Gangdese Belt - Xietongmen / Xiongcun, Newtongmen, Gaerqiong-Galale, Sharang, Jiru, Qulong / Julong, Jiama, Lunggar, Bangpu, Zhunuo, Lakange, Beimulang |
|
Xizang - Tibet, China |
Main commodities:
Cu Au Mo
|
|
 |
|
 |
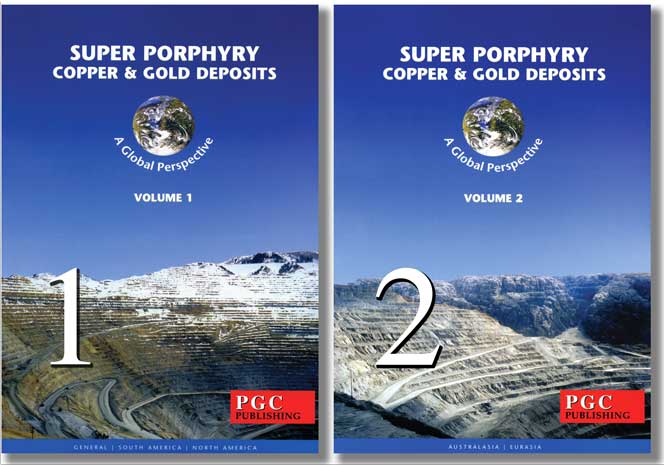 |
Super Porphyry Cu and Au

|
IOCG Deposits - 70 papers
|
All papers now Open Access.
Available as Full Text for direct download or on request. |
|
 |
The Gangdese Belt of porphyry and porphyry-skarn copper ± gold ± molybdenum ± iron deposits were formed during the Middle Jurassic, Early and Late Cretaceous, Paleocene to Eocene and Miocene within the east-west trending Gangdese Magmatic Arc of southern Tibet. These deposits, as described in detail individually later in this record, include (after Wang et al., 2018):
► Jurassic
• Xietongmen - 219.8 Mt @ 0.43% Cu, 0.51 g/t Au, 3.87 g/t Ag,
• Newtongmen - 388.9 Mt @ 0.32% Cu, 0.18 g/t Au and 0.87 g/t Ag.
These two deposits are ~260 km WSW of Lhasa and are ~3 km apart. They are related to 176 to 171 Ma, Jurassic quartz diorite and granodiorite porphyries. Other smaller Cu-Au prospects in the same district include Langtongmen, Olitongmen, Tangbai and Zemoduola.
► Cretaceous
Cretaceous skarn and porphyry style mineralisation hosted within Late Palaeozoic to Mesozoic carbonate rocks over the central Lahsa Terrane. Iron rich skarns are related to ~115 to 110 Ma granitic intrusions (e.g., Lunggar - 37 Mt @ 56% FeTotal), while skarn-porphyry Cu-Au systems are closely associated with ~90 to 80 Ma intrusions (e.g., Ga'erqiong-Galale, Balazha and Ri'a-Nixiong, ~1200, ~700 and ~650 km WNW of Lhasa respectively). The Ga'erqiong and Galale Cu-Au-Mo deposits apparently contain 24 and 40 t of Au respectively (Zhang et al., 2015).
► Eocene
• Sharang Mo - 10 Mt @ 0.061% Mo,
• Jiru Cu - 41.9 Mt @ 0.43 Cu.
These two small, low grade deposits are located in the eastern Gangdese belt (east of 88°E) and are dated at 52.25±0.31 and 49.2±1.7 Ma respectively in the Eocene. However, Jiru was apparently also overprinted by a pulse of Miocene mineralisation.
► Miocene
• Qulong / Julong Cu-Mo - 1420 Mt @ 0.5% Cu (Yang et al., 2009); 5.73 Gt @ 0.33% Cu, 0.02% Mo, 2.51 g/t Ag (Zijin Mining, 2023),
• Jiama Cu-Mo - 1054 Mt @ 0.44% Cu (Ying et al., 2014; Zheng et al., 2016).
These two deposits are dated at 16.4±0.5 and 14.7±0.3 Ma in the Miocene respectively. Other smaller, lower grade or less well investigated deposits include Tinggong, Lakang'e, Bangpu, Tangbula, Dabu, Zhunuo and Beimulang porphyry Cu-Mo. Zhunuo is the only deposit containing economic reserves of Au within the Miocene Gangdese Porphyry Belt (Zeng et al., 2017). Miocene skarn alteration related mineralisation is also known (e.g., the Zhibula Cu skarn near the Qulong deposit with 19.5 Mt ore @ 1.64% Cu; Xu et al., 2016).
The Gangdese Belt is part of the East Tethyan Orogenic Belt of central to southeast Asia, which, in turn, is the eastern segment of the greater Tethyan Orogenic Belt that extends from Indochina in the SE to the Atlas Mountains of Morocco to the west.
Other significant porphyry clusters within the East Tethyan Orogenic Belt include the Duolong Cluster and enclosing Bangong Co-Nujiang Mineral Belt, the Yulong Porphyry Belt, Yidun or Zhongdian Arc and the Ailaoshan-Red River Belt. The North Himalayan Mineral Belt lies to the south of the eastern half of the Gangdese Belt and includes hydrothermal Au, Au-Sb and Pb-Zn mineralisation within the Tethyan Himalaya Complex.
Regional Setting
The ~2000 km long by 150 to 300 km wide Gangdese Magmatic Arc lies within the generally east-west Lhasa Terrane of the Himalayan-Tibetan Orogen. This orogen is composed (from south to north) of the Tethyan Himalaya (Gondwana/Indian affinity), Lhasa (Gondwana affinity basement), Qiangtang (divided into the Gondwanan South Qiangtang and North Qiangtang of mainly Tibetan/Asian affinity) terranes, and the Songpan-Garze Terrane. The latter comprises a thick sequence of Upper Triassic marine turbidites, dislocated by numerous thrusts, interpreted to be floored by oceanic crust. These terranes are separated from each other by the Indus-Yarlung Tsangpo, Bangong-Nujiang, and Jinsha River sutures, respectively (Yin and Harrison, 2000; Zhu et al., 2013). The Lhasa terrane is divided into the Northern, Central and Southern Lhasa sub-terranes, bounded by the Shiquan River-Nam Tso Mélange zone and the Luobadui-Milashan fault, respectively (Zhu et al., 2011, 2013). The Central Lhasa Sub-terrane broadly corresponds to a core of amphibolite to granulite facies crystalline basement, the Nyingchi Complex, which is mostly younger than 490 or 340 Ma (detrital zircon; Dong et al., 2010; Guo et al., 2012; Zhang et al., 2008), overlain by Palaeozoic to Cretaceous sedimentary cover (Hu et al., 2005; Zhu et al., 2011). This older core is regarded to have been a sliver rifted from the margin of Gondwana during the Late Triassic, and transported north ahead of the approaching Indian plate which had similarly been detached from Gondwana (Zhu et al., 2013; Li et al., 2016).
The Lhasa Terrane appears to have collided with the Qiangtang Terrane on its northern margin in the Early Cretaceous (Kapp et al., 2005; Zhu et al., 2016). The Northern Lhasa Sub-terrane is largely occupied by Jurassic to Cretaceous arc volcanic rocks, intruded by 130 to 80 Ma plutonic rocks (Zhu et al., 2009), assumed to be the result of southward subduction of the intervening Bangong-Nujiang oceanic crust prior to this collision (Yang et al., 2015). These Mesozoic intrusives included a wide range of lithologies, comprising gabbro, diorite, tonalite, granodiorite, monzogranite and granite. All of the intermediate to silicic intrusions are characterised by an adakitic affinity (Zheng et al., 2014). Cao et al. (2018) detail this collision as follows:
i). 145 to 120 Ma - continuation of southward subduction of the Bangong-Nujiang oceanic plate below the northern margin of the Lhasa terrane;
ii). 120 to 115 Ma - Lhasa-Qiangtang continental collision, accompanied by thrusting and imbrication and formation of S-type granitoids;
iii). 115 to 110 Ma - break-off of the Bangong-Nujiang Ocean slab followed by extension, asthenospheric upwelling and generation of bimodal magmatism;
iv). 110 to 95 Ma - reversal of the stress field, resulting in compression induced crustal thickening of the Central Lhasa lower crust;
v). 95 to 80 Ma - delamination of lithospheric mantle below the central Lhasa Terrane, followed by asthenospheric upwelling, lower crustal melting to form bimodal adakitic magmas that were Mg-rich and Sr-Nd-Hf depleted.
