Al and replacement of chlorite and sericite by kaolinite. During this process and subsequently, feldspar, carbonate, amphibole, muscovite and kaolinite were removed and substituted by hematite and/or quartz. Pre-existing breccia textures were progressively destroyed, as indicated by the 'fraying' and irregularly hematite-quartz embayments eroding clast margins. Other characteristics include: a). euhedral to subhedral quartz and hematite crystals projecting into small breccia cavities; b). dull colour changes in semi-altered clasts, and c). a reduction of porosity (Schlegel and Heinrich, 2015). This reduction in porosity, in contrast to the hematite-quartz alteration in the breccia, is interpreted to be due to the more intense hematite alteration and the major addition of silica which progressively occluded permeability and porosity (Schlegel and Heinrich, 2015).
These breccias and the transition to the adjacent hematite-chlorite-sericite-altered rocks host the bulk of the Au (+barite) mineralisation, but are generally poor in Cu-(Fe) sulphides. These enhanced gold concentrations are generally found in a zone some 10 to 20 m wide, typically containing 4 to 6 g/t Au, commonly at the outer edge of the hematite-quartz alteration zones, accompanied by abundant hematite and silica in the breccia matrix. Gold grains, vary in size from <5 to >500 µm, and have rims of copper sulphide and complex symplectic textures with both copper and iron oxides.
ii). hematite-aluminosilicate breccia, containing hematite-chlorite-sericite ±siderite ±ankerite ±kaolinite ±barite ±fluorite ±fluorapatite ±REE minerals [e.g., monazite], uraninite, coffinite ±Cu-sulphide alteration. On the basis of the K/Al ratio in bulk assay data, Schlegel and Heinrich (2015) infer the 'sericite' that is closely associated with the Cu(-Fe) mineralisation is a phengitic muscovite. This 'sericite' has a probable 1585 and 1570 Ma age range (49Ar/39Ar; Bowden, 2017). These breccias are generally porous and softer than the hematite-quartz breccia and have textures that vary from being finely layered, to massive, with fine-grained to mm- scale crystalline hematite aggregates, meshes or disk-shaped networks. They are typically dark grey to black, although in contrast, the earthy hematite matrix (see below) on the margin of this breccia is generally fine grained, granular and red to brown (Schlegel and Heinrich, 2015). The porosity and permeability of these breccias which may have contributed to their susceptible
to increased fluid penetration is interpreted to be the product of inferred carbonate dissolution and widespread hematite replacement which is likely associated with net mass loss of CaO, Na2O, and variable CO2 and Al2O3
to increased fluid penetration over time (Schlegel and Heinrich, 2015).
Hematite-aluminosilicate breccias are the main host to Cu mineralisation which predominantly occurs within the breccia matrix.
Cu-(Fe) sulphide minerals that are abundant in the breccia matrix include chalcocite, digenite, bornite, idaite and chalcopyrite. These sulphides are variously intergrown with fine- to coarse-grained hematite, sericite, barite, fluorite, carbonate and locally quartz, or replace euhedral to anhedral pyrite (Belperio et al., 2007; Schlegel, 2010). Cu-(Fe) sulphides also infill voids in earlier hematite-quartz altered breccia where the two styles overlap. High Cu grades associated with chalcocite are typically confined to sections of the breccia matrix containing fine- to coarse-grained crystalline hematite and visible sericite (hematite-sericite matrix breccia; see below). Intense hematite-fluorite-barite alteration (hematite-fluorite matrix breccia; see below) is also locally developed in spatial association with chalcopyrite-pyrite mineralisation, occurring in patches adjacent to hematite-only breccia matrix. Discrete accumulations of fluorite and/or barite are intergrown with hematite and Cu-(Fe) sulphides in these patches. Calcite and siderite accompany the hematite-fluorite-barite assemblage and are generally fine grained, filling matrix interstices or are major constituents of veins cutting the hematite breccia (Schlegel and Heinrich, 2015).
Some hematite can be seen to occur as pseudomorphs after feldspars in volcanic clasts, whilst Cu-(Fe) sulphide minerals also replace breccia clasts and unbrecciated host rock, although not homogeneously across the entire breccia or host rock unit. For example, small sub-cm sized clasts are commonly completely replaced by chalcocite or chalcopyrite and fluorite, whereas larger clasts are partially corroded, and fracture controlled alteration of unbrecciated hosts produces pseudobreccias. High grade Cu can occur over short intervals as chalcocite- and bornite-rich replacements of unbrecciated host rock. Where replacement mineralisation impinges on hematite-quartz altered breccia, Cu-(Fe) sulphides are intergrown with barite and together replace breccia clasts, whilst the matrix remains poor in Cu sulphides and barite (Schlegel and Heinrich, 2015).
Variably developed Cu-(Fe) sulphide assemblages, with or without pyrite, are found throughout the Main Host Sequence and upper sections of the footwall volcanic sequence and display complex textures. Those developed in previously hematite-quartz altered breccia usually comprise chalcocite replacing digenite-coated bornite. Rims of Fe-depleted chalcocite and digenite surround bornite relicts in weakly mineralised hematite-quartz altered rocks and in transition zones to hematite-chlorite-sericite altered breccia. However, no other obvious deposit-scale zonation of Cu-(Fe) sulphides or dependence of mineralogy or depth below the palaeoerosion surface has been observed. Paragenetic relationships of mineral precipitation is therefore derived mainly from smaller scale petrographic relationships (Schlegel and Heinrich, 2015). Petrographic observations of the relationships between chalcocite, bornite, chalcopyrite, pyrite and hematite indicate copper, gold, uranium and rare earth elements were introduced contemporaneously with iron oxides. While hematite was introduced in all stages of the system, the relatively rare sulphides are inferred to have developed in the sequence pyrite → chalcopyrite → bornite → idaite → chalcocite (Schlegel and Heinrich, 2015). This progression is consistent with the continued introduction of a Cu bearing oxidised solution to replace Fe in existing sulphides with Cu to advance from pyrite to chalcocite and in doing so, liberate Fe to combine with the excess O to form hematite.
Hematite-aluminosilicate breccias were divided into two sub-grouping by Belperio et al. (2007) and repeated by subsequent authors, reflecting some of the sulphide-gangue associations detailed above. These have been described as follows by Belperio et al. (2007):
- Chalcopyrite-uranium or hematite-fluorite matrix breccia, which are characterised by an assemblage of chalcopyrite-gold ±bornite ±uraninite ±fluorite ±pyrite in a strongly developed breccia that has a purple, dark grey to jet black, porous matrix, with rounded clasts of intrusive, volcanic and sedimentary rocks. This variety commonly displays well-preserved compositional layering and contains late barite-quartz-fluorite veining. Grades typically average ~1.4 wt.% Cu and 0.6 g/t Au, whilst the uranium content locally exceeds 0.5%, commonly associated with high concentrations of fluorite in the matrix and late barite veining. Uranium occurs as sporadic, fine, discrete grains of coffinite and uraninite as inclusion within hematite or chalcopyrite. Rare earth elements cerium and lanthanum are more widespread than uranium, occurring as cerium-bearing apatite, cerium- and lanthanum-bearing monazite, bastnaesite, and a variety of rare earth element oxides throughout the broad iron oxide alteration halo. However, local grains of coffinite also occur in distal crackle-veined earthy breccias. Rare earth element concentrations average about 3000 ppm cerium + lanthanum, whilst silver grades average about 3 g/t, but are generally lower than in chalcocite-bornite zones.
