Gawler Craton and Olympic Iron Oxide Copper Gold Uranium (IOCG) Province |
|
South Australia, SA, Australia |
Main commodities:
Cu Au U REE Fe
|
|
 |
|
 |
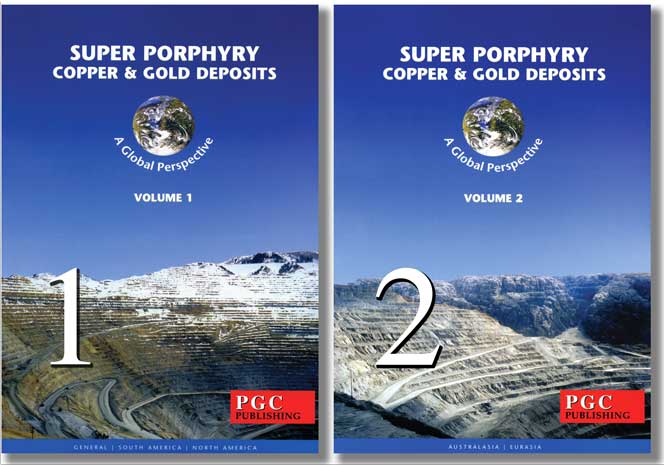 |
Super Porphyry Cu and Au

|
IOCG Deposits - 70 papers
|
All papers now Open Access.
Available as Full Text for direct download or on request. |
|
 |
The Archaean to Palaeoproterozoic Gawler Craton, which extends over an area of around 700 km east-west by 800 km north-south in central South Australia, hosts the giant Mesoproterozoic Olympic Dam (Cu-U-Au-Ag-REE), Prominent Hill (Cu-Au), Cairn Hill (Fe and Cu-Au-Fe), Carapateena, Fremantle Doctor and Khamsin, Oak Dam West, (Cu-Au) and Hillside (Cu-Au), deposits, and the historic Wallaroo-Kadina-Moonta deposits, all of which are IOCG systems, as well as Au occurrences and other deposits in the western part of the craton which are embraced by regional iron oxide alteration systems. In addition, there are numerous iron oxide (magnetite±hematite) deposits with varying amounts of associated Cu (from virtually none to ~0.3%, but locally >0.5%) and gold. These include Wirrda Well, Acropolis, Titan-Bopeechee and Vulcan,
Manxman,
Oak Dam East,
Emmie Bluff, Murdie Murdie and
Island Dam.
The craton also hosts the early Palaeoproterozoic Challenger Au deposit, Ag-Pb-Zn mineralisation (eg. Menninnie Dam), epithermal Ag (e.g., Paris), the Palaeoproterozoic banded iron formation iron ore of the Middleback Ranges and the Early Palaeoproterozoic Warramboo gneiss hosted magnetite deposit in the eastern and central Eyre Peninsula of the south-central parts of the craton.
Cratonic Setting
The Gawler craton is the major crustal province of the southern Australian Proterozoic. Its evolution is dominated by two major phases of tectonic activity, in the Meso- to Neoarchaean and late Paleoproterozoic to early Mesoproterozoic, spanning a period of nearly 2 billion years.
The oldest rocks of the Gawler craton comprise Mesoarchaean to early Palaeoproterozoic metamorphics and igneous suites that form a core to the craton. The Mesoarchaean of the Gawler Craton is dominated by felsic magmatism, including the ~3400 to 3150 Ma Cooyerdoo Granite and underlying TTG basement, followed by further felsic magmatism represented by the ~2820 Ma Coolanie Gneiss. In contrast to this predominantly felsic magmatism the Neoarchaean to Palaeoproterozoic is characterised by sedimentation and bimodal volcanism, whilst the Mesoproterozoic includes both bimodal (Hiltaba) and felsic volcanism further west (SA Geological Survey website,viewed Nov 2021).
The late Archaean to earliest Palaeoproterozoic, from ~2.56 to 2.47 Ga, was a period of basin development, represented by the Sleaford and Mulgathing complexes in the southern and northern Gawler Craton respectively. The two probably represent a single late Archaean belt. Arenaceous and aluminous metasediments, banded iron formation and carbonates (Carnot Gneiss, Wangary Gneiss, Christie Gneiss and Kenella Gneiss) were deposited coeval with arc-like felsic magmatism and plume related mafic-ultramafic magmas, including the Lake Harris Komatiite. These volcano-sedimentary packages were intruded by felsic to intermediate magmas (Glenloth Granite and Dutton Suite) derived from contaminated mantle melts. Maximum depositional ages of sedimentary rocks in the Middleback Ranges of ~ 2.56 Ga within and directly south of the Middleback Ranges, suggest sedimentation also occurred in the Neoarchaean in the NE Eyre Peninsula, but the relationship of these sediments to the Sleaford and Mulgathing complexes is uncertain. Deposition of these sequences and intrusive emplacement was followed by the Sleaford Orogeny compressional deformation from 2.48 to 2.42 Ga and then by ~400 m.y. of tectonic quiescence, probably within an early Palaeoproterozoic continental interior. This quiescence was interrupted by the intrusion in central Eyre Peninsula of the Miltalie Gneiss, which has high-grade migmatitic fabrics, grading westward to a quartzofeldspathic gneiss, derived from a granodiorite protolith. Synchronously, in the southern Eyre Peninsula, the Red Banks Charnockite and other unnamed charnockitic granites were intruded into the Sleaford Complex (SA Geological Survey website,viewed Nov 2021).
This older nucleus was overlain after ~2000 to near 1730 Ma by the Hutchison Group, a sequence of subaerial to shallow marine clastic and chemical metasedimentary rocks (including BIFs) with minor bimodal felsic and mafic volcanic rocks, that were initially deposited as a series of rift-related basins, and on a continental passive margin (Parker, 1993; Daly et al., 1998). Other similar units in this period and setting included the Broadview Schist and Myola Volcanics, Moonabie Formation and McGregor Volcanics, Wallaroo Group, Peake Metamorphics, Price Metasediments, and unnamed sediments in the Nawa and Fowler Domains and Mt Woods inlier. Along the eastern margin of the craton, both the cratonic core and the Hutchison Group and related sequences were metamorphosed and intruded by batholith-scale granitic magmatism, represented by the ~1850 Ma granitoids of the Donington Suite during the Cornian Orogeny, a short-lived contractional deformation event (Hand et al., 2007; Reid et al., 2008). This suite, partially derived from anatexis of the late Archaean basement, is dominated by granodiorite gneiss, with subordinate metamorphosed alkali-feldspar granite, gabbronorite, tonalite and quartz monzonite intrusions with mafic dykes (Ferris et al., 2002).