Skarn garnet, epidote, diopside magnetite iron deposits were formed, distributed over an interval of ~1200 km of the central Lhasa Terrane, hosted by Permian carbonate rocks and related to 113 Ma granitoids, while porphyry-skarn Cu-Au-Mo deposits accompanied the ~90 Ma granites.
To the south, northward subduction of the Neo-Tethyan oceanic plate, separating the Indian plate and Lhasa Terrane, began in the Late Triassic or Early Jurassic (Chu et al., 2006) and also produced voluminous Jurassic-Cretaceous calc-alkaline magmatism within the Lhasa Terrane simultaneously with the closure of the Bangong-Nujiang ocean to the north. Significant porphyry copper mineralisation is developed over a >200 km interval west of Lhasa related to this magmatism in the Southern Lhasa Sub-terrane associated with Early to Middle Jurassic 182 to 166 Ma intrusions (Tafti et al., 2014; Zou et al., 2017). These intrusions are characterised by high zircon Ce4+/Ce3+ ratios and Eu anomalism, with evidence they were oxidised, water-saturated and exsolved volatile phases at an early stage (Zou et al., 2017).
Whole-rock Nd and zircon Hf isotopic compositions of the granitoid rocks in the Lhasa Terrane suggest an old and isotopically evolved central Lhasa subterrane with juvenile northern and southern subterranes reflecting the two subduction regimes (Zhu et al., 2011; Hou et al., 2015). These subdivisions also broadly correspond to the Northern, Central, and Southern Lhasa subterranes.
A magmatic gap, or quiescent period, occurred in Gangdese arc magmatism in the southern Lhasa terrane between ~80 and 70 Ma (Wen et al., 2008; Ji et al., 2009).
The time of India-Asia continental collision is variously interpreted, as 65 to 55 Ma (Beck et al., 1995; DeCelles et al., 1998; Searle et al., 1999; Yin and Harrison, 2000; Ding et al., 2005); 50 to 45 Ma (Dewey et al., 1989; Le Pichon et al., 1992); and 35 Ma (Aitchison et al., 2007). Wu et al. (2008) suggested 55 Ma as the peak time of the main-stage continental collision, when large volumes of volcanic and plutonic magmatism was emplaced in the southern Tibetan plateau.
Alternatively it has been suggested that roll-back of the Neo-Tethyan slab probably occurred at ~69 to 53 Ma (Zhou et al., 2018), as suggested by the development of an extensional setting in the Qiangtang Terrane during this period, characterised by east-west trending sedimentary basins (Chung et al., 2005). Intrusions of this age range have gabbroic, monzonitic, quartz monzonitic and granodioritic compositions, and plot in the high-K calc-alkaline field. They have slightly higher magmatic temperatures of 770 to 670°C, and Ti in zircon temperatures of up to 860 to 580°C, and slightly lower zircon Hf isotopic ratios compared to Jurassic to Cretaceous magmas (Wang et al., 2017).
This roll-back was followed by slab break-off at ~53 to 50 Ma which corresponded to the most voluminous magmatism in the Gangdese belt (Kapp et al., 2005; Wen, 2007; Wang et al., 2015; Zhu et al., 2015; Zhou et al., 2018), represented by Paleocene to Eocene I-type intrusive rocks and the widespread Linzizong volcanic successions (Mo et al., 2008; Zhu et al., 2015, 2017). These rocks all fall within calc-alkaline and high-K calc-alkaline fields and include gabbroic-diorite, diorite, monzonite, quartz monzonite, granodiorite and granitic compositions. Abnormally high zircon saturation temperature estimates of up to 800°C and Ti in zircon temperatures of up to 980°C suggest a period of thermal anomalism during this interval (Zhou et al., 2018). Starting from ~53 Ma, magmas have increased K2O contents, whilst their zircons have decreased Th/U ratios, and Y and Yb contents. These zircons have heterogeneous Hf isotopic compositions (εHf(t)=-5.3 to 15.1). All have weak to moderate enrichments of LREE relative to MREE and HREE, and most have weakly negative Eu anomalies. Zhou et al. (2018) and Wang et al. (2018) suggest the isotopic compositions and geochemical characteristics of this magmatism provides evidence supporting slab break-off and subsequent ingress of asthenosphere mantle, resulting in extensive crustal melting, bimodal magmatism, magma mixing and crustal growth through magma underplating.
The Indian-Tibet/Asian collision was ongoing between ~49 to 43 Ma, but only produced minor magmas. These plot in calc-alkaline, high-K calc-alkaline and shoshonitic fields with high Th/Y and La/Yb ratios and K2O contents, but significantly lower Zr saturation and magmatic crystallisation temperatures, mostly <750°C.
Magmatic rocks emplaced between 65 and 41 Ma have relatively juvenile isotopic compositions, interpreted to suggest the absence of ancient Tibetan SCLM beneath the Gangdese arc throughout this interval (Wang et al., 2018). A third stage of porphyry copper mineralisation is associated with this magmatism at ~50 Ma during the late stages of slab break-off. Magmas during this period are relatively water-poor (c.f., low La/Yb and Sr/Y ratios, negative Eu anomalies and high temperatures) and not well oxidised, and hence less fertilised> This is consistent with the generally limited size and number of porphyry Cu deposits formed during this interval (Zhou et al., 2018).
A further magmatic gap or quiescent period extended from ~40 to ~30 Ma. This would be consistent with flat subduction of the Indian plate throughout the Oligocene, suggesting the Indian continent was subducted/thrust below southern Tibet at a shallow angle with little or no intervening asthenospheric mantle wedge (Guillot et al., 2008; Ding et al., 2016). This gap was followed between ~30 and 13 Ma by the emplacement of at least 5 Oligo-Miocene igneous suites in southern Tibet, comprising small volume potassic to ultrapotassic (trachytic) volcanic rocks and small adakite-like calcalkaline to alkaline intrusions. This magmatism, which decreases from west to east, is interpreted to be post-collisional. More than 50 post-collisional adakite-like intrusions are recognised in a narrow 1500 km long corridor along the southern margin of the Lhasa terrane. They vary from strongly porphyritic to more equigranular and occur as stocks or dykes ranging from 10 km2 to <100 m2 in area with ages ranging from 40 and 13 Ma, peaking at 16±2 Ma (Hou et al., 2009). Only those located in the eastern part of the southern Lhasa Terrane (the 700 km long Gangdese porphyry Cu belt) are associated with known Cu-Mo mineralisation (Hou et al., 2009). In contrast, known deposits in the western Gangdese are mainly Pb-Zn polymetallic, e.g., the Narusongduo breccia Pb-Zn (Ji et al., 2012); Sinongduo epithermal Pb-Zn-Ag-Au (Tang et al., 2016); Chagele skarn-hydrothermal Pb-Zn-Mo (Wang et al., 2012); Chaizi hydrothermal vein Pb-Zn; Nuocang skarn Pb-Zn polymetallic (Jiang et al.., 2018).
Under the interpretation described above, the collision of the India and Tibet/Asia plates started at ~50 Ma when the Greater Indian plate (i.e., Indian continental margin; Ali and Aitchison, 2005) first encountered the edge of the Lhasa terrane (Meng et al., 2012; van Hinsbergen et al., 2012; Zhu et al., 2015; Ding et al., 2016) which was by then accreted to Tibet/Asia. For the next 16 m.y., the Indian continental margin continued to subduct beneath the Tibet/Asia plate, accommodated by shortening of the latter, and accompanied by early uplift of the Tibetan Plateau (soft collision; Ali and Aitchison, 2005). At ~30 Ma, the thicker and more rigid Indian Craton encountered the edge of the Lhasa Terrane (hard collision) and the character of subduction changed to the mode that has continued to the present.