- Chalcocite-bornite or hematite-sericite matrix breccia, which are characterised by an assemblage of chalcocite-gold ±bornite ±covellite ±diginite in a strongly developed breccia that has a bluish-grey, moderately well layered matrix, with sedimentary and/or volcanic clasts that are wispy shaped and sericitised, or rounded and 'rubbly'. These shapes reflect progressive pervasive hematite and/or sericite alteration of coarse clasts of claystone, chert, volcanic rock and sandstone. The matrix comprises platy hematite crystals, granular hematite aggregates, interstitial earthy hematite, and variable quantities of disseminated copper sulphides. Chalcocite and bornite are abundant and relatively fine grained. Grades typically averaging ~2.5 wt.% Cu and 0.6 g/t Au with low to moderate <0.01% U and <400 ppm F compared to the chalcopyrite breccias. Chalcocite is found as both simple grains within the matrix and as complex intergrowths with hematite, whilst bornite occurs as symplectic intergrowths with chalcocite, inferred to suggest coprecipitation. Bornite and chalcocite are also commonly seen to replace earlier formed euhedral pyrite.
Prominent Hill drill hole DDHURAN, the 'discovery hole'
a - hematite altered brecciated sandstone, at a depth of 242 m in a 44 m interval that averaged 1.52% Cu, 0.63 g/t Au;
b - hematite altered heterolithic breccia, with sandstone, mafic volcanic and hematite clasts, at a depth of 293 m, in a 35 m interval averaging 4.3% Cu, 0.67 g/t Au;
c - fined grained protolithic sedimentary sandstone breccia with minor hematite in matrix and overprinting hematite bands, at a depth of 310 m, in a largely un-mineralised interval between 307 and 430 m;
d - heterolithic sedimentary breccia with mafic volcanic and siliceous sedimentary clasts in a hematitic sandy matrix, at a depth of 332 m, in the same largely un-mineralised interval between 307 and 430 m;
e - heterolithic breccia with sandstone, mafic volcanic and hematitic clasts in a strongly altered to steely hematite matrix, at a depth of 440 m, within an interval between 430 and 466 m that averaged 1.6% Cu, 0.75 g/t Au;
Core from SA Geological Survey Core Library; Images by Mike Porter 2022.
• Earthy hematite alteration and veining - pervasive earthy and granular hematite alteration/flooding and weak brecciation, together with crackle-veined stockwork veining that is predominantly composed of hematite with lesser carbonates, are developed within argillaceous and dolomitic sedimentary rocks peripheral to copper mineralised breccias. The veins are irregular and up to 10 cm thick and occur as crackle and stockwork arrays with a dominant mineralogy of hematite + carbonate + gold. However, in addition to this base assemblage, other mineral packages define four distinct vein types, which in addition to gold, comprise (Schlegel and Heinrich, 2015):
i). Specular hematite-Cu-(Fe) sulphides ±quartz;
ii). hematite-calcite-barite-pyrite ±fluorite ±chalcopyrite ±bornite ±chalcocite ±magnetite;
iii). hematite-siderite-quartz-fluorite-barite chalcopyrite ±calcite, although a variety without barite and calcite, is also locally abundant; and
iv). hematite-barite-bornite-chalcocite.
The same mineral assemblages also fill porous breccia and centimeter-sized cavities in the breccias. In addition, rare paragenetically late fluorite-pyrite-pyrrhotite-magnetite-bearing veins occur locally in the footwall of the Hanging-wall Fault zone. Gold grains within these veins range in size from 5 to 800 µm. Petrological and SEM observations of these gold grains show a persistent association of gold, copper (bornite, chalcocite or chalcopyrite) and hematite, implying a genetic relationship. In particular, gold grains are both rimmed with a copper sulphide, and contain minute copper sulphide inclusions. The rims and inclusions may be either chalcocite or chalcopyrite, and are ubiquitous whether proximal or distal to the high grade copper zones (Schlegel and Heinrich, 2015; Belperio et al., 2007).
A well developed alteration succession can be seen in the volcanic rocks of the Footwall Volcanic Sequence in the structural footwall of the deposit. These rocks were albitised before ore formation. Albite was sequentially overprinted by chlorite, Fe-Mg bearing illite, hematite and sulphides+carbonates. The white mica-hematite alteration intensity increases unevenly towards the orebody, controlled by variations such as the primary porosity, stratigraphic contacts and fracturing. Much of the hematite formed directly as microspecularite with no precursor magnetite. Hematite is commonly associated with minor minerals such as apatite, barite and REE minerals (mainly Ca-REE fluorocarbonates), florencite and REE-only fluorocarbonates that are found in the orebody (Williams et al., 2017). The same authors conclude that the distribution of ore and ore-related alteration at Prominent Hill suggests that fluid flow during mineralisation was strongly influenced by the major discontinuity between the stratigraphic base of the volcanic sequence and the Main Host Sequence.
Simplified geological map of the Prominent Hill deposit area below the 90 to 150 m thick Phanerozoic cover overlying a Permian unconformity, and is based on drilling. The main copper-gold bearing clastic and shelf carbonate sequence is located between metamorphic calcsilicate and metasomatic skarn altered rocks to the north, and unmetamorphosed but strongly altered, volcanic rocks to the south. The main copper-gold ore is hosted by the hematite altered polymictic breccias, while the hematite quartz breccias contain variable gold. The location of the principal resource blocks (Main Prominent Hill Cu-Au, Eastern Cu-Au, Western Au and Western Cu) which lie within these breccias are indicated. Modified after: Williams et al. (2017) and Freeman and Tomkinson (2010).
Economic Cu mineralisation at Prominent Hill was introduced comparatively late in the mineral paragenesis (Freeman and Tomkinson, 2010), and comprises relatively abundant Cu sulphides in an oxidised, otherwise low sulphur system. Hematite-dominant alteration continued throughout all of the stages of mineralisation, as evidenced by multiple hematite overgrowths and encrustations. Within the matrix of hematite breccias, early pyrite occurs as euhedral to subhedral crystals, or is intergrown with hematite to form anhedral aggregates (Schlegel et al., 2017). This implies at least two generations of pyrite were deposited, ± weak chalcopyrite, apparently predating much of the Au mineralisation, which in turn, was temporally associated with hematite-quartz alteration and late barite-bearing veins. The Au and barite were succeeded by late hematite-aluminosilicate alteration of brecciated/permeable rocks accompanied by Cu mineralisation that included chalcopyrite [CuFeS2], bornite [Cu5FeS4], idaite [Cu5FeS6], digenite [Cu9S5] and chalcocite [Cu2S] accompanied by barite, with chalcopyrite seen to replace the early pyrite (Belperio et al., 2007; Schlegel, 2010). Bornite coexists with chalcocite and chalcopyrite, but chalcocite and chalcopyrite are not observed together (Belperio et al., 2007). Mineralisation appears to have evolved with the progressive addition of Cu from an early low Cu:S ratio pyrite-chalcopyrite with accompanying hematite to a high Cu:S suite including chalcocite, also with hematite, as recorded by mutually overprinting generations of Cu-(Fe) sulphide assemblages (Schlegel and Heinrich, 2015). This progression is observed in particular in hypogene sediment hosted copper deposits (e.g., the Kupferschiefer ores in Poland; Oszczepalski, 1999). The reaction is chalcopyrite → bornite: 2 CuFeS2 + 3 Cu+ + e ↔ Cu5FeS4 + Fe2+; and bornite → chalcocite: Cu5FeS4 + 3 Cu+ + e ↔ 4 Cu2S + 4 Fe2+ (e.g., Macleod and Muir, 1983). Zhao et al. (2014) showed the chalcopyrite to bornite reaction increases in efficiency over 200°C, and in detail, can be achieved by reactions such as 2 CuFeS2 + 3 Cu(HS)2- + 3 OH- ↔ Cu5FeS4
+ Fe(OH)2 + 6 HS- + 0.5 H2O. At lower temperatures (e.g., in porphyry Cu supergene blankets, with continued addition of Cu, coatings and direct replacement of pyrite and chalcopyrite by chalcocite is common, with other secondary Cu-(Fe) sulphide by-products, without increasing S content.