The eastern Gawler Craton in South Australia contains a major lithospheric boundary, interpreted to be a palaeo-suture (Hayward and Skirrow, 2010; Betts et al., 2015). The precise location of this boundary is uncertain in the north where it is masked by thick younger cover, although it appears to spatially coincide with the western boundary of the Olympic Cu-Au-U Province. To the south, where not covered, this suture coincides with the Kalinjala Shear Zone, a major 4 and 6 km wide Palaeoproterozoic-aged mylonitic structure along the southeastern coast of Eyre Peninsula (Vassallo and Wilson, 2002). Betts et al. (2015) argue that the suture initially formed at ~1850 Ma during the Cornian Orogeny when the Gawler Craton was accreted to the North Australian Craton, which also included what was to become the Curnamona Block. They further suggest that the South Australian/Gawler and North Australian Craton were then rifted apart during a period of extension and basin development that lasted until ~1740 Ma, and that the two terranes were then finally re-sutured during the 1730 to 1690 Ma Kimban Orogeny. Curtis and Thiel (2019) suggest that on the basis of Nd, Hf in zircon and inherited zircon ages of felsic magmatic rocks, as well as magnetotelluric and deep seismic data, that the Roopena Fault is the northern extension of the Kalinjala Shear Zone and represent a palaeo-suture between the Mesoarchean cratonic core of the Gawler Craton to the west, and a Neoarchean-Palaeoproterozoic terrane to the east. It marks a major lithospheric boundary, characterised in magnetotelluric modelling, by the interface between resistive and conductive lithosphere. They suggest the conductive lithosphere may represent upper mantle and lower crust fertilised by arc-related magmatism during a subduction event, most likely part of the Kimban Orogeny. The major faults and shear zones formed during the Kimban Orogeny are part of an east-verging fold and thrust belt extending into the lower crust. These faults and shear zones are imaged as conductive features in 2D resistivity modelling (Curtis and Thiel, 2019).
Between ~1770 and 1740 Ma, subsequent to the emplacement of the Donington Suite, extension along the eastern, northern and western margins of the craton, resulted in the development of a series of extensive basins, as mentioned above, during the post-Cornian rifting of the North Australian and Gawler cratons. Some of these basins contain bimodal volcanic rocks, including the Wallaroo Group, which is extensively developed within the Olympic IOCG Province on the eastern margin of the preserved craton, and is an important host to IOCG alteration and mineralisation. Further west, between 1680 and 1640 Ma, sedimentation in these extensional basins included the Tarcoola Formation and Corunna Conglomerate, as well as the ~1690 to 1680 Ma and felsic magmatism of the Tunkillia Suite (SA Geological Survey website,viewed Nov 2021).
During the Palaeoproterozoic, the Curnamona Province, then part of the North Australian Craton, is interpreted to have collided with and been accreted to the Gawler Craton in the east, as described above. A major linear discontinuity in magnetic and gravity data beneath the Neoproterozoic cover of the Adelaide Geosyncline intracratonic rift complex is interpreted to mark the suture zone (Hayward and Skirrow, 2010, and sources quoted therein) although Betts et al. (2015) suggest the same suture is further west as described above. The interval between ~1730 and ~1630 Ma encompasses the Kimban Orogeny (1730 to 1690 Ma), Ooldean Event (1660 to 1630 Ma), and the widespread emplacement of various felsic igneous rocks, and formation of several small intracontinental sedimentary basins (Hand et al., 2007).
Significant basin development had been terminated by the Kimban orogeny, which produced widespread crustal-scale shear systems, granitic magmatism, and low- to high-grade metamorphism.
A subsequent renewed period of extension from 1.68 to 1.64 Ga, led to local magmatism and sedimentation which in part appears to have been coincident with the high temperature-low pressure and high metamorphic grade Ooldean Event in the western Gawler craton.
At around 1.65 to 1.63 Ga, the St. Peter Suite of intrusions and coeval extrusive rocks were emplaced over a widespread area on the south-western Gawler craton. The St. Peter Suite is bimodal and covers a large equi-dimensional area rather than a linear belt. This association includes significant volumes of mafic to ultramafic rocks of the ~1.635 Ga Andrew Young Event (e.g., Hoatson et al., 2008). These were followed by the extrusion of the voluminous 1.595 to 1.590 Ga Gawler Range Volcanics and coeval 1.595 to 1.575 Ga Hiltaba Suite granitoids, the latter of which is dominated by mantle related high-T fractionated felsic rocks with coeval mafic magmatism confirming a mantle involvement.
The emplacement of the Hiltaba Suite intrusives coincided with the major mineralising event of the Gawler craton, producing both i). the approximately 700 km long Olympic IOCG province on the eastern margin of the craton, extending from the Peake and Denison Ranges in the north to the Moonta-Wallaroo district in the south, and ii). the gold-dominated central Gawler gold province further west. The spatial distribution of IOCG versus Au-dominated mineral systems has been interpretted to reflect regional variations in crustal composition and Hiltaba Suite petrogeneses. In the Olympic IOCG province, the Hiltaba-aged granites are more evolved, richer in U and Th, and oxidised in comparison with similar aged granites in the Au province to the west.
Widespread NW-SE directed contractional deformation has been interpreted at the time of emplacement of the Hiltaba Suite formed and/or reactivated shear zones from local to crustal scale, with NW-trending structures having accommodated dilation associated with strike-slip movements. Intersection of these structures appears to have formed structural traps for 1.59 to 1.58 Ga mineralisation, occurring as iron oxide copper-gold, gold and silver deposits across the craton, and is the single most important mineralising event within the craton. Haywood and Skirrow (2010) infer the IOCG mineralisation was most likely mostly formed during the late stages of a short lived episode of NNW-SSE extensional strain that coincided with eruption of the Gawler Range Volcanics between ~1595 and 1590 Ma, and the development of ENE-trending grabens, including those near the Prominent Hill and Olympic Dam deposits. They suggest however, this contraction was preceded and closely followed by more protracted NW-SE to NNW-SSE contraction during the broader episode of Hiltaba Suite pluton emplacement between 1600 and 1575 Ma (Hand et al., 2007). Widespread NW-SE directed contractional deformation at the time of emplacement of the Hiltaba Suite formed and/or reactivated shear zones from local to crustal scale, with NW-trending structures having accommodated dilation associated with strike-slip movements. Intersection of these structures has been interpreted to have formed structural traps for 1.59 to 1.58 Ga mineralisation, occurring as iron oxide copper-gold, gold and silver deposits across the craton, and is the single most important mineralising event within the craton. The timing of syn-Hiltaba deformation overlaps the transpression and medium- to high-grade metamorphism of the 1.57 to 1.54 Ga Kararan Orogeny in the central northern part of the craton. The youngest deformation in the craton is seen as the reactivation of shear zones from 1.47 to 1.45 Ga and regional cooling.