Seismic tomographic observations indicate the Indian continental lithosphere currently extends northward below the Tibetan plateau, where it is in direct contact with the base of the Tibetan crust. The Tibet/Asia plate subcrustal lithospheric mantle (SCLM) appears to be absent in the south below the Lhasa Terrane, particularly in the west, and is assumed to have delaminated and been detached ahead of the hard collision (Chung et al., 2009; Nábělek et al., 2009) immediately following or during the Neo-Tethyan slab break-off in the Eocene. To the south of the Lhasa Terrane, the Indian Plate is 100 to 200 km thick, whilst in northern to central Tibet, the Tibet/Asian plate is ~180 to 200 km thick. Seismic data indicate that the crust in southern Tibet, below the Lhasa Terrane, is ~75 km thick, comprising ~50 km of Tibetan crust, underthrust by ~25 km of Indian continental crust, underlain by Indian SCLM (Owens and Zandt, 1997; Nábělek et al., 2009). These thickness changes could be explained by detachment of the SCLM from below the Tibet/Asian plate, while Neoproterozoic to Miocene sedimentary cover of the Indian Plate is 'scraped off' and imbricated to form a thick accretionary prism that is the Tethyan Himalaya terrane (e.g., Nábělek et al., 2009; Capitanio et al., 2010). Only a thinner (~25 km thick), remnant basal section of the Indian Plate is then subducted below the Lhasa Terrane. The degree of underthrusting of the Indian plate, which is NE vergent, decreases from west to east, while the dip angle of thrusting increases in the same direction. The main Miocene Gangdese porphyry deposits are in the east where an thin asthenospheric wedge may exist between the base of Tibet/Asia continental crust and the top of Indian continental crust.
Wang et al. (2018) propose a model that involves Tibet/Asia continental crust directly overlying flat dipping Indian continental crust initially, but beginning to steepen from east to west with time, allowing a thin intervening asthenospheric wedge to develop. In this model, an underplate of residual fertilised, oxidised, lithosphere from Eocene and earlier subduction forms the base of the Tibet/Asia crust. The subducted/underthrust Indian continental plate included carbonate, evaporitic and other sedimentary rocks that are common in north India. These were subjected to dehydration, which further hydrated, oxidised and added sulphur to the overlying underplated base of the Tibet/Asia crust. To the east, upon opening of the asthenospheric mantle wedge during the Miocene, mantle upwelled and raised the thermal gradient allowing hydrated and oxidized Tibetan/Asian lower crust to begin to melt at an ambient temperature of ~800°C and depth of 45 to 50 km. Deeper, at ~70 km in the Indian crust and SCLM, trachytic magmatism commenced due to phlogopite breakdown at ~1100°C,
near the garnet-spinel transition, producing low volume, K-rich, trachytic partial melts (e.g., Condamine et al., 2016).
The affect of these trachytic melts varied across the Gangdese Magmatic Belt. In the west, they rose into cool, relatively rigid crust, with no extensive associated crustal melting, and consequently are the dominant Miocene magmatic rocks occurring as both small intrusions and extensive trachytic volcanic rocks. In contrast, in the east, the upwelling mantle within the asthospheric wedge resulted in the 1100°C isotherm extending up to encroach upon the upper Indian SCLM and continental crust, as well as the lower Tibet/Asia continental crust and fertilised underplate. This resulted in large scale melting, including the hydrous fertilised underplate at the base of the Tibet/Asia plate, to produce the extensive high Sr/Y magmas of the Gangdese Batholith and the Linzizong volcanic succession. These lower crustal melt sheets, which were fertile for porphyry Cu deposit formation, trapped and absorbed the alkaline trachytic rising from below, inhibiting their further rise to the surface. This contrast with the western section of the belt where only small volumes of high-Sr/Y granitoids were generated, with only Zhunuo Cu-Mo deposit and a few intrusions with mineralization, but where large volumes of trachytic volcanic rocks were erupted.
The information in this section of the summary is drawn from Zheng et al., 2014, Yang et al., 2015, Wang et al., 2018 and Zhou et al., 2018.
JURASSIC DEPOSITS
Xietongmen Cu-Au
The Xietongmen deposit lies within the Xietongmen district, which is ~260 km to the west to WSW of Lhasa in the Tibet Autonomous Region of China, at altitudes of from 3900 to 5700 masl. It lies a few kilometres north of the Indus-Tsangpo suture which juxtaposes rocks of Asian and Neo-Tethyan/Indian affinity to the north and south, respectively.
Porphyry mineralisation at Xietongmen formed during Middle Jurassic volcanic arc activity in the Lhasa Terrane, prior to its accretion to the southern margin of Tibet/Asia.
District geology. The district is underlain by rocks that range from an i). Early Jurassic or older flysch sequence of clastic, carbonate and volcaniclastic rocks which are exposed in the southern part of the district; ii). Early Jurassic (~188 to 177 Ma) mafic flows, tuffs and fragmental units and coeval intrusive rock, with lesser felsic tuffs, as well as fine-grained clastic sedimentary rocks with minor carbonate or calcareous horizons in the central part of the district. These sequences are crosscut by iii). Early to Middle Jurassic (182 to 171 Ma) hornblende quartz diorite porphyry to hornblende diorite porphyry dykes and stocks, including intrusions related to porphyry Cu-Au±Mo mineralisation, and occur in a NW-trending belt across the centre of the district. The Jurassic igneous assemblage was intruded by iv). mafic dykes between the Late Jurassic and the Cretaceous, including diorite and variably porphyritic diorite to andesite, then by v). an Eocene (50 to 47 Ma) biotite granodiorite batholith and related dykes on the eastern side of the district, and finally by volumetrically minor vi). biotite lamprophyre dykes that are mostly <1 m thick (Tafti et al., 2014).
The structure of the district is dominated by four east-west striking, and moderately north dipping, sinistral-oblique thrust faults, located in the southern part of the district. The suturing and crosscutting relationships between thrust structures and intrusions dated to between 180 and 174 Ma, constrain the main stage of thrust fault activity in the district to the Middle Jurassic. Two of these faults, the Contact and Adit-1 thrusts, truncate the Xietongmen deposit and form the footwall and hanging wall to mineralisation, respectively. Numerous zones of cataclasis deform the Xietongmen deposit between these bounding thrust surfaces (Tafti et al., 2014).
A NW-trending (290 to 310°) corridor of alteration that is >4.5 km long and up to 1.5 km wide, encloses and extends well beyond the limits of known mineral occurrences in the district. It is obscured by Quaternary cover to the SE and is likely truncated by the Eocene batholith to the east. This broader alteration halo includes K silicate, sodic-calcic, quartz-sericite-pyrite and propylitic assemblages of variable intensity. Known Cu deposits and significant Cu-Au anomalism is associated with the K silicate assemblage, defined by the presence of K feldspar, and with Na-Ca alteration, defined by the presence of albite, actinolite and epidote. Strong to intense quartz-sericite-pyrite alteration is best developed west and NW of the Xietongmen deposit
and north and NE of the Newtongmen deposit. These zones are enclosed by a more extensive development of propylitic alteration, characterised by chlorite, epidote and calcite (Tafti et al., 2014).
Deposit Geology. At a cutoff grade of 0.15% copper, the Xietongmen deposit has plan dimensions of 1300 x 600 m, with an average true vertical thickness of ~250 m. It forms a north dipping, east plunging tabular body, trending WNW at 280 to 300°. The hanging wall and footwall are defined by the post-mineral Adit-1 and Contact brittle-ductile thrust faults respectively, both of which truncate mineralisation. These faults strike at 265 to 280° and dip at ~40°N. The deposit is truncated to the east and NE by the Eocene granodiorite , whilst the less distinct western boundary is gradational, declining laterally from above average grade to below the 0.15% Cu cut-off over an interval of <100 m.