Petrographic observations of the relationships between these sulphides, other minerals and hematite indicate Cu, Au, U and REE were emplaced contemporaneously with iron oxides (Belperio et al., 2007). Oriented drill core containing geopetal markers show economic Cu sulphide mineralisation was deposited during and after the brecciated host strata had been rotated to a steeply dipping attitude, close to the current geometry. This and probable 1585 and 1570 Ma age range (49Ar/39Ar; Bowden, 2017) of phengitic muscovite that accompanies economic Cu mineralisation, suggest that the latter occurred after, or late in, the extrusion of the inferred ~1590 Ma lower Gawler Range Volcanic equivalents of the Footwall volcanic sequence (Belperio et al., 2007). Also, the absence of significant Cu mineralisation in the 'magnetite skarn' in the Hanging wall metamorphic sequence, other than regional subeconomic pyrite-(chalcopyrite), suggest it was tectonically juxtaposed after the main stage economic Cu mineralisation at Prominent Hill (Schlegel et al., 2018).
Introduction of Sulphides - On the basis of the observations outlined above, sulphides are interpreted to have been introduced in two stages (Schlegel et al., 2017):
• Stage I, which is characterised by pyrite and minor chalcopyrite in magnetite-altered metasedimentary rocks, part of a pre-ore magmatic hydrothermal sulphide phase responsible for regional magnetite-sulphide alteration and barren to low grade iron oxide accumulations. Deposition of these sulphides was in response to the host rocks interacting with multiple magmatic-hydrothermal fluid pulses that had evolved along a moderately reduced reaction path (Schlegel et al., 2017). Such mineralisation is found throughout the Olympic IOCG Province and Mount Woods Domain, and includes the 'magnetite skarn' above the Hanging-wall Fault at Prominent Hill which has a mineralogy that includes phlogopite + chlorite + pyrite + chalcopyrite + actinolite-tremolite + serpentine + talc + scapolite. At least two early pyrite generations within the matrix of hematite (rather than magnetite) breccias at Prominent Hill, as described above, can be shown to texturally predate high grade Cu mineralisation, and are interpreted to represent this stage I (Schlegel et al., 2017).
• Stage II - petrographic evidence supports Stage I being followed by a late, magmatic derived, oxidised sulphide phase involving multiple pulses of fluid that had first interacted with the oxidised surface environment. Examples of stage II sulphides are observed to have formed by the reaction of stage I pyrite with Cu-bearing fluids to produce Cu sulphides. Petrographic evidence from ore textures, distribution of sulphides and barite and sulphur isotope data are interpreted (Schlegel et al., 2017) to indicate sulphide stage II mineralisation was caused by a combination of two end-member processes, but also a third where sulphides form directly with hematite, namely:
i). magmatic sulphur disproportionation* followed by reduction of magmatic sulphate and precipitation of Cu-(Fe) sulphides. Disproportionation of magmatic sulphur resulted from the mixing of oxygen-rich meteoric waters and acid magmatic hydrothermal fluid at or near the surface:
4 SO2 + 4 H2O + 2 O2 ↔ 4 HSO4- + 4 H+
This reaction produced sulphuric acid (accompanied by hydrofluoric acid generated from the abundant F within the same fluids) which leached Cu from the enclosing volcanic rocks. The Cu and acid magmatic sulphate were subsequently co-transported to the deposit site where the latter was neutralised by reaction with a reductant containing Fe2+ present within, or advected into, the host rock package, as follows:
HSO4- + 8 Fe2+ + 8 H2O ↔ 4 Fe2O3 + H2S + 15 H+. This neutralisation of acidity led to the release of disolved Cu that combined with available reduced S in H2S to precipitate Cu-(Fe) sulphides;
ii). isovolumetric replacement of stage I precursor pyrite by Cu-rich sulphides during sulphide stage II. This involves the replacement of previously precipitated magmatic-hydrothermal pyrite by Cu-rich sulphides, with incomplete isotopic equilibration between pre-existing and newly formed sulphides during reaction with an oxidised fluid containing abundant Cu. In the process, Fe2+ is expelled as a by-product, as follows:
14 Cu2+ + 5 FeS2 + 12 H2O ↔ 7 Cu2S + 5 Fe2+ + 3 HSO4- + 21 H+.
Note that this simplified equation is shown to generate chalcocite direct, although observation indicates a progression through chalcopyrite and bornite as detailed previously may have occurred also.
iii). direct formation of hematite and sulphides from a fluid with Cu, limited sulphur as reduced aqueous sulphide, in the presence of high total Fe, in the same fluid, a second fluid or host rock, to form hematite and chalcopyrite:
Cu2+ + 2 HSO4- + 9 Fe2+ + 4 H2O ↔ 4 Fe2O3 + CuFeS2 + 8 H+. This process is similar to that in Stage I where the fluid has limited S and Cu to produce weak pyrite-chalcopyrite with hematite/magnetite mineralisation. The balance between hematite and magnetite is governed by the equilibrium between the following two reactions
3 H2O + 2 Fe2+ ↔ Fe2O3 + H2 + 4 H+ and 3 Fe2O3 + H2 ↔ 2 Fe3O4 + H2O.
This is influenced by the amount of H2 in the fluids and sinks for H+ in acid consuming alteration (Schlegel et al., 2018).
Stage II Cu sulphides, the deposition of which postdated, or overlapped, but outlasted the stage I magnetite- (or hematite)-associated sulphide, are responsible for the bulk of economic mineralisation at Prominent Hill (Schlegel et al., 2017). Stage II mineralisation also postdates the onset of the extrusion of the mafic to intermediate lower Gawler Range Volcanics (Carter et al., 2003; Belperio et al., 2007; Harris et al., 2013) as these rocks are mineralised in the Prominent Hill area.
* NOTE: Disproportionation is a reaction in which a substance is simultaneously oxidised and reduced, giving two different products.