While the interpretations above emphasise a period of dominant compression during the Hiltaba Event, Tiddy et al. (2020) have demonstrated that the Mount Woods Domain (MWD), immediately to the north of Prominent Hill, represent a partially preserved metamorphic core complex that developed during the ~1600 to 1580 Ma Hiltaba Event. The lower plate of the complex predominantly comprise the Mount Woods Metamorphics which have a maximum detrital zircon depositional age of ~1860 Ma and were polydeformed and metamorphosed to upper amphibolite to granulite facies during the ~1740 to 1690 Ma Kimban Orogeny. These metamorphic rocks cover a WNW-ESE elongated area of ~60 x 30 km. To the south, the upper plate is composed of a younger succession, which includes metasediments that were deposited at ~1750 Ma. Subsequently, sedimentary and volcanic successions of the Gawler Range Volcanics were deposited into half grabens that evolved during brittle normal faulting of the upper plate caused by the updoming that resulted from the rise of the lower plate. This upper plate brittle deformation is underlain by a major basal detachment fault separating the upper and lower plate of the core complex. The geometry of normal faulting in the upper plate is consistent with NE-SW extension. Both plates were intruded by ~1795 to 1575 Ma Hiltaba Suite granitic and mafic plutons. Extensive modification of the core complex occurred during the ~1570 to 1540 Ma Kararan Orogeny which is characterised by shear zone formation. Exhumation had commenced along the Southern Overthrust at ~1592 to 1582 Ma (Forbes et al., 2012), and was likely further accommodated by Kararan-aged faults. Exhumation continued until ~1540 Ma, likely accommodated by the Southern Overthrust and possibly by continued activity along the Skylark and Panorama Fault Zones (Tiddy et al., 2020). These observation suggest the Hiltaba Suite intrusives and Gawler Range Volcanics were emplaced under a NE-SW directed extensional regime, rather than NW-SE directed transpression. As such, the extensional interval associated with mineralisation envisaged by Haywood and Skirrow (2010) may have been more than a short interregnum. For more detail on this metamorphic core complex, see the Prominent Hill record.
A late episode of metamorphism and magmatism at 1463 to 1444 Ma has been identified in part of the Nawa Domain in the northern to northwestern Gawler craton. It is reflected in unfoliated granites and strongly foliated, migmatitic garnet-biotite bearing gneiss that present evidence for partial melting. Two samples investigated suggest peak conditions of 815 to 775°C and pressures of 3.2–5.4 kbar, corresponding to high apparent thermal gradients of >45°C/km, interpreted to reflect pluton-enhanced metamorphism, consistent with associated coeval A-type granites. A monazite age of 1444±10 Ma, from this gneiss is interpreted to date the timing of granulite facies metamorphism (Morrissey et al., 2018). Although these results only directly relate to a small number of drill holes that have penetrated the almost complete cover of the Nawa Domain, they appear to represent a distinct, ~1450 Ma thermal event in at least part of the domain. These intrusives have previously been interpreted as being part of the Hiltaba suite. There is no evidence that this event is more widespread. However, elsewhere in the Gawler Craton, there are indications of a possible extensional regime at ~1500 to 1400 Ma, most notably with deposition of the Pandurra Formation in the Cariewerloo Basin (Beyer et al., 2018). The Pandurra Formation comprises unmetamorphosed, dominantly fluvial red-bed sediments that include medium- to coarse-grained, poorly sorted, subangular quartz and lithic sandstone with minor conglomerate, mudstone, siltstone. The sandstones are characterised by large-scale cross-bedding and liesegang banding (Geoscience Australia, Australian Stratigraphic Units Database). It is interpreted to have been deposited as part of a continental rift system after ~1490 Ma (Beyer et al., 2018). Following deposition, fluid flow events occurred at ~1450 to 1420 Ma within the Pandurra Formation (Cherry et al., 2017; Beyer et al., 2018). Lithospheric-scale shear zones in the Gawler Craton, including the Karari, Tallacootra, Coorabie and Kalinjala shear zones, were active/re-activated between 1470 and 1450 Ma (Foster and Ehlers 1998; Swain et al., 2005; Fraser and Lyons 2006; Thomas, Direen and Hand 2008; Fraser, Reid and Stern 2012), confirming the presence of regional deformation in this interval (Morrissey et al., 2018). Consequently, it is possible the high metamorphism in the Nawa Domain, reactivation of crustal-scale shear zones across the craton and the formation of a significant, intracontinental sedimentary basin in the central-eastern Gawler Craton are all part of a coherent tectonic regime dominated by extension (Morrissey et al., 2018).
Extension and sedimentation, related to the break-up of the late Neoproterozoic Rodinia supercontinent, produced the Adelaide Fold Belt rift basin that defines the eastern margin of the preserved Gawler craton, and separates it from the Curnamona Province that was amalgamated with the Gawler craton during the Palaeo- and Mesoproterozoic. Several hundreds off metres of shelf sediments fringing the thick rift basin lap onto the cratonic domains on either side.
Regional setting of the Olympic Dam IOCG province - The tectonic framework and location of the main iron oxide-alkali altered mineralisation of the combined Mesoarchaean to Mesoproterozoic Gawler Craton and Curnamona Province of South Australia. Note the central Archaean to Early Palaeoproterozoic nucleus, bounded in part by the Elizabeth Creek Fault and Kalinjala Mylonite Zone. The Neoproterozoic Adelaide Geosyncline rift basin (related to Rodinia break-up extension) masks the mid- to late-Palaeoproterozoic suture between the Gawler craton and Curnamona Province. The northeastern, eastern and southeastern margins of the preserved craton are determined by the Tasman Line, which marks the Rodinia break-up and separation of the eastern sections of the cratonic mass, while the southern margin is the Pangea break-up across which cratonic remnants now in Antarctica were separated. Prior to the break-up of Rodinia, the Gawler craton, Curnamona Provinces, and cratonic elements in North America (?) and Antarctica were all part of the larger Mawson craton. The northern and western margins are collisional with the North Australian (~1560) and Yilgarn crustal elements (~1350 to 1260 and ~1210 to 1140 Ma) respectively. This emphasises the internal location of the Olympic IOCG Province within the Mawson craton at the time of its formation. Note also the temporal progression of the centre of magmatism across the interior of the craton from ~1630 to ~1550 Ma, over 300 km from the nearest craton margin to the northwest. The youngest magmatic-metamorphic event recorded is ~1450 Ma in the northwestern part of the craton, to the north and NW of the Hiltaba suite plutons. The Mafic/Ultramafic Magmatism inset (top-centre), shows the envelopes surrounding regions of mafic to ultramafic dykes, sills, stocks and layered complexes (after Claoué-Long and Hoatson, 2009) that define mafic igneous provinces within the craton. Note that the diachronous progression from the 1630 to 1615 Ma St Peter, to the 1600 to 1575 Ma Hiltaba and 1580 to 1550 Ma Bimbowie Suites is reflected in mafic igneous province ages. The relationship between the older north-south 1780 to 1750 Ma group (immediately west of the mid Palaeoproterozoic Curnamona-Gawler suture) and the gabbros of the Pine Point structural corridor (assumed to be of the same Hiltaba age as the Curramulka gabbronorite immediately to their west) is of interest. Diagram after Hayward and Skirrow (2010), Conor et al. (2010), Claoué-Long and Hoatson (2009) and others quoted therein).
Olympic IOCG Province
The significant IOCG sensu stricto deposits on the eastern margin of the preserved Gawler craton define the Olympic IOCG Province (see diagram). These include Olympic Dam (Hayward and Skirrow, 2010; Reynolds, 2000; Reeve et al., 1990), Prominent Hill (Freeman and Tomkinson, 2010; Belperio et al., 2007), Carrapateena (Porter, 2010; Vella and Cawood, 2006) and Hillside (Conor et al., 2010).