Host rocks to the Xietongmen deposit are intensely altered, commonly obscuring the protolith texture and composition. However, there is sufficient evidence to indicate the host rocks to mainly be hornblende diorite porphyry with an age of 183 to 177 Ma (U-Pb zircon; Tafti et al., 2014). The deposit is centred on a distinct Middle Jurassic quartz diorite porphyry dated as 171.7±1.2 and 171.3±1.0 Ma (Tafti et al., 2009) that was intruded into the more extensive hornblende diorite porphyry intrusions and mafic volcanic and volcaniclastic tuff units. This intrusion is a generally continuous, body that is <100 to 200 m in diameter in the centre of the deposit and closely correlates with the distribution and grade of Cu-Au mineralisation, the density of quartz-sulphide veining and alteration zoning patterns.
Alteration, veining and mineralisation are zoned around the same central quartz diorite porphyry intrusion. The highest grade mineralisation is associated with a core of early K silicate alteration and related veining that occurs within and proximal to that intrusion, with weaker grades in a transitional shell of pervasive biotite-sericite that passes outward into poorly mineralised quartz-sericite-pyrite±pyrrhotite alteration. A similar trend is observed from the deeper northern half to shallower southern sections of the deposit.
• The K silicate alteration is texturally destructive and is characterised by an assemblage of brown biotite, magnetite, K feldspar, pyrite, chalcopyrite, minor andalusite, and anhydrite, with variable, but mostly low to absent pyrrhotite, and trace molybdenite. Biotite and magnetite are best developed adjacent to the central quartz diorite porphyry intrusion contact, with magnetite and biotite decreasing outward as K feldspar and quartz become more abundant. K silicate alteration has a higher chalcopyrite:pyrite ratio and a lower overall sulphide concentration, generally <5%. Quartz-sulphide, biotite-sulphide, magnetite-sulphide, and chalcopyrite-dominated veinlets, are closely associated with early K silicate alteration.
• Quartz-sericite-pyrite±pyrrhotite alteration, which is commonly texturally destructive, defines a broad envelope around the deposit. It generally only contains geochemically anomalous levels of Cu but often carries 100 to 300 ppb Au, with narrow intervals where there are high densities of pyrite-dominated veinlets locally with >1 g/t Au. Sericite partially replaces early K silicate biotite, K feldspar and andalusite that forms a biotite-sericite transition zone. Pyrrhotite is a major component of this alteration, and is commonly >5% in the south part of the deposit just above the Contact thrust fault. Where pyrite and pyrrhotite occur together, they are intergrown and were likely coprecipitated. This alteration type is at least partly coeval with the K silicate stage, and any overlap may reflect telescoping during thermal collapse.
• Quartz-sericite-pyrite alteration is the only alteration style that is known in the hanging wall of the Adit-1 fault (and the orebody). It extends well to the west into and beyond the Langtongmen prospect, but is truncated to the east by the Eocene granodiorite batholith. It is overprinted by later hornfelsing.
• Biotite-sericite transition zone, which is not regarded as a separate alteration style, but a transition from the K silicate to quartz-sericite-pyrite±pyrrhotite zones, with relicts of the former evident. However, it contains copper-gold mineralisation of moderate grade and locally has a higher concentration of pyrite, accompanied by minor pyrrhotite, than early K silicate alteration
• Propylitic alteration is rare in the immediate vicinity of the deposit, and is only found as a localised, much younger, fault-controlled overprint. It comprises an assemblage of chlorite, epidote, sericite or illite, and carbonate, minor albite, relicts of hematized igneous magnetite, and typically <2% disseminated pyrite.
• Sodic-calcic alteration affects the hornblende diorite intrusion below the footwall Contact thrust fault and, more weakly, the hornblende diorite
intrusion located west of the deposit. It succeeds an early brown biotite and comprises various combinations of albite, biotite, actinolite, quartz, dravite tourmaline, epidote and sericite, with a low sulphide concentration of minor pyrite and trace chalcopyrite. It has been structurally juxtaposed on the deposit, but is not found within it.
Veining. A number of vein types are recognised within the deposit, namely:
• Early quartz-sulphide veinlets which occur throughout the deposit, but are best developed on the margins of the quartz diorite
porphyry intrusion where they may coalesce to form >70% of the rock volume. Elsewhere in the deposit, these veinlets is typically between 5 and 10% and are the most closely associated with high-grade Cu-Au mineralisation. Veins are mostly <1 cm wide and are typically sinuous, discontinuous (A-type), and are strongly folded and/or brecciated. The major vein mineral is grey quartz with intergranular pyrite and chalcopyrite.
• Biotite-sulphide and magnetite-sulphide veinlets which are paragenetically equivalent. Biotite-sulphide veinlets are concentrated to the south, whereas magnetite-sulphide are mostly in the north. Both cut early quartz-sulphide veinlets and have a vein density mostly of <1 to 2%. Both contain quartz,
biotite, magnetite, pyrite, chalcopyrite, minor sericite and rare sphalerite. Both form stockworks of planar, narrow (<3 mm) veinlets as well as wider alteration 'bands' and irregular replacements. Narrow selvages of biotite-sulphide ±magnetite are common.
• Chalcopyrite-rich sulphide veinlets which are sinuous, mostly <1 cm in width, and are defined by a total sulphide concentration that is >75%. They contain chalcopyrite, commonly accompanied by pyrite and/or less common pyrrhotite, along with minor quartz and trace calcite. Sulphide-rich
veins evolve from early, sinuous chalcopyrite-pyrite ±pyrrhotite veins to progressively more planar veinlets increasingly dominated by pyrite. They are most common in zones of high-density quartz-sulphide veinlets at the margin of the quartz diorite porphyry intrusion.
• Late pyrite veinlets are found throughout the deposit, but are most abundant within peripheral quartz-sericite-pyrite alteration. Minor accessory minerals include quartz, biotite, chlorite and rare magnetite. These veins typically lack chalcopyrite and are mostly <2 cm thick, but range up
to tens of cms. At the periphery of the deposit, these veins have prominent alteration envelopes of quartz, sericite and pyrite, locally accompanied by pyrrhotite.
• Late polymetallic veins found throughout the deposit, but relatively more densely distributed in the centre of the deposit and in the hanging wall to the deposit above the Adit-1 fault. They vary from narrow fracture infills to >1 m thick veins and can be planar, sinuous or sheared. Principal minerals are sphalerite and pyrite accompanied by minor quartz, galena, and chalcopyrite and highly variable concentrations of pyrrhotite and carbonate.
Distribution of Mineralisation. Oxide, supergene and hypogene mineralisation occurs in four distinct zones, overlain by a thin, barren alluvial layer sourced from the unmineralised hornblende diorite intrusion west of the deposit. These include: i). strongly oxidised upper zone of variably transported colluvial debris of altered and mineralised material derived from the immediately underlying deposit. Copper has been leached, but gold is retained; ii). a variably developed supergene sulphide zone in which chalcopyrite and, to a lesser extent, pyrite and pyrrhotite have been replaced to form chalcocite and minor covellite; iii). a transitional zone containing hypogene chalcopyrite incipiently to partially replaced by supergene chalcocite and trace covellite; and iv). a hypogene zone. Supergene development is strongest to the south, whilst only hypogene mineralisation is present in the north below the Adit-1 thrust fault. The oxide (leached cap) and supergene sulphide zones form <1 and about 9% of the total mineral resource, respectively.
The hypogene sulphide content of the deposit ranges from ~3% to >15%, averaging ~8.5%. Pyrite is the most abundant sulphide, while pyrrhotite is commonly <1% but is locally >5%;, being most abundant and regularly distributed in lower grade intervals just above the footwall Contact thrust fault in the south part of the deposit but is only locally and erratically present in higher grade zones. Chalcopyrite is the only identified hypogene copper mineral, mostly occurring as disseminations and in veinlets mostly accompanying K silicate and biotite-sericite alteration. Molybdenite is a minor, erratically distributed phase. Sphalerite occurs in trace amounts in most types of veins but mostly in late polymetallic veins which also contain the bulk of the minor galena and arsenopyrite. A few ~5 µm gold and electrum grains have been identified, occurring as inclusions in pyrite. Grains of silver-telluride and bismuth-silver telluride have been recognised in association with the electrum.