Fluid Inclusions - Applying petrography, microthermometry, and LA-ICPMS microanalysis, Schlegel et al. (2018) were able to characterise pre-, syn- and post-mineral fluid inclusion assemblages and discriminate four fluid end members, as follows:
• Fluid A, interpreted to comprise oxidised surface water containing magmatic solutes. It is the main Stage II ore fluid, and occurs as inclusions in fluorite and barite that are intergrown with Cu sulphides in hematite breccia matrix. It is weakly saline (≤10 wt.% NaCl equiv.) and contains low concentrations of K, Pb, Cs and Fe (600 ppm), but is rich in Cu (~1000 ppm) and U (0.5 to 40 ppm). Its low molar Br/Cl ratio of 0.003 is typical of magmatic fluids, acidic volcanic lake water and evaporated bittern brines, whilst its low Ca and comparatively high K contents, suggests a magmatic-derived origin of the salinity in the inclusion. The Cu and U content are consistent with a fluid that is highly acidic and highly oxidised (e.g., Bastrakov et al., 2010). Its high Al content is also consistent with a highly acidic fluid and the large-scale Al leaching in the core of the Prominent Hill alteration system (Schlegel and Heinrich, 2015). Schlegel et al. (2018) argue the source of this fluid could not have been a playa lake, because such a surface brine would not have been acidic enough to produce the intense hydrothermal alteration, Al leaching and mineral zonation it is interpreted to have produced at Prominent Hill. Schlegel et al. (2018) therefore suggest the solute inventory of the fluid was of magmatic origin, derived through shallow fluid exsolution and degassing of late Gawler Range Volcanics, which were disolved in meteoric water that became enriched in magmatic components. This was followed by complete oxidation of magmatic sulphur via atmospheric oxygen in a volcanic lake environment. The cooling and oxidation of magmatic SO2 in water produced sulphuric acid which aggressively leached Cu and U from eruption rubble in the vent, subaerial lavas and wall rocks of lakes and rivers (Christenson and Wood, 1993). The fluorine-rich nature of the Gawler Range Volcanics magmas (Agangi et al., 2012), suggest the effusive volcanism probably also released HF in gaseous or readily leachable form which would have further contributed to the fluids ability to leach metals. The low Ca concentration of fluid A allowed efficient fluorine transport that eventually became enriched in the deposit by interaction with more Ca-rich brines or host rocks. This fluid is interpreted to have then migrated through oxidised aquifers to the site of the Prominent Hill deposit, where it became the main driver of Stage II copper mineralisation (Schlegel et al., 2018).
An alternate explanation is that the magmatic component of Fluid A was derived from a large, lower crustal mafic to ultramafic magma chamber (as implied by magnetotelluric and deep seismic data; Reid, 2019) rather than having degassed from late Gawler Range Volcanics. As such it may have been derived from fluid collected in the apex of that chamber having segregated from the large volume of magma below, and already be enriched in Cu as well as the other elements detailed above. It rose to shallower depths and mingled with highly oxidised basinal brines in a fault controlled basin filled with red-beds, tuffs and volcano-sedimentary rocks to form the hybrid fluid A, and been modified as described above. If this basin was hydraulically connected to the underlying host sequence, the fluid could have migrated down through oxidised aquifers to the site of the Prominent Hill deposit while scavenging additional Cu and U en route.
• Fluid B, which is a modified magmatic-hydrothermal brine, is found in inclusions hosted by siderite-quartz-fluorite-chalcopyrite and barite-chalcopyrite bearing veins that crosscut the Prominent Hill hematite breccia. Similar fluid inclusion assemblages occur in barren magnetite-quartz veins cutting the weakly mineralised 'magnetite skarn' above the Hanging-wall Fault. It is dominated by NaCl and is hypersaline, the most saline of the four fluids, with a total NaCl+CaCl2 concentration of 36 to 45 wt.% and a low Ca/Na mass ratio of 0.3. It also has the highest concentrations of K, Fe, Mn, Pb and Cs and contains ~70 ppm Cu with a composition that is typical of a moderately reduced magmatic-hydrothermal brine modified by fluid-rock interaction (Audétat et al., 1998, 2008; Ulrich et al., 2001; Samson et al., 2008; Kouzmanov and Pokrovski, 2012). The fluid-rock interaction is interpreted to have involved quartz-feldspar±carbonate bearing rocks, which increased the Ca/Na ratios in the fluid to a moderate degree (Dolejs and Wagner, 2008; Hennings et al., 2017) compared to pristine magmatic fluids. The high temperature of origin of the fluid inclusion assemblage imply that magmatism was active during the formation of the deposit. Schlegel et al. (2018) conclude that the solute components in fluids A and B were both ultimately sourced from magmas with similar composition, but the two are distinct fluids that have evolved along different fluid-flow pathways in the system.
Very similar fluid inclusion assemblages are found in barren magnetite-quartz veins cutting the weakly mineralised magnetite-'skarn' like alteration assemblage in the Hanging wall metamorphic sequence and in the Joe's Dam sub-domain 10 to 12 km to the NW (see images above for location). Although these fluids cannot be temporally linked across the Hanging-wall Fault, neither has produced economic mineralisation and both are tentatively linked as a regional Fluid B. Albite, magnetite and hematite replacement of feldspar and carbonate-rich rocks at Joe's Dam and Joe's Dam South East (Freeman and Tomkinson, 2010) in the vicinity of the fluid-saturating 1562 ±14 Ma (U-Pb zircon; Allen et al., 2016) White Hill Igneous Complex supports a magmatic source of the high temperature Fluid B, modified by exchange of Na for Ca. This interpretation is further supported by the very similar sulphur isotope signature of pyrrhotite and chalcopyrite from magnetite-bearing miarolitic cavities in the marginal gabbro of the intrusion to those of Fluid B, and the presence of magnetite bands in the same gabbro (Schlegel et al., 2017). This implies that late Hiltaba age mafic to ultramafic intrusives may be the source of Fluid B, possibly exsolved and expelled due to mingling with Balta age granites or felsic gneiss country rocks as described above in the Regional Alteration and Mineralisation section.
• Fluid C, a non-magmatic crustal rock-buffered brine that occurs as inclusions in fluorite and barite within bornite-chalcocite bearing hematite-aluminosilicate breccia matrix ores. It is a CaCl2-bearing and NaCl dominated calcic-sodic brine with an intermediate salinity of 16 to 28 wt.% NaCl+CaCl2, an elevated Ca/Na ratio of ~0.6 and high Br/Cl ratios, characteristic of basin brines of residual bittern origin. It carries less Fe than fluid B, but contains ~200 ppm Cu, and likely contributed metals to economic mineralisation. Schlegel et al. (2018) note that the Pb/Cl ratios are similar to those in metal rich basinal brines (Stoffell et al., 2008; Fusswinkel et al., 2013; Wilkinson, 2013; Wagner et al., 2016), supported by the ranges in Ca/K and K/Na mass ratios. Fluid inclusion assemblages similar to fluid C closely overlap data from red bed brines, interpreted to be modified sediment hosted Cu ore fluids (e.g., Kupferschiefer; Fusswinkel et al., 2014). In addition, fluid C data overlap with metalliferous oil field brines from the central Mississippi basin. A component of magmatic salinity is possible in addition to contributions from sedimentary rocks or bittern brines. Fluid inclusion assemblages that approximate end-member fluid C contain ~500 ppm S, which is typical of the sulphate content of basin brines with 30 wt.% total salinity (Hanor, 1994). However, sulphur isotope data provides no evidence of a major sulphate contributions from a sedimentary-evaporitic source, but are readily explained by partial inheritance of S from preexisting pyrite in the host rock sequence (Schlegel et al., 2017).