The emplacement of these deposits has been interpreted to be temporally associated with the ~1.59 Ga Hiltaba Suite granitoids (and the co-magmatic bimodal Gawler Range Volcanics - GRV). The Hiltaba Suite are dominantly a highly fractionated granite to granodiorite (>70% SiO2), with accompanying minor, but spatially widespread coeval mafic to ultramafic intrusions (Creaser, 1989, 1996; Stewart and Foden, 2003; Hand et al., 2007). Syn-mineralisation mafic dykes within the Olympic Dam deposit are interpreted to belong to the latter, although these may represent a late phase, marginally post-dating the main felsic Hiltaba Suite (Chambefort et al., 2009; McPhie et al., 2010) whereas other post-ore mafic intrusions may belong to the 825 Ma Gairdner Dyke Swarm. One of the largest of the mafic to ultramafic intrusions in the Olympic IOCG Province is the composite White Hill Complex in the Mount Woods domain, a few kilometres north of the Prominent Hill deposits, which comprises layered pyroxenite, norite and gabbro covering an area of ~200 km2, and is apparently coeval with Hiltaba Suite magmatism in that domain (Belperio et al., 2007). Similarly, mafic and ultramafic intrusions are known in the Olympic Dam district (Johnson and Cross, 1995; Jagodzinski, 2005; Skirrow et al., 2007). Gabbroic intrusives of the regional Pine Point Fault structural corridor with which the Hillside deposit is associated, and the gabbro and granitic intrusions within the Hillside ore zone, are assumed to also be of Hiltaba Suite age, on the basis of structural and textural comparison with those dated elsewhere in the district (e.g., the 1589±5 Ma Curramulka Gabbronorite; Conor et al., 2010; Zang, 2003). The latter is supported by zircons from the deposit that yielded a range of SHRIMP U-Pb ages between ~1580 and ~1560 Ma (Ried et al., 2010).
The GRV commence with a lower 0.5 to 3 km thick sequence of texturally and compositionally varied volcanic units that are gently to moderately dipping (10 to 30°) and best exposed to the west and south. These lower units range in composition from basalt to rhyolite and comprise lavas, ignimbrites and other minor volcaniclastic facies, with tholeiitic basalt and andesite being subordinate (~10 vol.%) to dacite and rhyolite (Allen et al., 2009). However, the lower Gawler Range Volcanics do include locally thick basalt and andesite (Drexel et al., 1993), which are predominantly found to the east of the Elizabeth Creek fault (see diagram). In comparison, the upper GRV comprises widespread, very gently dipping (<5°), thick (250 to 300 m), crystal-rich (15 to 40%), rhyolitic and dacitic units considered to be lavas. The GRV have been intruded by porphyritic rhyolitic dykes and granites. Juxtaposition of granites with the GRV suggests that at least 1 km of the GRV and/or associated sedimentary rocks have been eroded (Allen et al., 2009). Creaser and White (1991), and Stewart (1994), showed that the magmas that fed the GRV had a high temperature (900 to 1100°C) and were relatively dry (<2 wt.% H2O). Stewart (1994) also recognised that the dissolved volatiles in these magmas included significant halogens, particularly fluorine (up to 1.3 wt.%). This high fluorine content would significantly reduce the normally high viscosity of the felsic lavas, to allow them to erupt effusively and form extensive lava sheets (Allen et al., 2009). Petrogenetic studies suggest that the mafic magmas, though subordinate in volume, were derived from the mantle and that the silicic magmas were generated by fractional crystallisation of the mafic parent, and in some cases, melting and assimilation of Archaean or Palaeoproterozoic crust (Giles, 1988; Stewart, 1994; Creaser, 1995).
The Hiltaba Suite is composed of both A- and I-type granitoids, with the former dominating in the Olympic IOCG Province (see diagram) which hosts all of the major IOCG deposits listed above) in the east, and I-type in the central and western Gawler craton (Skirrow et al., 2007), with more S-type in the Curnamona Province to the east (Skirrow, 2009).
The Olympic IOCG Province spatially coincides with, and overprints, an accreted Palaeoproterozoic terrane of <2000 to 1740 Ma metasedimentary and metavolcanic rocks that is bounded to the west by the Mesoarchaean to early Palaeoproterozoic nucleus of the Gawler craton (see diagram). Its eastern limit is concealed below the north-south trending Neoproterozoic Adelaide Geosyncline intracratonic rift, and corresponds to the suture with the contiguous Curnamona province, which is believed to have docked with the Gawler craton between 1.9 and 1.8 Ga as part of the assembly of the Nuna/Columbia supercontinent (Hayward and Skirrow, 2010; Conor et al., 2010). Subduction related to this collision could have resulted in mantle enrichment in volatiles below what was to become the Olympic IOCG Province within an essentially intracratonic terrane.
The Hiltaba Suite and Gawler Range Volcanics occupy a broadly circular to oval-shaped area with a diameter of ~500 to 600 km, a preserved area of >25 000 km2 and thickness of up to more than 3 km. It is the central and largest of a progression of three zones of magmatic activity defining a diachronous WSW-ENE trending corridor of bimodal I-, A- and subordinate S-type intrusions from the western margin of the preserved Gawler craton to the Curnamona Province (see diagram), which was by that time amalgamated with, and part of the Gawler craton. These three zones comprise the ~1630 to 1615 Ma Nuyts Volcanics and St Peter Suite bimodal magmas to the west (Fanning et al., 2007), the ~1600 to 1575 Ma intrusive Hiltaba Suite and coeval Gawler Range Volcanics, and the ~1580 to 1550 Ma intrusive Bimbowrie Suite and Benagerie volcanics (Hayward and Skirrow, 2010) to the east of the Adelaide Geosyncline in the Curnamona Province (see diagram). Each of these three continental I- and A-type intrusive suites is bimodal, showing evidence for mingling of mantle mafic melts and silicic crustal melts (Stewart, 1994; Skirrow, 2009; Swain et al., 2008). This would result in the magma chemistry varying in accordance with lithospheric composition, heat flow (i.e., temperature and depth of crustal melting), and the degree of involvement of metasomatised sub-crustal lithospheric mantle (Skirrow, 2009).
Numerous separate and collective subduction zones have been proposed to explain this distal continental magmatism, mostly associated with the distant western and northwestern margins of the craton accompanying terrane accretion events during the amalgamation of the North and South Australia cratons between 1690 and 1550 Ma (discussed in Hayward and Skirrow, 2010), although none appear to convincingly explain their geometry and composition. The eastern to southeastern and southern limits of the remaining Gawler craton-Curnamona Province are Rodinia and Pangea breakup rift margins respectively, across which cratonic elements now in North America (?) and Antarctica were separated.