Newtongmen Cu-Au-Mo
The Newtongmen porphyry Cu-Au±Mo deposit is ~3 km NW of Xietongmen. It was discovered by surface prospecting and rock chip geochemical sampling by Continental Minerals in 2005, with follow-up drilling in 2006 (Lang et al., 2007). It is the most northwesterly of the known deposits within the district, although anomalous zones of Cu-Au±Mo mineralisation, continue northwestward within the alteration corridor (Tafti et al., 2014).
Geology. Rock types within, and immediately surrounding the deposit, include a bedded mafic tuff that was sequentially intruded by irregular to dyke-like bodies of hornblende diorite porphyry and quartz diorite porphyry, all of which have Early to Middle Jurassic ages (U-Pb; zircon). The mafic tuff and fragmental volcaniclastic interbeds are predominantly found on the east to NE side of the deposit and only host minor amounts of the Cu-Au mineralisation. The most abundant rock type in the deposit is the hornblende diorite porphyry which hosts the bulk of the Cu-Au mineralisation, and is texturally and mineralogically very similar to the major host rock at the Xietongmen deposit. Numerous, but volumetrically minor quartz diorite porphyry intrusions cut the mafic tuff and the hornblende diorite porphyry. This lithology is widely distributed throughout the deposit and has a close spatial association with Cu-Au±Mo. The deposit is truncated on its southern and southwestern boundaries by a thick, NW trending intrusion of quartz diorite porphyry. This intrusion is characterised by up to 20% round to subhedral and commonly resorbed, quartz phenocrysts which are mostly between 10 and 15 mm in size, as well as a coarser grained matrix and a more felsic composition. It only has minor, low-grade Cu-Au±Mo mineralisation hosted by sodic-calcic alteration which has overprinted similar but higher grade mineralisation related to early K silicate alteration. Jurassic rocks are cut by minor andesite, diorite and rare lamprophyre dykes that are mostly <1 to 2 m thick (Tafti et al., 2014).
Structure. The deposit is cut by two main faults, i). a NE trending sinistral structure to the NW of the deposit; and ii). a major NNW aligned fault on the eastern side of the deposit, with only apparent minor offset. Brittle-ductile structures are present but rare and are typically <1 m in width (Tafti et al., 2014).
Alteration. Cu-Au±Mo mineralisation is associated with pervasive alteration and occurs in veins that were formed at 174.2±1.1 Ma in the Middle Jurassic (Re-Os; molybdenite; Tafti et al., 2009). The strongest mineralisation occurs in zones of early K silicate alteration, characterised by an assemblage of biotite, magnetite, quartz, K feldspar, pyrite, chalcopyrite and molybdenite as as i). sulphide disseminations and ii). in veins that include quartz-sulphide veins and veinlets carrying quartz, pyrite, chalcopyrite, molybdenite, magnetite and biotite, and in biotite-magnetite-sulphide veins and veinlets. Molybdenite±quartz±pyrite veins and veinlets are locally present, with crosscutting relationships suggesting they are a slightly younger variant of early quartz-sulphide veinlets (Tafti et al., 2014).
• Sodic-calcic alteration overprints the K silicate assemblage, mostly to the south and west adjacent to the quartz diorite porphyry intrusion. This alteration comprises various combinations of albite, epidote, actinolite, magnetite, chlorite, minor tourmaline and quartz, and occurs as both pervasive alteration and in associated, mineralogically similar veins and their selvages. Where intensely developed, sodic-calcic alteration does not contain strong Cu or Au mineralisation. It forms a partial overprint on older, well mineralized K silicate alteration, and in those cases significant Cu-Au±Mo mineralisation is retained, or redistributed into chlorite-chalcopyrite veins that also contain minor quartz, magnetite and biotite (Tafti et al., 2014).
• Quartz-sericite-pyrite alteration and associated pyrite-dominated veins are poorly mineralised, and mainly occur on the NE and eastern margins of the deposit where they overprint early, well-mineralized K silicate alteration and dilute grade (Tafti et al., 2014).
• Propylitic alteration comprises an assemblage of quartz, chlorite, calcite, epidote, hematite, sericite and pyrite, predominantly surrounding the deposit, but also occurring irregularly distributed through the mineralised zone.
In contrast to Xietongmen, Newtongmen has not been significantly recrystallised. It is located at the outer, western margin of the hornfels aureole which surrounds the Eocene granodiorite batholith and thermal recrystallisation is weak to absent. Similarly, mechanical recrystallisation related to brittle-ductile deformation is also rare, weak and localised.
Mineralisation. Hypogene mineralisation has a total sulphide content of between 3 and 5%, with a pyrite:chalcopyrite ratio that is commonly close to 1:1. Sulphide minerals, in order of decreasing relative abundance, comprise pyrite, chalcopyrite and molybdenite, with trace sphalerite, galena and pyrrhotite. No gold grains have not been observed in core or in thin section, but gold and copper exhibit an close correlation with an Au:Cu ratio of 0.77:1. The deposit has very low concentrations of potentially deleterious trace elements (Tafti et al., 2014).
Hypogene sulphides dominate, with chalcopyrite being the only primary copper mineral identified. Molybdenite is found throughout the deposit with wide zones of >100 ppm Mo. Supergene oxidation is weak across the deposit with hypogene chalcopyrite and pyrite in outcrop. Weak supergene effects extend to tens of metres depth but only produced partial replacement of hypogene chalcopyrite and pyrite with chalcocite and minor covellite. Minor chrysocolla, malachite and/or azurite are locally observed in open fractures within a few tens of metres of the surface (Tafti et al., 2014).
Xietongmen District Jurassic Deposits
Approximately 1.3 km west of the Xietongmen deposit there is a strongly deformed development of mineralisation that comprises the Langtongmen Cu-Au prospect. It occurs in the immediate hanging wall of the Adit-1 thrust fault that forms the hanging wall at Xietongmen. The Langtongmen prospect has characteristics that are identical to those found in the deeper parts of the Xietongmen deposit (Tafti et al., 2014).
The Newtongmen deposit and the Olitongmen Cu-Au prospect, which are not strongly deformed, occur to the north, in the hanging wall to the SBF thrust fault (500 to 600 m north of, and parallel to the Adit-1 fault). The characteristics of the Newtongmen deposit are generally similar to those in the Xietongmen deposit but Newtongmen contains only minor supergene mineralisation, is cut by very few late polymetallic veinlets, and contains zones of strong, weakly mineralized sodic alteration related to a relatively later stage of quartz diorite porphyry intrusion. The Olitongmen prospect has characteristics similar to the Newtongmen deposit (Tafti et al., 2014).
The Xietongmen deposit and the Olitongmen prospect underwent thermal recrystallisation in the hornfels aureole of the Eocene granodiorite batholith. These thermal effects are only of minor significance or are absent at Langtongmen and Newtongmen. Au (ppm): Cu (%) ratios are closely correlated within each of the two main deposits, ranging from 1.5 to 1.2 at Xietongmen to between 0.8 and 0.6 at Newtongmen.
Mineralisation in the Xietongmen district occurred in a number of coeval mineralisation centres. The differences between the mineralised zones are interpreted to reflect exposure at different relative palaeodepths resulting from displacement and deformation by post-hydrothermal, sinistral-oblique movement on thrust faults. Xietongmen was transposed to the south by deformation on and between the bounding Adit-1 and Contact thrust faults. Langtongmen was separated from the deep hanging wall of the Xietongmen deposit and displaced approximately 600 m vertically and 1300 m to the west by the Adit-1 thrust fault. The Newtongmen deposit and the Olitongmen prospect were uplifted relative to Xietongmen and Langtongmen in the hanging wall to the SBF thrust fault and were not significantly deformed.