• Fluid D is a basement brine and is hosted by inclusions in fluorite of late calcite-barite-pyrite-fluorite veins of the ore stage crosscutting the hematite breccia. It is a Ca-dominated and Na-bearing brine with a total salinity range of between 19 and 30 wt.% NaCl+CaCl2, whilst the concentrations of K, Mn, Cs and Pb are similar to those of fluid C. Fluid inclusions that are predominantly composed of fluids C or D have a continuum of Ca/Na ratio, but fairly constant and distinct Cs/Cl and Pb/Cl ratios. The fluid D Ca/Na ratio of is much higher than in typical basin brines. It is interpreted to be a basement brine with characteristically high Ca/Na ratios of ~2, and averages ~100 ppm Cu. This fluid appears to play no significant role in economic Cu-Au mineralisation, as petrographic evidence show that it typically postdates sulphide precipitation in the breccia matrix, although it may have been significant during Cu remobilisation (Schlegel et al., 2018).
Sulphur Isotopes - Schlegel et al. (2017) showed that sulphides from the Prominent Hill deposit and nearby prospects in the surrounding Mount Woods Domain have a wide range in δ34SV-CDT values of between -33.5 and 29.9‰ for Cu-(Fe) sulphides, and a narrower range of 4.3 to 15.8‰ for barite. The Fe sulphides pyrite and pyrrhotite have a narrower range of sulphur isotope compositions, whereas Cu-bearing sulphides, bornite, chalcocite, digenite and idaite on average, have a much wider variation, and more negative δ34SV-CDT values of between -16.2 and -4.6‰.
This wide spread has been interpreted in terms of diverse sulphur sources that include contributions from magmatic, sedimentary, seawater or evaporitic sulphur. In order to test these alternatives, Schlegel et al. (2017) performed a detailed sulphur isotope study of Cu-(Fe) sulphides from Prominent Hill and IOCG prospects (e.g., Joe's Dam) within a 30 km radius which had been influenced by similar regional fluids, particularly Fluid B as described above. This study concluded that the diversity in sulphur isotope composition can be produced by different fluid evolution pathways along reducing or oxidising trajectories, but ultimately derived from a magmatic source that had a δ34SBulk composition of 4.4 ±2‰. This value approximates the magmatic sulphur isotope value determined from pyrrhotite of a Hiltaba Suite-age gabbro in the Mount Woods domain (Bastrakov et al., 2007) similar to that that appears to be the source fluid for mineralisation at Joe's Dam as detailed above (Schlegel et al., 2018). A reduced pathway is indicated for the Stage I sulphide mineralisation related to Fluid B, when intrusion-derived magmatic-hydrothermal fluids produced early pyrite and minor chalcopyrite at Prominent Hill, and iron ±copper sulphides in regional magnetite 'skarns' and in some pervasively altered volcanic rocks of the Gawler Range Volcanics. An oxidised regime was interpreted from related reduced magmatic-hydrothermal fluids vented to the surface, completely oxidised by reaction with atmospheric oxygen to produce sulphate and sulphuric acid with a sulphur isotope composition equal to their magmatic source. These were mixed with oxidised water from the hydrosphere and returned to depth, but contained magmatic solute components, notably sulphate to mix with reduced fluids at depth and react with Stage I sulphide to produce the Stage II mineralisation. Schlegel et al. (2017) argue that their modelling of the sulphur isotope fractionation processes in response to reducing and oxidising pathways demonstrates the entire spectrum of sulphur isotope data from Stage I and Stage II mineralisation can be explained with a single, ultimately magmatic sulphur source. However, some of the early Stage I (or pre-Stage I) pyrite in the host sequence is of sedimentary origin, diagenetic, or partly reworked by early sedimentary brecciation (Allen et al., 2016).
Fluid Mixing and Wallrock Reaction - Schlegel et al. (2018) conclude economic Cu-Au mineralisation at Prominent Hill is the consequence of concurrent fluid mixing and fluid-rock reaction.
Mixing of fluids A, B and C is interpreted to have dominated the deposition of hematite, low-sulphidation and low total sulphide Cu-(Fe) mineralisation in the Prominent Hill high-grade orebody, and the main gangue minerals, including fluorite and barite. The same authors interpret fluid A to be the main ore fluid contributing the bulk of the Cu, the relatively small amount of U at Prominent Hill, and oxidised sulphur as sulphuric acid. Fluid B is inferred to have contributed limited sulphur as reduced aqueous sulphide, as well as a high fraction of the total Fe that caused iron oxide deposition. Fluid C contributed some Cu, particularly in peripheral zones with lower ore grades. This fluid-mixing scenario involves three main reservoirs of dominant ore-forming components by precipitation reactions of ore minerals that additionally depend on fluid-rock reactions for acid-neutralising host-rock strata. At the Prominent Hill deposit, the highest copper grades are found at the transition between the most intense hematite-quartz alteration front (Al removal) and hematite-chlorite-sericite altered zones. In these zones, fluid A was neutralised and reduced by reaction with carbonates, aluminosilicates and the Fe2+ bearing fluids B and C (and possibly also Fe2+ bearing minerals, e.g., chlorite).
Ore Deposit Formation - The following timing and processes in the development of mineralisation and ore at Prominent Hill are proposed, modified after Schlegel et al. (2018):
• Deposition of the Main Host Sequence after ~1750 Ma (possibly nearer 1600 Ma) in an extensional setting, including precursors to the main Gawler Range Volcanic equivalents late in the sequence. This succession unconformably overlies the Mount Woods Domain sedimentary suite, which is interpreted to be equivalent to the 1765 ±27 to 1741 ±1 Ma the Wallaroo Group. The latter was intruded by the ~1691±25 Ma Engenina Adamellite;
• Deposition of the Gawler Range Volcanics equivalents ('Neptune Volcanics') and coeval intrusion of the anorogenic Hiltaba equivalent 1584 ±18 Ma Balta Granites and crust contaminated mantle related 1562 ±14 Ma (U-Pb zircon; Allen et al., 2016) White Hill Igneous Complex and similar 1587±3 Ma (U-Pb zircon; Jagodzinski, 2005) leucogabbro near Peculiar Knob. The underlying mantle lithosphere probably had retained fertility from subduction during the ~1850 Ma Cornian Orogeny when the Donington Suite granitoids were intruded, and during the 1760 and 1739 Ma Kimban Orogeny when the South Australian/Gawler and North Australian (including the Curnamona Province) cratons were amalgamated. The 'Hiltaba age' igneous activity was accompanied by south vergent reverse faulting along structures that were precursors of the Hanging-wall Fault and Southern Overthrust. The resultant uplift to the north led to the deposition of coarse clastics along the fault front. This compressional event was probably the result of transpression and led to basin inversion, and tilting to near vertical of the sequence immediately to the south of the fault. Fluid B was expelled by the mafic to ultramafic White Hill Igneous Complex, possibly the result of mingling with basement felsic gneisses, Engenina Adamellite or Balta granites. This hypersaline fluid, with a high fraction of total Fe2+, but limited sulphur as reduced aqueous sulphide, led to the development of Stage I regional iron oxide-alkali (sodic and potassic) alteration, magnetite>pyrite ±weak chalcopyrite mineralisation, and large disseminated to massive magnetite ±pyrite bodies (e.g., Manxman and Joe's Dam).