The temporal association of IOCG mineralisation in the Olympic IOCG Province with the Hiltaba Suite/GRV is largely based on relationships at the Olympic Dam deposit, where the Olympic Dam Breccia Complex (ODBC), which hosts the ore deposit, cuts the Roxby Downs Granite (RDG) of the Hiltaba Suite. Reeve et al. (1990) proposed that ore formed within multiple mafic maar-diatreme volcanoes, now represented by mafic dykes, and bedded facies considered to represent phreatomagmatic ash from a maar lake. In this case, the ODBC would be related to an explosive Lower GRV mafic volcanic diatreme. However, recent drilling has revealed significant additional volumes of mafic/ultramafic derived sedimentary facies, mafic/ultramafic dykes, and isolated occurrences of basaltic lavas in the upper section of the deposit (Ehrig, 2010). Following this drilling, McPhie et al. (2010) argued that the RDG intruded into now eroded overlying comagmatic GRV, and that the bedded facies represent sediments from a more extensive basin overlying (or intercalated within) the GRV, deposited prior to the development of the ODBC. This conclusion is based on the following: (1) the downward decrease of heterolithic clasts (including GRV and sediments) in the hematitic breccias of the ODBC; (2) domains with clasts of GRV in the breccias, indicating the presence of GRV above the RDG when the ODBC was formed; (3) sedimentological features, grain composition, mixed volcanic-granitoid provenance and geochemical signatures which suggest the bedded facies are detrital rather than phreatomagmatic, and that they were deposited below wave-base in an intracontinental, fault bounded lacustrine basin prior to the formation of the ODBC. If this is the case, the bedded sedimentary facies cannot be the surficial ‘within-crater’ deposits of a diatreme. These conclusions question both the maar-diatreme origin of the ODBC, and that it represents a GRV centre of volcanism. The presence of the bedded facies and their character implies the hydrothermal brecciation that produced the ODBC propagated from a deeper site in the RDG, upwards into the GRV and overlying bedded facies sediments, most likely not breaching to the surface (K. Ehrig, pers. comm., 2011). Age determinations for the RDG, GRV and zircons from the bedded facies, all cluster around 1590 Ma (Johnson and Cross, 1995). It would therefore follow that the ODBC was formed after 1590 Ma, although most likely related to late stage hydrothermal activity associated with the same gross mantle induced event that produced the Hiltaba Suite and GRV. Alteration and mineralisation of the intensity and scale of the Olympic IOCG Province require the influence of a major crustal scale magmatic event. If not related to the Hiltaba/GRV event, the next possible major magmatism is inferred by 1169±48 Ma zircon populations, of unknown provenance, found in regional drainages across the craton (Belousova et al., 2009), and the 0.825 Ga Gairdner dyke swam magmatism (e.g., Claoué-Long and Hoatson, 2009). Both of these latter options appear less viable, as at the Oak Dam East prospect, 57 km southeast of Olympic Dam, IOCG-U mineralisation is unconformably overlain across a sharp unconformity by the unmineralised and unaltered, post 1424 Ma Pandurra Formation, which contains numerous rounded boulders and pebbles of massive iron oxide closely resembling that of the deposit (Davidson et al., 2007).
Hayward and Skirrow (2010) note that in deep seismic transect data, the 3D crustal-scale architecture of the Olympic Dam mineralised system comprises a discrete >40 km wide, elliptical, lower- to mid-crustal zone of reduced or transparent seismic reflectivity (with no associated gravity high) that has been interpreted to reflect voluminous Hiltaba-age migmatites and altered felsic batholiths. This zone is localised above a crust-penetrating fault zone at the edge of an inferred mafic underplate (see Figs. 5 and 6, in Hayward and Skirrow, 2010). This east-dipping, deep fault, which up to 5 km, broadly coincides with the implied eastern margin of the Archaean nucleus of the Gawler craton and may represent the sub-surface extent of the Elizabeth Creek fault (see diagram). Inversions of geophysical data suggest that magnetite-rich alteration extends several kilometres beneath the Olympic Dam deposit, to near the top of the interpreted batholiths.
A coincident zone of low resistivity in the mid-crust beneath the deposit, imaged in magnetotelluric data, may be associated with conductive mineral seams, probably graphite, related to this alteration event. Hayward and Skirrow, (2010) note there is little evidence for a mafic underplate in seismic reflection traverses in the Olympic Dam area (Direen and Lyons, 2007), although west of the Elizabeth Creek Fault Zone (the inferred eastern margin of the Archaean nucleus of the Gawler craton; see diagram), Huynh et al. (2001) used Bouguer gravity data to model a ~5 km thick, ~200 km diameter, high density (~3.0 g/cm3) mafic sheet at the base of the crust beneath the Gawler Range Volcanics. This sheet is interpreted to have resulted from decompression melting of ascending mantle that drove the most intense part of the 1600 to 1575 Ma crustal anatexis event that produced the Hiltaba Suite and Gawler Range Volcanics.
A national compilation and analysis of dated mafic and ultramafic magmatism has been undertaken by GeoScience Australia (Claoué-Long and Hoatson, 2009), based on both significant intrusions and areally distributed clusters of sparsely dispersed, small to moderate dykes, sills, stocks and some layered complexes (see diagram inset). This study has defined a broad, eastnortheast trending corridor that crosses the Gawler craton/Curnamona Province, composed of a series of overlapping, eastward migrating pulses centred on 1680, 1635 and 1590 Ma respectively. The last two both spatially and temporally overlap, the Nuyts Volcanics/ St Peter Suite, Hiltaba Suite/Gawler Range Volcanics, and Bimbowrie Suite/Benagerie volcanics described above, covering similar areas, and are taken to represent a period of progressive underplating below sections of the Gawler craton/Curnamona Province. No significant exposed magmatic event appears to be related to the older 1680 Ma group of mafic intrusives to the west, although the similarly aged block to the east overlaps the Broken Hill lead-zincsilver district. An older, narrower, linear belt of mafic to ultramafic magmatism follows the eastern margin of the Olympic IOCG province from Wallaroo to the Mount Woods Inlier, with pulses at 1780 and 1750 Ma, generally coincident with A-type magmatism in the Wallaroo Group (Conor et al., 2010) and the 1760 to 1739 Ma Kimban Orogeny. This locus of mafic to ultramafic intrusions is located just to the west of the inferred Mid- Palaeoproterozoic suture between the Curnamona Province and the main Gawler craton.
Regional Alteration
Although the bulk of iron oxide-alkali altered mineralised systems in the Gawler craton are interpreted to have accompanied post-1600 Ma Hiltaba Suite magmatism, the oldest known alteration has been dated at ~1620 Ma, from monazite associated with syn-deformational magnetitebiotite, and from titanite in sodic-calcic assemblages in the Moonta-Wallaroo district (Raymond et al., 2002; Skirrow et al., 2006, 2007).
In the Curnamona Province, regional-scale stratabound sodic (albitic) alteration was formed during diagenesis at ~1630 Ma (Teale and Fanning, 2000). Magnetitedominant and minor hematitic IOCG-style mineralisation associated with K-Fe alteration, and high-temperature syn-tectonic gold (copper-molybdenum), developed in the southern Curnamona Province possibly as early as 1630 to 1612 Ma (Skirrow et al., 2000; Williams and Skirrow, 2000), although re-analysis gave a Re-Os age of ~1603 Ma (Skirrow, 2009).