The information in this section of the summary is drawn from Tafti et al., 2014.
CRETACEOUS DEPOSITS
Lunggar Fe(-Cu)
The Lunggar iron skarn deposit is located ~190 km NE of Zhongba City and ~1000 km WNW of Lhasa in the western section of the Central Lhasa Terrana (Gao et al., 2011). Permian limestones are exposed in the central part of the deposit area, while Quaternary gravels mask much of the country rock to the east and SW.
Intrusive rocks include:
Granodiorite - which has been dated at ~113 Ma in the Early Cretaceous and intrudes Permian limestone. Skarn alteration, which is the host to iron mineralisation is extensively developed along its contact with the Permian carbonate rocks. It comprises grey to white and medium to coarse grained, containing 38 to 44 vol.% plagioclase, 20 to 25% quartz, 15 to 19 vol.% alkali feldspar, 5 to 8 vol.% biotite, 10 to 14 vol.% amphibole and accessory apatite, Fe-Ti oxides, zircon and titanite. It has the characteristics of an I‐type granodiorite, containing high SiO2 and low MgO, with negative Nb, Ta and Ti, and positive Rb, Th, K and Pb anomalies, LILE enrichment and HFSE depletion, typical of crystallisation from a crustally derived magma (e.g., Chappell and White, 2001; Gao et al., 1998). The high 86Sr/87Sri ratios
and negative εNd(t) and εHf(t) values with the TDM2 ages of 1.064 to 1.28 Ga collectively suggest a predominantly Mesoproterozoic crustal source (Cao et al., 2018).
Granite Porphyry - which has also been dated at ~113 Ma, is exposed on the margin of the granodiorite. It is grey to white in colour and has a porphyritic texture. Phenocrysts include 10 to 15 vol.% oligoclase with polysynthetic twinning, 3 to 5 vol.% orthoclase with Carlsbad twinning, 5 to 7 vol.% quartz with corrosion borders, and 3 to 5 vol.% biotite. The matrix shows an aplitic texture and includes microcrystalline plagioclase, quartz and magnetite, with minor apatite, zircon and glass. It has significantly negative anomalies of Eu, Ba, Nb, Ta, Sr, P and Ti, but although these contents could be attributed to the anatexis of supracrustal rocks, the similar Sr-Nd-Pb-Hf isotopic compositions of the coeval granodiorite suggest that they share an identical magma source. The oxide contents of both rocks indicate that the granite porphyry fractionated from a granodiorite melt (Cao et al., 2018).
Diorite - which is located to the NW of the deposit. It is post-iron mineralisation, dated at ~91 Ma, and has been intruded into the granodiorite. It is greyish‐green in colour and is fine‐ to medium‐grained, with a mineralogy of 52 to 64 vol.% plagioclase, 17 to 22 vol.% amphibole, 2 to 5 vol.% quartz, 2 to 6 vol.% alkali feldspar, 1 to 2 vol.% pyroxene and 1 to 2 vol.% biotite. This diorite has intermediate SiO2 (>56 wt.%) and high Al2O3 (>15 wt.%) and Sr (>400 ppm) contents with high Sr/Y (>20) and (La/Yb)N ratios (>10), typical of adakitic magma (Defant and Drummond, 1990). These data indicate enriched LILE and LREE, and depleted in HREE and HFSE and represent relatively enriched whole rock Sr-Nd-Pb and zircon Hf isotopic compositions. Cao et al. (2018) conclude this Late Cretaceous diorite was most likely generated by partial melting of a substantially thickened and then delaminated lower crust, together with the involvement of juvenile material. The thickness of Central Lhasa crust almost doubled during the Late Cretaceous through thrusting and imbrication (Kapp et al., 2003). The increasing (La/Yb)N and Sr/Y ratios are taken to be indicative of thickened crust between ~95 and 80 Ma. The source of the diorite is consistent with partial melting of Central Lhasa lower crust, based on Pb isotopic compositions. Plots of 86Sr/87Sri versus εNd(t) also indicate that the diorite was generated through binary mixing of reworked ancient crust and juvenile mantle materials (Cao et al., 2018). This diorite post dates the main Early Cretaceous skarn iron deposition and is generally coeval with the Late Cretaceous Cu associated mineralisation described below.
A number of orebodies are scattered around the deposit area, which comprises two main orebodies and a few secondary ore zones. The largest of these has a plan area of 300 x 245 m (Fei et al., 2015). Mineralisation is disseminated, laminated, brecciated and massive, dominated by magnetite, with minor hematite, limonite, avasite [5Fe2O3•2SiO2•9H2O], chalcopyrite and malachite. Gangue minerals include diopside, garnet, epidote, phlogopite, actinolite, serpentine, chlorite, quartz and calcite. Alteration assemblages include of skarn, secondary carbonates and silicification. The iron orebody is only gently dipping, overlying the granodiorite and intervening skarn facies.
The information in this summary is principally drawn from Cao et al., 2018.
Ga'erqiong-Galale Cu-Au-Mo
The Ga'erqiong-Galale deposits are located ~30 km east of Geji county, ~1275 km WNW of Lhasa and ~100 km SW of the Bangong-Nujiang suture zone, towards the northern margin of the western Central Lhasa Terrane. Galale is ~3 km ENE of Ga'erqiong.
• Ga'erqiong - The exposed country rock at Ga'erqiong is predominantly the Cretaceous Duo'ai Formation, which is largely composed mainly of marble and limestone and volcaniclastic rocks, mainly tuff, volcanic breccia and volcanic agglomerate. There are no well developed structures in the district, with the most significant being a NE-SW (45 to 55°) trending, 70 to 85°SW dipping F1 fault which is closely related to mineralisation. This structure enclises and is surrounded by cataclasites, lenticular fault-breccias and discontinuous quartz ±sulphide extensional veins.
Intrusive rocks are moderately acidic granitoids, commonly comprising quartz diorite, quartz diorite porphyry, diorite, granodiorite and granite porphyry (the GE granite porphyry). The quartz diorite is the most closely related to mineralisation, with skarn copper-gold mineralisation developed in the contact skarn zone between the quartz diorite and marble or limestone. A concealed quartz diorite porphyry intrudes the quartz diorite and is the main syn-mineral intrusion associated with porphyry type molybdenum (copper) mineralisation. The GE granite porphyry is post-mineral and has a destructive with respect the skarn deposit formed in the earlier stages. Granodiorite and diorite occur as stocks or dikes in the central part of the district, but are apparently unrelated to mineralisation (Zhang et al., 2015).
The quartz diorite has an automorphic-hypidiomorphic granular texture and massive structure, primary composed of 25 to 40% amphibole, 40 to 55% plagioclase and 10 to 15% quartz, with lesser orthoclase, augite and biotite. The GE granite porphyry is variably exposed, occurring throughout the deposit area as ~100 × 150 m lenses, which have a porphyritic texture and massive structure. Phenocrysts are >50% quartz and >40% plagioclase in a matrix dominated by fine grained quartz and feldspar with the lesser K feldspar, biotite and amphibole (Zhang et al., 2015). U-Pb zircon dating gives an age for quartz diorite of 88±2 Ma; and GE granite porphyry of 83±1 Ma (Ouyang et al., 2017).
Skarn Alteration is predominantly composed of garnet, diopside, tremolite, wallostonite, epidote and chlorite, gradationally zoned outward from the intrusive contact as follows, from garnet-diopside-tremolite with chalcopyrite + bornite + pyrite → diopside-wallostonite-tremolite with chalcopyrite + gold + silver → epidote-chlorite-tremolite with gold + silver. The essentially garnet-diopside → diopside-wallostonite → tremonite-epidote zonation reflects a outward gradient from high to low temperatures (Zhang et al., 2015).