• Economic mineralisation developed during the late stages in the extrusion and deposition of the Gawler Range Volcanics. Mineralisation at Prominent Hill has been dated between ~1585 and 1570 Ma (49Ar/39Ar phengitic muscovite associated with mineralisation; Bowden, 2017). Late volcanism produced comparatively water-poor but hot magmas that released metal-, SO2-, F- and volatile-rich volcanic gases, vapours and fluids into the oxidising environment close to the surface as shallow-venting to subaerial volcanic discharges. These emissions mixed with oxygen-rich meteoric water, most likely in an acidic volcanic lake environment. Such strongly acid (sulphuric and hydrofluoric) fluid is envisaged to have aggressively leached Cu and U from unconsolidated porous tuffs, eruption rubble in the vent, subaerial brecciated and vughy lavas and wall rocks of lakes and rivers (Christenson and Wood, 1993) to form Fluid A. Alternatively, a hot, strongly acidic, F- and Cu-rich magmatic component of Fluid A may have separated from, and accumulated in the apex of a large parental mafic to ultramafic magma chamber (as indicated by magnetotelluric and deep seismic data; Reid, 2019), deep in the crust and followed a transcrustal structure to near surface to mingle with a cool, strongly oxidised brine in a shallow, volcanic fed red-bed basin, to form Fluid A. Whichever the origin, this heavy, cooling, metal-rich, acidic and oxidised, but relatively low salinity Fluid A is envisaged as having migrated downward due to brine reflux, traversing oxidised aquifers, and continuing to leach metals. Fluid circulation is also interpreted to have in part been been driven by topography (Schlegel et al., 2018). The aquifers traversed were presumably porous ignimbrites and tuffs and structures within the Gawler Range Volcanics, as well as the coarse oxidised red-bed units near the base of the volcanic pile, and the coarse clastic rocks below the unconformable base of the volcanic sequence (Bull et al., 2015).
In the first option, to source the amount of Cu in the resource at Prominent Hill of 280 Mt @ 1% Cu, i.e., 2.8 Mt of Cu, from rhyolitic and basaltic Gawler Range Volcanics containing ~50 and ~100 ppm Cu respectively, averaging 75 ppm Cu, it would be necessary to completely leach an ~13.5 km3 volume of aquifer (e.g., a large ignimbrite sheet such as the 30 km3 Taupo Ignimbrite in New Zealand) and efficiently focus the flow of pregnant fluid to and through the Prominent Hill deposit site. To account for loss and surrounding low grade envelopes, etc., a larger volume would need to be leached. If the alternative source is a segregated fluid derived from a very large volume (hundreds of km3) mafic to ultramafic magma chamber at depth, the supply of this amount of copper is far less problematic.
It is suggested that as Fluid A sank further below the surface, its temperature was elevated by heat from underlying igneous activity, thus reducing its density, and promoting upward flow, preferentially via the steep overturned limb of the calcareous Prominent Hill Main Host Sequence which had a permeability structure facilitating efficient fluid infiltration. In this limb, it encountered similarly upward percolating Fluid B which continued to emanate from the White Hill Igneous Complex or similar underlying deeper intrusions, as well as Fluid C resident in pores within the sequence. This resulted in Stage II mineralisation reactions (see the 'Introduction of Sulphides' section above) between the Cu2+ and HSO4- carried by Fluid A; Fe2+ in Fluid B; and the carbonates of the Main Host Sequence wallrocks to precipitate bornite and hematite via sulphate reduction, as follows (Schlegel et al., 2018):
5 Cu2+ + 4 HSO4- + 37 Fe2+ + 40 CaCO3 → 2 Cu5FeS4 + 18 Fe2O3 + 40 Ca2+ + 40 CO2 + 2 H2O. Comparable reactions created chalcopyrite and chalcocite, also accompanied by hematite deposition. Similarly the second Stage II reaction, pyrite replacement, occurred as a result of reaction between Cu in Fluid A, and pyrite and carbonate in the host rock to precipitate chalcocite and hematite (and bornite similarly), as follows (Schlegel et al., 2018):
28 Cu2+ + 10 FeS2 + 21 CaCO3 + 3 H2O → 14 Cu2S + 10 Fe2+ + 6 HSO4- + 21 Ca2+ + 21 CO2. Limited studies undertaken in sections of the deposit suggest that precipitation of Cu sulphides via pyrite replacement, dominates over that from sulphate reduction (Schlegel et al., 2018).
The reactions between Fluids A and B within each of the two equations in the paragraph above involve the generation of significant H+ in the first part of the reaction (e.g., in the sulphate reduction equation):
5 Cu2+ + 4 HSO4- + 37 Fe2+ + 38 H2O → Cu5FeS4 + 18 Fe2O3 + 80 H+.
This H+ is then neutralised by the reaction in the second part of the amalgamated equation which 'decalcifies' the host sequence carbonates and provides space for the deposition of Cu-(Fe) sulphides and hematite from sulphate reduction equation above, i.e.,
2 H+ + CaCO3 → Ca2+ + CO2 + H2O.
The excess Ca2+ is released to the mixed fluids or combines with the F in Fluid A to precipitate fluorite. The acid neutralisation process leads to replacement of the abundant carbonates by hematite while precipitating Cu-(Fe) sulphides. The molar volumes of the CaCO3 removed and Fe2O3 deposited in its place are similar, and hence the reaction does not occlude porosity or (presumably) permeability, but may allow the focusing of fluid flow and sulphide precipitation, thus further facilitating the alteration reaction. Given that HSO4- occurs on opposite sides of the coeval Stage II sulphate reduction and pyrite replacement reaction equations above respectively, little additional acid is required to replace large amounts of carbonate by hematite, and the excess acidity in Fluid A is available to replace further carbonates and feldspars and subsequently other aluminosilicates (e.g., micas), thereby continuing to enhance porosity and permeability while still precipitating hematite (Schlegel et al., 2018).
When the reactive carbonate has been exhausted in the host, or volcanic and/or alumino-silicate containing clastic host rocks dominate, the H+ generated by the continuing reaction of Fluids A and B creating Cu-(Fe) sulphide-hematite is neutralised by the alteration of alkali feldspars (e.g., K feldspar) to phyllosilicates (e.g., muscovite) by reactions such as:
3 KAlSi3O8 + 2 H+ → KAl3Si3O10(OH)2 + 6 SiO2 + 2 K+. As this process continues, aluminosilicate destruction progresses towards the hematite-quartz front to generate kaolinite from muscovite: 2 KAl3Si3O10(OH)2 + 2 H+ + 3 H2O → 3 Al2Si2O5(OH)4 + 2 K+
and then progresses to release silica and remove Al from kaolinite and combine with the co-precipitated hematite that continues to accumulate and produce, in some instances, almost complete replacement of the host by 'steely' hematite-quartz alteration assemblage: Al2Si2O5(OH)4 + 6 H+ → 2 SiO2 + 2 Al3+ + 5 H2O.