Post-1600 Ma, regional-scale alteration patterns are well developed within the Olympic IOCG Province. The earliest phase recognised is sodic-calcic-iron, characterised by an assemblage of albite-calc silicate ±magnetite. Actinolite, clinopyroxene (diopside or salite) with minor titanite and scapolite occur in places (Hayward and Skirrow, 2010).
This alteration is well developed in the Mount Woods Inlier to the north, and Moonta-Wallaroo district to the south (see diagram), but not generally in the Olympic Dam district (an exception being at the Oak Dam East prospect), although it may be present at depth, where large scale magnetitealteration is interpreted from geophysical modelling. This interpreted deep alteration corresponds to the elliptical zone of lower-crustal reduced or transparent seismic reflectivity above the Elizabeth Creek Fault Zone described above, interpreted in turn to reflect a zone of anatexis, comprising migmatites and felsic intrusions. For more detail consult Hayward and Skirrow (2010). A second phase of alteration, characterised by biotite-magnetite, is well represented in both the Mount Woods Inlier and Moonta-Wallaroo districts, where it shows mutually crosscutting relationships with the Hiltaba Suite, and is clearly imaged in regional aeromagnetic data which shows it to be widespread. Albite is a stable phase during this K-Fe alteration which is often accompanied by local low grade (<0.5% Cu) pyrite, chalcopyrite, pyrrhotite, monazite and titanite (Hayward and Skirrow, 2010).
A third phase, occurring as an assemblage of magnetite-K feldspar ±actinolite ±carbonate is important in the Olympic Dam district, but appears to be absent in other parts of the Olympic IOCG Province. It is locally accompanied by minor pyrite, quartz, carbonate, chalcopyrite, apatite and titanite, although these only account for generally low grade (<0.5% Cu) copper ±uranium ±gold mineralisation (Hayward and Skirrow, 2010). The very large, sub-economic magnetite-rich alteration systems at the Acropolis, Wirrda Well and Murdie Murdie prospects are representatives of this assemblage, while relicts of it are observed in many other IOCG systems (e.g., Davidson et al., 2007).
Fluid inclusion data and oxygen isotope geothermometry for the magnetite-bearing assemblages in the Olympic Dam district indicate that fluid temperatures reached ~420 to 540°C (Oreskes and Einaudi, 1992; Bastrakov et al., 2007; Davidson et al., 2007). Based on Br/Cl ratios, and isotope and other data, Hayward and Skirrow (2010) concluded that sulphur in the generally minor sulphides associated with most magnetite-bearing alteration was arguably of magmatic-hydrothermal or leached-igneous rock origin (e.g., Bastrakov et al., 2007).
The three images that follow illustrate the geology, gravity and magnetic signature of the central Olympic IOCG Province and the location of the key IOCG deposits and Fe±Cu deposits and prospects of that region. For detail on each of these deposits/prospects, follow the links at the head of this record.
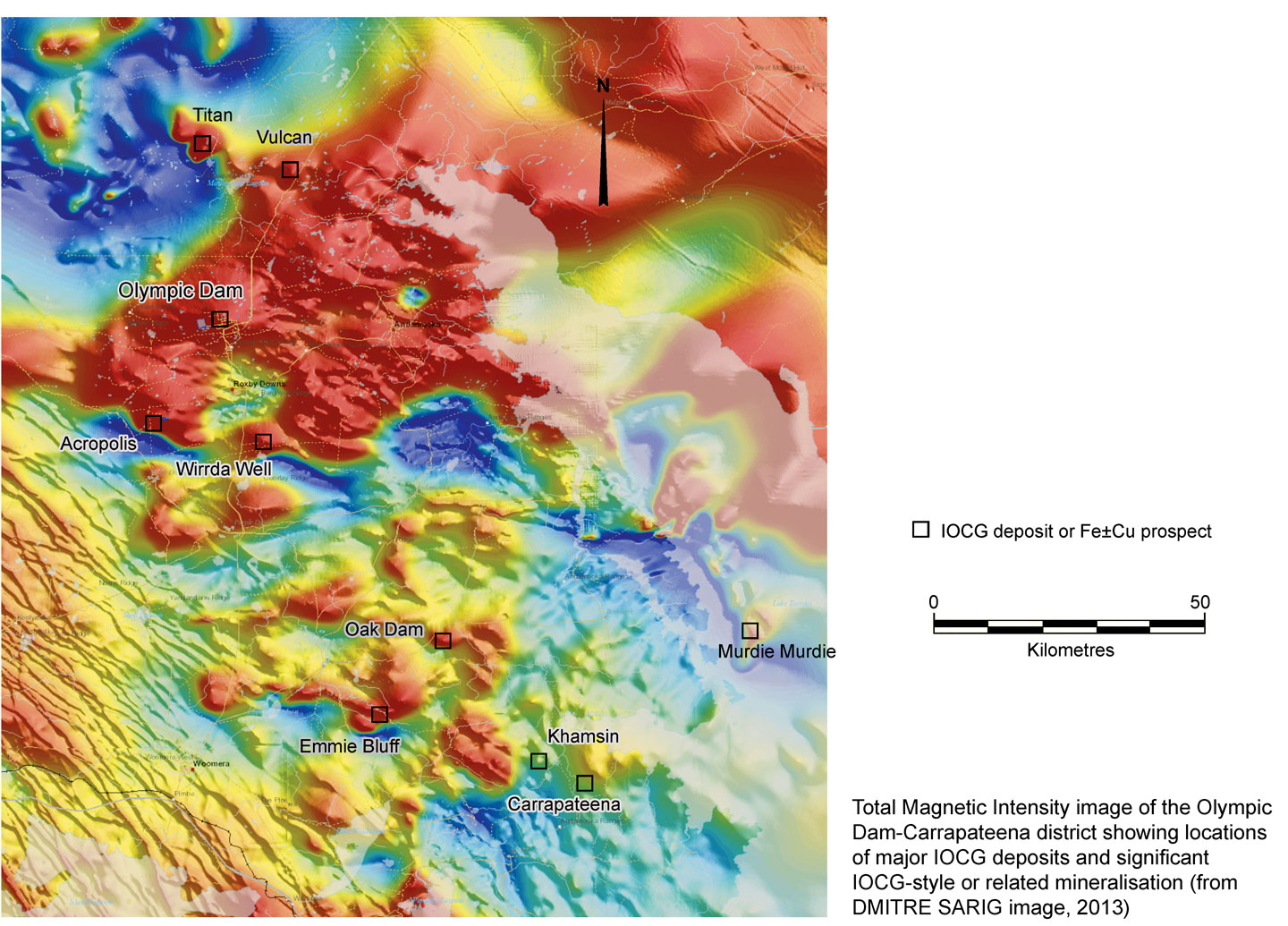
The most recent source geological information used to prepare this decription was dated: 2019.