Mineralisation at Ga'erqiong occurs in three main orebodies, No. I, II and III. The main orebody, No. I is divided into two parts, an upper part which is dominantly skarn copper-gold developed between the quartz diorite contact and the peripheral unaltered marble and limestone country rock. The lower section is quartz stockwork porphyry molybdenum (-copper) mineralisation within the quartz diorite porphyry. The No. II orebody forms the northwestern margin of the Ga'erqiong complex is is mainly contact skarn mineralisation, with minor molybdenum within quartz diorite porphyry. The No. III orebody is a major, linear, Fe oxide-Cu-Au body within the F1 fault.
The mineralogy of the ores includes chalcopyrite, bornite, magnetite, hematite, molybdenite, native gold, electrum, kustelite, cuprian kustelite, cuproaurite, cuprian electrum and petzite in a gangue of skarn minerals, hornfels.
• Galale - The country rock at Galale comprises the Cretaceous Langjiu and Jiega formations. The former is principally composed of volcanic rocks, including dacite to andesite volcaniclastic rocks and amphibole quartz trachyandesite, while the Jiega Formation, which is the main host to skarn mineralisation, is made up of dolomite, dolomitic marble, peloidal limestone, shelly limestone.
Intrusive rocks include diorite, quartz diorite, granodiorite and granite porphyry (the GL granite porphyry). The latter is not exposed at surface, and appears to be mostly confined to the NE of the deposit area. The granodiorite occurs as a stock which is closely associated with with mineralisation, with skarn alteration developed on its contact with the dolomite and dolomitic marble of the Jiega Formation. The granodiorite has a automorphic-hypidiomorphic granular texture and is massive. It is principally composed of 60% feldspar, 20% quartz, 10% biotite and 7% amphibole. Feldspar is grey-white, and is slabby or columnar upon weathering. Quartz is smokey grey, with irregular grains and a greasy lustre. Biotite is black and flaky with pearly lustre, while amphibole is black and columnar. The GL granite porphyry has a porphyritic texture, with phenocryst comprising 55% plagioclase, 42% quartz and 3% biotite in a matrix of plagioclase and quartz, and minor amphibole and biotite.
Skarn Alteration is largely an assemblage of olivine, serpentine, diopside and phlogopite, with minor pleonaste [(Mg,Fe)Al2O4], tremolite, garnet, epidote, brucite and muscovite, representing a magnesian skarn. There is an outward zonation from the intrusive contact of higher temperature forsterite-serpentine-garnet → diopside-forsterite → lower temperature phlogopite-tremolite-epidote skarn assemblages. This progression is accompanied by a transition of accompanying mineralisation of deep-seated copper (-molybdenum) &rtarr; copper-iron-gold in the middle layer → shallow-seated copper-gold mineralisation (Zhang et al., 2013).
Mineralisation at Galale occurs in three skarn copper-gold orebodies, KT1, KT2 and KT3. KT1 is located at the east central part of the deposit complex and is lenticular, apparently following bedding within the Jiega Formation, with dolomite in the hanging wall and overlies granodiorite which also contains Cu-Au-Fe mineralisation. It strikes at 340 to 355°, and dips at 60 to 75°NE. KT2, which is the smallest of the three orebodies, occurs on the north of the complex and is to KT1, in that it follows bedding within the skarn altered carbonate host, strikes at ~40 to 45° and dips at ~60 to 75°NE. It contains Au-Fe mineralisation. KT3 is located in the SW of the complex, and has a lenticular, apparently conformable shape, trending NW-SE at ~280 to 285° with a dip of 55 to 65°SW. It also has a dolomitic marble hanging wall and granodiorite footwall and carries with Cu-Au-Fe mineralisation.
The ore mineralogy includes magnetite, chalcopyrite, bornite, native gold, electrum, kustelite, cuprian kustelite and petzite, with minor chalcocite, digenite, covelline, limonite, ilmenite and hematite in a skarn mineral gangue (Zhang et al., 2013).
The information in this summary is principally drawn from Zhang et al., 2015.
EOCENE DEPOSIT
Sharang Mo
The Sharang porphyry Mo deposit is located ~200 km NE of Lhasa, and lies in the north eastern section of the Gangdese Metallogenic Belt (#Location: 30° 10'N, 92° 40'E) at an altitude of 4700 to 5300 masl.
Geology. Basement rocks to the Sharang Intrusive Complex, which is related to the porphyry mineralisation at Sharang, is a succession that includes Pre-Ordovician Lower Palaeozoic metamorphic rocks to the south and Late Carboniferous to Early Permian sedimentary rocks to the north. Together these rocks form the older core of the Lhasa Terrane. To the north, this basement is intruded by Mesozoic igneous rocks, including Jurassic monzogranite and Cretaceous syenogranite, granite, porphyritic granite and dolerite dykes, and is overlain by Cretaceous rhyolitic crystal tuff. To the the northeast, these are, in turn, overlain to the Eocene Pana Formation, the uppermost preserved member of the Linzizong Group. The Pana Formation comprises andesitic volcanic and volcaniclastic rocks overlain by a predominantly lacustrine succession of shales, mudstones, sandstones and carbonates (Ingalls et al., 2013).
The porphyry Mo mineralisation at Sharang is hosted by granite porphyry in the southern section of the composite Sharang Intrusive Complex, which was emplaced into the Upper Permian Mengla Formation. The latter is composed of siliceous detrital and carbonate rocks, mostly composed of grey-white metamorphosed quartz sandstone and dolostone.
The Sharang Intrusive Complex is composed of pre-mineral quartz monzonite and quartz diorite; syn-mineral granite, porphyritic granite, granite porphyry, quartz porphyry and aplite; and post-mineral granodiorite porphyry, dacite porphyry and lamprophyre. The post-mineral granodiorite and dacite porphyries intrude the host granite porphyry. The intrusive sequence outlined above is largely based on field investigation and relationships observed in drill core. Dating of pre- and syn-mineral intrusions yield Eocene ages of between 55 and 51 Ma (U-Pb; Zircon; Zhao, Qin, Li, Li, Xiao, Chen, unpublished), while post-mineral intrusions are 22 to 17 Ma, Miocene in age. This indicates the Sharang Intrusive Complex represents at least two separate magmatic pulses.
The syn-mineral granite porphyry, is exposed over an area of 0.75 to 0.9 km2 and intrudes the pre-mineral quartz diorite, quartz monzonite and early-mineral granite. The granite porphyry has 15 to 20% phenocrysts which comprise 5 to 8%, 1 to 5 mm K feldspar; 3 to 5%, 0.5 to 1 mm quartz; 3 to 5%, 0.5 to 5 mm plagioclase; and 1 to 2%, 0.5 to 2 mm biotite. These phenocrysts are set in an 80 to 85% matrix of 35 to 40%, 0.05 to 0.2 mm K feldspar; 25 to 30%, 0.05 to 0.25 mm quartz; 10 to 15%, 0.1 to 0.2 mm plagioclase, and <1% biotite.
Hydrothermal and magmatic breccias occur as pipes in the Sharang deposit. The two types have different textures, mineral composition and alteration styles, although both are gently north-dipping in the granite porphyry.
The main structural features of the Sharang district are syn-ore east-west faulting and post-ore north-south striking faults.
Alteration and Mineralisation - Pervasive hydrothermal alteration related to the Sharang deposit covers an area of ~15 km2 and is zoned from a weak potassic core and an outer propylitic periphery, overprinted by a quartz-sericite-pyrite and an argillic zone. Molybdenum mineralisation is usually associated with both potassic alteration and quartz-sericite alteration zones, but rarely in the argillic assemblage. Veinlet mineralisation is strongly developed within the granite porphyry.
The deposit is composed of several lenticular to tabular ore shells hosted within the granite porphyry. In 2008, 19 holes (totalling nearly 20 000 m) were drilled by Tibet Bureau of Geology and Explortaion in the centre of the granite porphyry stock over an area of 1 km2, and a preliminary resource of 10 Mt @ 0.061% Mo estimated.