The 'steely' hematite-quartz alteration zone at Prominent Hill represents the most intense hematite alteration in the mineralised system, reflecting the maximum flux of reacting Fluids A and B, and the locus of the most complete alteration of host rocks. This implies it marks the source direction from which the fluids emanated. The boundary between the strongly oxidised intense hematite-quartz ±barite alteration and the more reduced hematite-aluminosilicate alteration marks an advancing redox front, where the oxidised and extremely acidic ore fluid became reduced and neutralised by ferrous iron from magmatic-hydrothermal or ambient basement fluids and by reaction with chlorite- and carbonate-rich host rocks and formation waters contained within them. Copper is strongly soluble in hot, oxidised (high Eh) and acid (low pH) fluids, and consequently remained in solution in the 'steely' hematite-quartz zone, but was precipitated on passing the redox front into the hematite-aluminosilicate zone where higher Eh and pH conditions prevailed. Cu is virtually absent within the 'steely' hematite-quartz zone, while the highest grade Cu is found in the hematite-aluminosilicate zone adjacent to the redox front where chalcocite is the dominant Cu sulphide. The Cu remaining in the outflowing fluid that had progressively increasing Eh and pH values was precipitated at progressively lower grades and lower Cu:S ratios (i.e., chalcocite → bornite → chalcopyrite-pyrite), to an outer zone of earthy hematite with sulphide veining. This outward decrease in Cu tenor corresponded to a decrease in alteration intensity from kaolin → mica → mica-feldspar → feldspar-mica. As mineralisation progresses, so does the 'steely' hematite-quartz to hematite-aluminosilicate redox front, with Cu being re-disolved from behind and overprinting and reinforcing the grade ahead of the front, as well as the width of the mineralised zone increasing outward from the front. Gold, with differing solubility properties is deposited on the 'steely' hematite-quartz side of the redox front. The location of the deposit was strongly dependent upon a confining corridor of permeable and porous, reactive host rock, namely a pre-existing, carbonate rich sedimentary breccia, into which the fluids could be focused.
Production, Ore Reserves and Mineral Resources
Global reserves and resources at the deposit as of May 2010 were estimated to be:
278.8 Mt @ 0.98% Cu, 0.75 g/t Au, 2.5 g/t Ag.
The declared Ore Reserves and Mineral Resources at Prominent Hill in mid 2008, prior to the commencement of production in 2009, were:
Copper resource - Measured + Indicated + Inferred Resources: 174.20 Mt @ 1.39% Cu, 0.56 g/t Au, 3.4 g/t Ag (0.5% Cu cut-off).
Gold resource - Measured + Indicated + Inferred Resources: 109.2 Mt @ 0.09% Cu, 1.21 g/t Au, 1.0 g/t Ag (0.5 g/t Au cut-off & <0.5% Cu).
TOTAL Resource: 283.4 Mt @ 0.89% Cu, 0.81 g/t Au, 2.48 g/t Ag.
Western Copper resource (additional) - Inferred Resources: 14.5 Mt @ 1.69% Cu, 0.28 g/t Au, 3.7 g/t Ag (0.5% Cu cut-off).
The declared Ore Reserves and Mineral Resources at Prominent Hill in June 2011 were (OZ Minerals, 2012):
Proved + Probable Reserves: 72.3 Mt @ 1.13% Cu, 0.64 g/t Au, 3.03 g/t Ag. (included within resources)
Copper resource - Measured + Indicated + Inferred Resources: 214.9 Mt @ 1.23% Cu, 0.5 g/t Au, 2.8 g/t Ag.
Gold resource - Measured + Indicated + Inferred Resources: 57.8 Mt @ 0.07% Cu, 1.5 g/t Au, 1.1 g/t Ag.
Remaining reserves and resources at Prominent Hill in June 2015 were (OZ Minerals, 2015):
Proved + Probable Reserves: 73 Mt @ 1.0% Cu, 0.6 g/t Au, 2.9 g/t Ag.
Copper-gold resource - Measured + Indicated + Inferred Resources: 152 Mt @ 1.2% Cu, 0.6 g/t Au, 2.8 g/t Ag.
Gold resource - Measured + Indicated + Inferred Resources: 27 Mt @ 0.1% Cu, 1.3 g/t Au, 1.5 g/t Ag.
Remaining Ore Reserves and Mineral Resources at Prominent Hill at 30 June 2017 were (OZ Minerals, 2017):
Ore Reserves
Open Pit - Copper
Proved + Probable Ore Reserves: 8 Mt @ 1.0% Cu, 0.6 g/t Au, 3 g/t Ag.
Underground - Copper
Proved + Probable Ore Reserves: 39 Mt @ 1.4% Cu, 0.6 g/t Au, 3 g/t Ag.
Surface stockpiles - Copper
Proved Ore Reserves: 12 Mt @ 0.8% Cu, 0.4 g/t Au, 2 g/t Ag.
Surface stockpiles - Gold
Proved Ore Reserves: 15 Mt @ 0.1% Cu, 0.8 g/t Au, 2 g/t Ag.
TOTAL Proved + Probable Ore Reserves Copper + Gold: 74 Mt @ 1.0% Cu, 0.6 g/t Au, 3 g/t Ag.
Mineral Resources - Copper (includes Ore Reserves)
Open Pit (0.25% Cu cut-off)
Measured + Indicated + Inferred Mineral Resources: 8 Mt @ 1.0% Cu, 0.6 g/t Au, 3 g/t Ag.
Underground - (AUD 57 NSR envelope cut-off)
Measured + Indicated + Inferred Mineral Resources: 120 Mt @ 1.2% Cu, 0.6 g/t Au, 3 g/t Ag.
Surface stockpiles
Measured Mineral Resources: 12 Mt @ 0.8% Cu, 0.4 g/t Au, 2 g/t Ag.
TOTAL Measured + Indicated + Inferred Mineral Resources - Copper: 140 Mt @ 1.2% Cu, 0.5 g/t Au, 3 g/t Ag.
Mineral Resource - Gold (includes Ore Reserves)
Open Pit (0.25% Cu equiv. cut-off)
Indicated + Inferred Mineral Resources: <0.5 Mt @ 1.0% Cu, 0.9 g/t Au, 1 g/t Ag.
Underground - (AUD 57 NSR envelope cut-off)
Indicated + Inferred Mineral Resources: 7 Mt @ 0% Cu, 2.4 g/t Au, 1 g/t Ag.
Surface stockpiles
Measured Mineral Resources: 15 Mt @ 0.1% Cu, 0.8 g/t Au, 2 g/t Ag.
TOTAL Measured + Indicated + Inferred Mineral Resources - Gold: 23 Mt @ 0.1% Cu, 1.3 g/t Au, 2 g/t Ag.
TOTAL Measured + Indicated + Inferred Mineral Resources - Copper + Gold: 163 Mt @ 1.04% Cu, 0.6 g/t Au, 3
g/t Ag.
Production in 2016-17 was 112 008 t of Cu metal and 3.94 t Au
For more detail see the references listed below.
The most recent source geological information used to prepare this decription was dated: 2020.