Record last updated: 20/5/2021
This description is a summary from published sources, the chief of which are listed below. © Copyright Porter GeoConsultancy Pty Ltd. Unauthorised copying, reproduction, storage or dissemination prohibited.
|
|
Armit, R.J.,. Betts, P.G., Schaefer, B.F., Pankhurst, M.J. and Giles, D., 2104 - Provenance of the Early Mesoproterozoic Radium Creek Group in the northern Mount Painter Inlier: Correlating isotopic signatures to inform tectonic reconstructions: in Precambrian Research v.243, pp. 63-87.
|
Bastrakov E N, Skirrow R G and Davidson G J, 2007 - Fluid Evolution and Origins of Iron Oxide Cu-Au Prospects in the Olympic Dam District, Gawler Craton, South Australia: in Econ. Geol. v102 pp 1415-1440
|
Brotodewo, A., Tiddy, C., Zivak, D., Fabris, A. and Giles, D., 2021 - Geochemical discrimination of igneous zircon in the Gawler Craton, South Australia: in Australian J. of Earth Sciences v.68, pp. 557-579. doi.org/10.1080/08120099.2021.1825993
|
Budd A R and Skirrow R G, 2007 - The Nature and Origin of Gold Deposits of the Tarcoola Goldfield and Implications for the Central Gawler Gold Province, South Australia: in Econ. Geol. v102 pp 1541-1563
|
Chapman, N.D., Ferguson, M., Meffre, S.J., Stepanov, A., Maas, R. and Ehrig, K.J., 2019 - Pb-isotopic constraints on the source of A-type Suites: Insights from the Hiltaba Suite - Gawler Range Volcanics Magmatic Event, Gawler Craton, South Australia: in Lithos v.346-347, doi.org/10.1016/j.lithos.2019.105156.
|
Courtney-Davies, L., Ciobanu, C.L., Verdugo-Ihla, M.R., Cook, N.J., Ehrig, K., Wade, B.P., Zhu, Z.-Y. and Kamenetsky, V.S., 2020 - ~1760 Ma magnetite-bearing protoliths in the Olympic Dam deposit, South Australia: Implications for ore genesis and regional metallogeny: in Ore Geology Reviews v.118, doi.org/10.1016/j.oregeorev.2020.103337.
|
Curtis, S. and Thiel, S., 2019 - Identifying lithospheric boundaries using magnetotellurics and Nd isotope geochemistry: An example from the Gawler Craton, Australia: in Precambrian Research v.320, pp. 403-423.
|
Curtis, S., Wade, C. and Reid, A., 2018 - Sedimentary basin formation associated with a silicic large igneous province: stratigraphy and provenance of the Mesoproterozoic Roopena Basin, Gawler Range Volcanics: in Australian J. of Earth Sciences v.65, pp. 447-463.
|
Davidson G J, Paterson H, Meffre S and Berry R F, 2007 - Characteristics and Origin of the Oak Dam East Breccia-Hosted, Iron Oxide Cu-U-(Au) Deposit: Olympic Dam Region, Gawler Craton, South Australia: in Econ. Geol. v102 pp 1471-1498
|
Direen N G and Lyons P, 2007 - Regional Crustal Setting of Iron Oxide Cu-Au Mineral Systems of the Olympic Dam Region, South Australia: Insights from Potential-Field Modeling: in Econ. Geol. v102 pp 1397-1414
|
Ernst R.E., Liikane D.A., Jowitt S.M., Buchan K.L. and Blanchard, J.A., 2019 - A new plumbing system framework for mantle plume-related continental Large Igneous Provinces and their mafic-ultramafic intrusions: in Journal of Volcanology and Geothermal Research, v.384, pp. 75-84. doi.org/10.1016/j.jvolgeores.2019.07.007.
|
Fabris, A., 2022 - Geochemical characteristics of IOCG deposits from the Olympic Copper-Gold Province, South Australia: in Corriveau, L., Potter, E.G. and Mumin, A.H., (Eds.), 2022 Mineral systems with iron oxide coppergold (IOCG) and affiliated deposits Geological Association of Canada, Special Paper 52, pp. 247-262.
|
Forbes, C., Giles, D., Freeman, H., Sawyer, M. and Normington, V., 2015 - Glacial dispersion of hydrothermal monazite in the Prominent Hill deposit: An exploration tool: in J. of Geochemical Exploration v.156, pp. 10-33.
|
Fraser G L, Skirrow R G, Schmidt-Mumm A and Holm O, 2007 - Mesoproterozoic Gold in the Central Gawler Craton, South Australia: Geology, Alteration, Fluids, and Timing: in Econ. Geol. v102 pp 1511-1539
|
Hand M, Reid A and Jagodzinski L, 2007 - Tectonic Framework and Evolution of the Gawler Craton, Southern Australia: in Econ. Geol. v102 pp 1377-1395
|
Heinson, G., Didana, Y., Soeffky, P., Thiel, S. and Wise, T., 2018 - The crustal geophysical signature of a world-class magmatic mineral system: in Scientific Reports 2018:8, DOI:10.1038/s41598-018-29016-2.
|
Huang, Q., Kamenetsky, V.S., Ehrig, K., McPhie, J., Kamenetsky, M., Cross, K., Meffre, S., Agangi, A, Chambefort, I., Direen, N.G., Maas, R. and Apukhtina, O., 2016 - Olivine-phyric basalt in the Mesoproterozoic Gawler silicic large igneous province, South Australia: Examples at the Olympic Dam Iron Oxide Cu-U-Au-Ag deposit and other localities: in Precambrian Research v.281, pp. 185-199.
|
Huang, Q., Kamenetsky, V.S., McPhie, J., Ehrig, K., Meffre, S., Maas, R., Thompson, J., Kamenetsky, M., Chambefort, I., Apukhtina, O. and Hu, Y., 2015 - Neoproterozoic (ca. 820-830 Ma) mafic dykes at Olympic Dam, South Australia: Links with the Gairdner Large Igneous Province: in Precambrian Research v.271, pp. 160-172.
|
Jagodzinski, E.A., Crowley, J.L., Reid, A.J., Wade, C.E. and Bockmann, M.J., 2021 - High-precision CA-TIMS dating of the Hiltaba Suite, Gawler Craton: in Department for Energy and Mining, South Australia, Adelaide, Report Book 2021/00002, 63p.
|
Katona, L., 2020 - Geophysical signatures of IOCG prospects in the Olympic Cu-Au Province: in Mesa Journal v.92, pp. 26-37.
|
Kontonikas-Charos, A., Ciobanu, C.L., Cook, N.J., Ehrig, K., Krneta, S. and Kamenetsky, V.S., 2017 - Feldspar evolution in the Roxby Downs Granite, host to Fe-oxide Cu-Au-(U) mineralisation at Olympic Dam, South Australia: in Ore Geology Reviews v.80, pp. 838-859.
|
Morrissey, L., Barovich, K., Hand, M., Howard, K., Payne, J. and Reid, A., 2018 - The final event in the long evolution of the Gawler Craton: new constraints on 1450 Ma metamorphism and magmatism: in Mesa Journal, v.88/3, pp. 4-11.
|
Morrissey, L.J., Barovich, K.M., Hand, M., Howard, K.E, and Payne, J.L., 2019 - Magmatism and metamorphism at ca. 1.45 Ga in the northern Gawler Craton: The Australian record of rifting within Nuna (Columbia): in Geoscience Frontiers v.10, pp. 175-194. doi.org/10.1016/j.gsf.2018.07.006.
|
Payne, J.L., Hand, M., Barovich, K.M. and Wade, B.P., 2008 - Temporal constraints on the timing of high-grade metamorphism in the northern Gawler Craton: implications for assembly of the Australian Proterozoic: in Australian J. of Earth Sciences v.55, pp. 623-640.
|
Pollett, A., Thiel, S., Bendall, B., Raimondo, T. and Hand, M., 2019 - Mapping the Gawler Craton-Musgrave Province interface using integrated heat flow and magnetotellurics: in Tectonophysics v.756, pp. 43-56.
|
Porter T M, 2010 - Current Understanding of Iron Oxide Associated-Alkali Altered Mineralised Systems: Part II, A Review: in Porter T M, (Ed), 2010 Hydrothermal Iron Oxide Copper-Gold and Related Deposits: A Global Perspective, PGC Publishing, Adelaide v.3 pp. 33-106
|
Porter T M, 2010 - Current Understanding of Iron Oxide Associated-Alkali Altered Mineralised Systems: Part II, A Review: in Porter T M, (Ed), 2010 Hydrothermal Iron Oxide Copper-Gold and Related Deposits: A Global Perspective, PGC Publishing, Adelaide v.3 pp. 33-106
|
Reid, A. and Forster, M., 2021 - Mesoproterozoic thermal evolution of the northern Gawler Craton from 40Ar/39Ar geochronology: in Precambrian Research v.358, 16p. doi.org/10.1016/j.precamres.2021.106180.
|
Reid, A., 2019 - The Olympic Cu-Au Province, Gawler Craton: A Review of the Lithospheric Architecture, Geodynamic Setting, Alteration Systems, Cover Successions and Prospectivity: in Minerals (MDPI) v.9, issue 6, 37p.
|
Reid, A.J. and Fabris, A., 2015 - Influence of Preexisting Low Metamorphic Grade Sedimentary Successions on the Distribution of Iron Oxide Copper-Gold Mineralization in the Olympic Cu-Au Province, Gawler Craton: in Econ. Geol. v.110, pp. 2147-2157
|
Reid, A.J. and Payne, J.L., 2017 - Magmatic zircon Lu-Hf isotopic record of juvenile addition and crustal reworking in the Gawler Craton, Australia: in Lithos v.292-293, pp. 294-306.
|
Reid, A.J., Jagodzinski, E.A., Armit, R.J., Dutch, R.A., Kirkland, C.L., Betts, P.G. and Schaefer, B.F., 2014 - U-Pb and Hf isotopic evidence for Neoarchean and Paleoproterozoicbasement in the buried northern Gawler Craton, South Australia: in Precambrian Research v.250, pp. 127-142.
|
Rutherford, L., Hand, M. and Barovich, K., 2007 - Timing of Proterozoic metamorphism in the southern Curnamona Province: implications for tectonic models and continental reconstructions: in Australian J. of Earth Sciences v.54, pp. 65-81.
|
Selway, K., Ozaydın, S. and Payne, J., 2023 - Metasomatism and depletion of the southern Gawler Craton from combined mantle xenocryst and AusLAMP magnetotelluric data: in Exploration Geophysics, 20 Nov 2023, doi.org/10.1080/08123985.2023.2282711
|
Skirrow R G, Bastrakov E N, Barovich K, Fraser G L, Creaser R A, Fanning C M, Raymond O L and Davidson G J, 2007 - Timing of Iron Oxide Cu-Au-(U) Hydrothermal Activity and Nd Isotope Constraints on Metal Sources in the Gawler Craton, South Australia: in Econ. Geol. v102 pp 1441-1470
|
Skirrow, R.G., 2022 - Hematite-group IOCG ±U deposits: an update on their tectonic settings, hydrothermal characteristics, and Cu-Au-U mineralizing processes: in Corriveau, L., Potter, E.G. and Mumin, A.H., (Eds.), 2022 Mineral systems with iron oxide-copper-gold (IOCG) and affiliated deposits, Geological Association of Canada, Special Paper 52, pp. 27-51.
|
Skirrow, R.G., 2021 - Iron oxide copper-gold (IOCG) deposits - a review (part 1): settings, mineralogy, ore geochemistry, and classification within the Cu-Au-Fe (±Co, REE) deposit family: in Preprint accepted Nov 2021, for Ore Geology Reviews, 71p. doi.org/10.1016/j.oregeorev.2021.104569
|
Tiddy, C., Zivak, D., Hill, J., Giles, D., Hodgkison, J., Neumann, M. and Brotodewo, A., 2021 - Monazite as an Exploration Tool for Iron Oxide-Copper-Gold Mineralisation in the Gawler Craton, South Australia: in Minerals (MDPI) v.11, 30p. doi.org/10.3390/min11080809.
|
Tiddy, C.J., Betts, P.G., Neumann, M.R., Murphy, F.C., Stewart, J., Giles, D., Sawyer, M., Freeman, H. and Jourdan, F., 2020 - Interpretation of a ca. 1600-1580 Ma metamorphic core complex in the northern Gawler Craton, Australia: in Gondwana Research v.85, pp. 263-290.
|
Wade, C.E., McAvaney, S.O., Gordon, G.A. and Keeling, J.L., 2014 - Epithermal-style textures, brecciation, veining and alteration, southern Gawler Ranges margin, South Australia: in Department of State Development, South Australia, Adelaide, Report Book 2014/00009 123p.
|
Wade, C.E., Payne, J.L., Barovich, K.M. and Reid, A.J., 2019 - Heterogeneity of the sub-continental lithospheric mantle and non-juvenile mantle additions to a Proterozoic silicic large igneous province: in Lithos v.340-341, pp. 87-107.
|
Wade, C.E., Reid, A.J., Payne, J.L. and Barovich, K.M., 2019 - The link is in the geochemistry: how we know a metasomatised mantle underlies the mineral systems of the Gawler Craton: in Mesa Journal v.90, pp. 42-47.
|
Wade, C.E., Reid, A.J., Wingate, M.T.D., Jagodzinski, E.A. and Barovich, K., 2012 - Geochemistry and geochronology of the c. 1585 Ma Benagerie Volcanic Suite, southern Australia: Relationship to the Gawler Range Volcanics and implications for the petrogenesis of a Mesoproterozoic silicic large igneous province: in Ore Geology Reviews v.206-207, pp. 17-35.
|
Wise, T. and Thiel, S., 2020 - Proterozoic tectonothermal processes imaged with magnetotellurics and seismic reflection in southern Australia: in Geoscience Frontiers v.11, pp. 885-893. doi.org/10.1016/j.gsf.2019.09.006.
|
Porter GeoConsultancy Pty Ltd (PorterGeo) provides access to this database at no charge. It is largely based on scientific papers and reports in the public domain, and was current when the sources consulted were published. While PorterGeo endeavour to ensure the information was accurate at the time of compilation and subsequent updating, PorterGeo, its employees and servants: i). do not warrant, or make any representation regarding the use, or results of the use of the information contained herein as to its correctness, accuracy, currency, or otherwise; and ii). expressly disclaim all liability or responsibility to any person using the information or conclusions contained herein.
|
Top | Search Again | PGC Home | Terms & Conditions
|
|