Record last updated: 19/11/2021
This description is a summary from published sources, the chief of which are listed below. © Copyright Porter GeoConsultancy Pty Ltd. Unauthorised copying, reproduction, storage or dissemination prohibited.
Prominent Hill
|
|
|
Belpario A and Freeman H 2004 - Common geological characteristics of Prominent Hill and Olympic Dam - Implications for iron oxide copper-gold exploration models : in Hi Tech and World Competitive Mineral Success Stories Around the Pacific Rim, Proc. Pacrim 2004 Conference, Adelaide, 19-22 September, 2004, AusIMM, Melbourne pp 115-125
|
Belperio A, Flint R and Freeman H, 2007 - Prominent Hill: A Hematite-Dominated, Iron Oxide Copper-Gold System: in Econ. Geol. v102 pp 1499-1510
|
Bowden, B., Fraser, G., Davidson, G.J., Meffre, S., Skirrow, R., Bull, S. and Thompson, J., 2017 - Age constraints on the hydrothermal history of the Prominent Hill iron oxide copper-gold deposit, South Australia: in Mineralium Deposita v.52, pp. 863-881.
|
Bull, S., Meffre, S., Allen, M., Freeman, H., Tomkinson, M. and Williams, P., 2015 - Volcanosedimentary and Chronostratigraphic Architecture of the Host Rock Succession at Prominent Hill, South Australia: in Society of Economic Geologists, 2015 Conference, Proceedings, Abstracts, 1p.
|
Carter, D., Belperio, T. and Freeman, H., 2003 - The discovery of the Prominent Hill copper-gold deposit, South Australia: in NewGenGold 2003 Conference, Case histories of discovery, Perth, Wetern Australia, November 2003, Louthean Media, Perth, Proceedings, pp. 102-116.
|
Curtis, S., Wade, C. and Reid, A., 2018 - Sedimentary basin formation associated with a silicic large igneous province: stratigraphy and provenance of the Mesoproterozoic Roopena Basin, Gawler Range Volcanics: in Australian J. of Earth Sciences v.65, pp. 447-463.
|
Fabris, A., 2022 - Geochemical characteristics of IOCG deposits from the Olympic Copper-Gold Province, South Australia: in Corriveau, L., Potter, E.G. and Mumin, A.H., (Eds.), 2022 Mineral systems with iron oxide coppergold (IOCG) and affiliated deposits Geological Association of Canada, Special Paper 52, pp. 247-262.
|
Forbes, C., Giles, D., Freeman, H., Sawyer, M. and Normington, V., 2015 - Glacial dispersion of hydrothermal monazite in the Prominent Hill deposit: An exploration tool: in J. of Geochemical Exploration v.156, pp. 10-33.
|
Freeman H and Tomkinson M, 2010 - Geological Setting of Iron Oxide Related Mineralisation in the Southern Mount Woods Domain, South Australia: in Porter T M, (Ed), 2010 Hydrothermal Iron Oxide Copper-Gold and Related Deposits: A Global Perspective PGC Publishing, Adelaide v.3 pp. 171-190
|
Hart, J. and Freeman, H., 2003 - Geophysics of the Prominent Hill prospect, South Australia: in Geophysical Signatures of South Australian Mineral Deposits ASEG Extended Abstracts, 2003:3, pp. 93-100. doi.org/10.1071/ASEGSpec12_08.
|
Hayward N and Skirrow R, 2010 - Geodynamic Setting and Controls on Iron Oxide Cu-Au (±U) Ore in the Gawler Craton, South Australia: in Porter T M, (Ed), 2010 Hydrothermal Iron Oxide Copper-Gold and Related Deposits: A Global Perspective PGC Publishing, Adelaide v.3 pp. 119-146
|
Katona, L., 2020 - Geophysical signatures of IOCG prospects in the Olympic Cu-Au Province: in Mesa Journal v.92, pp. 26-37.
|
Schlegel, T.U. and Heinrich, C.A., 2015 - Lithology and Hydrothermal Alteration Control the Distribution of Copper Grade in the Prominent Hill Iron Oxide-Copper-Gold Deposit (Gawler Craton, South Australia): in Econ. Geol. v.110, pp. 1953-1994
|
Schlegel, T.U., Wagner, T. and Fusswinkel, T., 2020 - Fluorite as indicator mineral in iron oxide-copper-gold systems: Explaining the IOCG deposit diversity: in Chemical Geology v.548, doi.org/10.1016/j.chemgeo.2020.119674.
|
Schlegel, T.U., Wagner, T., Boyce, A.J. and Heinrich, C.A., 2017 - A magmatic source of hydrothermal sulfur for the Prominent Hill deposit and associated prospects in the Olympic Iron Oxide Copper Gold (IOCG) province of South Australia: in Ore Geology Reviews v.89, pp. 1058-1090.
|
Schlegel, T.U., Wagner, T., Walle, M. and Heinrich, C.A., 2018 - Hematite Breccia-Hosted Iron Oxide Copper-Gold Deposits Require Magmatic Fluid Components Exposed to Atmospheric Oxidation: Evidence from Prominent Hill, Gawler Craton, South Australia : in Econ. Geol. v.113, pp. 597-644.
|
Tiddy, C., Zivak, D., Hill, J., Giles, D., Hodgkison, J., Neumann, M. and Brotodewo, A., 2021 - Monazite as an Exploration Tool for Iron Oxide-Copper-Gold Mineralisation in the Gawler Craton, South Australia: in Minerals (MDPI) v.11, 30p. doi.org/10.3390/min11080809.
|
Tiddy, C.J., Betts, P.G., Neumann, M.R., Murphy, F.C., Stewart, J., Giles, D., Sawyer, M., Freeman, H. and Jourdan, F., 2020 - Interpretation of a ca. 1600-1580 Ma metamorphic core complex in the northern Gawler Craton, Australia: in Gondwana Research v.85, pp. 263-290.
|
Williams, P., Freeman, H., Anderson, I. and Holcombe, R., 2017 - Prominent Hill copper-gold deposit: in Phillips, G.N., (Ed.), 2017 Australian Ore Deposits, The Australasian Institute of Mining and Metallurgy, Mono 32, pp. 611-614.
|
Williams, P.J., Benavides, J., Sadikan, P., Schlegel, T. and OZ Minerals Prominent Hill Geology Team, 2017 - Metallogenic Significance of Altered Volcanic Rocks Near the Prominent Hill IOCG Deposit, South Australia: in Mineral Resources to Discover, Society for Geology Applied to Mineral Deposits (SGA), 14th Biennial Meeting, Quebec, Canada, August 20-23, 2017, Proceedings, v.3, pp. 895-898.
|
Porter GeoConsultancy Pty Ltd (PorterGeo) provides access to this database at no charge. It is largely based on scientific papers and reports in the public domain, and was current when the sources consulted were published. While PorterGeo endeavour to ensure the information was accurate at the time of compilation and subsequent updating, PorterGeo, its employees and servants: i). do not warrant, or make any representation regarding the use, or results of the use of the information contained herein as to its correctness, accuracy, currency, or otherwise; and ii). expressly disclaim all liability or responsibility to any person using the information or conclusions contained herein.
|
Top | Search Again | PGC Home | Terms & Conditions
|
|