Molybdenite occurs as massive, lamellar and small flakes in i). quartz-molybdenite veins, systematically decreasing in grain size and vein thickness from top to bottom; ii). stockwork quartz-molybdenite veins; iii). quartz-molybdenite-pyrite ±anhydrite veins, and iv). spotted and plate molybdenite filling the matrix of hydrothermal breccia. Ore minerals are preddominantly molybdenite, with rare chalcopyrite, pyrite, sphalerite, galena and scheelite, and gangue minerals of quartz, K feldspar, plagioclase, kaolinite, epidote, sericite, illite, anhydrite, calcite and fluorite.
Magmatic breccia is characterised by mineralisation hosted within the magmatic matrix that encloses altered angular or sub-angular intrusive fragments. Argillic and quartz-sericite±pyrite are the two dominant types of alteration seen within the fragments. Quartz-sericite±pyrite altered fragments have also been identified in a hydrothermal matrix in an upper hydrothermal breccia.
In addition to the porphyry Mo deposit at Sharang, a number of skarn or vein-type Pb-Zn-Ag deposits, e.g., Yaguila, Dongzhongsongduo and Dongzhongla are also found in the Sharang district.
The information in this Sharang summary is drawn from Zhao et al., 2012.
HYBRID EOCENE AND MIOCENE DEPOSIT
Jiru Cu-Mo
The Jiru porphyry Cu-Mo deposit is a small low grade occurrence, more of geological than economic interest. It is located ~200 km west of Lhasa, 20 km west of Nanmuling (Namling), and ~50 km NE of the Xietongmen deposit. It lies in the western part of the Gangdese Metallogenic Belt, which is in the central Southern Lhasa Subterrane.
Three main lithologic units are recognised in the immediate Jiru deposit area:
i). An Early Eocene (48.6±0.8 Ma; SHRIMP U-Pb zircon; Zhang et al., 2008; Zheng et al., 2014) granitoid batholith with compositions ranging from quartz diorite to monzogranite, which account for ~60% of the outcrop area within the district, and is part of a regional batholith;
ii). A Late Eocene (36.±0.9 Ma; LA-ICP-MS U-Pb zircon; Wang et al., 2014) barren granite stock located ~2 km to the NE of the ore deposits, intruding the Early Eocene batholith; and
iii). Miocene intrusions (3 samples; 16.6 to 14.1 Ma; U-Pb zircon; Wang et al., 2014; Zheng et al., 2014), composed of monzonitic to monzogranitic porphyry stocks (J1 porphyry) and late-stage monzonite to diorite porphyry dykes (J2 porphyry).
Hydrothermal alteration has been developed in both Eocene and Miocene intrusions, and both Eocene and Miocene mineralisation identified. Three small, but distinct orebodies have been outlined. Two are exposed, with dimensions of 420 x 150 m (Orebody I) and 250 x 30 m (Orebody II). Both abut a NE-SW trending fault and are ~1 km apart. Orebody III is adjacent to Orebody II, but on the opposite side of the fault. It is a tabular deposit that is 20 to 30 m thick, and extends from 160 to 280 m below surface. Together the three orebodies contain a low grade resource of 42 Mt @ ~0.43 wt.% Cu, 0.09% Mo.
The orebodies are hosted by both Early Eocene monzogranite of the granitic batholith and by Miocene monzogranite porphyry, typically along the contact between the two. Later Miocene J2 diorite porphyry dykes are barren and unaltered. The Eocene monzogranites where fresh are pink, with a course to medium grained granitic texture containing 20 to 35% quartz, ~30% alkali feldspar, ~40% plagioclase, ~5% biotite and 2% amphibole. The Miocene J1 monzogranite porphyries are light grey with a porphyritic texture. Phenocrysts are 1 to 2 mm across and constitute ~20% of the rock. They comprise quartz, plagioclase, amphibole and biotite in a matrix of mostly <0.2 mm alkali feldspar, plagioclase, quartz, amphibole and biotite.
Two sets of faults are recognised in the deposit area, a NE-SW and a north-south trending set. The first displaces both the Eocene monzogranite and Miocene J1 monzogranite porphyries and the orebodies, but not the late stage J2 monzonite to diorite porphyry dykes, while the north-south set truncate all lithologies.
Both the Eocene monzogranite and Miocene monzogranite porphyries were intensely altered to produce concentric zones that range from an inner K silicate core, a phyllic quartz-sericite zone and an outer propylitic shell. The K silicate zone is characterised by the replacement of amphibole by euhedral biotite, with or without chalcopyrite, although no K feldspar alteration has been recognised. The propylitic shell has an alteration assemblage of epidote, chlorite, quartz, calcite and clay minerals which replaced biotite, hornblende and plagioclase. The phyllic alteration results in complete replacement of the original mineral assemblage. Biotite is replaced by sericite, quartz and minor chalcopyrite. Plagioclase is altered to sericite. Quartz is the most abundant mineral in the phyllic zone and occurs as several generations of stockwork veins and veinlets and as disseminated grains.
The sulphide minerals at Jiru are predominantly chalcopyrite, molybdenite and pyrite with minor galena and sphalerite, and a gangue of quartz, feldspar, sericite and epidote. Hypogene mineralisation is characterised by early-stage precipitation of chalcopyrite and late-stage deposition of molybdenite (Zheng et al., 2014). Alteration and mineralisation has been divided into three temporal associations, namely: i). K silicate-quartz-chalcopyrite as disseminations, veinlets and stockworks; ii). quartz-molybdenite stockworks with minor chalcopyrite; and iii). quartz-pyrite veining.
Copper mineralisation is largely confined to the potassic zone (Zheng et al., 2014). Accurate isotopic dating of five molybdenite samples collected from the Miocene J1 monzogranite porphyry produced a distinct age of 15.2±0.4 Ma (Re-Os isochron), whereas five molybdenite samples from the Early Eocene monzogranite (after compensating for errors) returned an age of 48 Ma (Zheng et al., 2014). In both cases these are only marginally younger than the host intrusion. Based on the dating results, Zheng et al. (2014) suggest the presence of two episodes of mineralization in the Jiru deposit, and the two-stages of mineralization are considered to be genetically associated with emplacement of the Early Eocene monzogranite and the Miocene J1 quartz monzogranite porphyry, respectively. Preliminary investigation of ore mineral paragenesis indicates that the Miocene mineralising event is relatively more important than that of the Early Eocene at Jiru (Yang et al., 2015).
The information in this Jiru summary is drawn from Zheng et al., 2014, and Yang et al., 2015.
MIOCENE POST-COLLISIONAL DEPOSITS
Qulong / Julong Cu-Au-Mo
Unlike the Jurassic porphyry-style deposits described above, Qulong (also translated as Julong) was developed in a Miocene post-collisional setting, rather than in a subduction related magmatic arc regime.
The magmatic event related to Cu-Mo mineralisation at Qulong has been dated at 21 to 13 Ma, with a mode at 16±1 Ma (Rui et al., 2003, 2004; Hou et al., 2004). As such, this event post-dates the most recent subduction-related arc magmatism by at least ~50 Ma, but is alsolater than the mid-
Miocene ultrapotassic magmatism that was initiated at 25 Ma (Miller et al., 1999; Turner et al., 1993). This post-collisional magmatism was coeval with rapid uplift and exhumation of the Gangdese arc batholiths at 21 to 18 Ma (Copeland et al., 1987; Harrison et al., 1992), east-west extension that started at ~18 Ma (Williams et al., 2001) and molasse deposition at 20 to 19 Ma (Harrison et al., 1992). The Cu-Mo mineralisation at Qulong has a similar age to the host post-collisional felsic stocks, indicating a direct genetic connection (cf. Hou et al., 2009).
Geology - The principal lithologic units in the Qulong district are:
► Early Jurassic granite porphyry (183.3 Ma) with an outcrop area of 1.5 km2. This intrusive is characterised by more felsic minerals (quartz and K feldspar) and only minor dark minerals. Phenocrysts are dominantly 1 to 3 mm quartz and 1 to 4 mm, up to 1 cm K feldspar, accounting for 15 to 25% of the whole rock. Whole rock analyses show the rock contains
about 74% to 82% SiO2, 3.3% to 6.5% K2O and 2.4% to 4.3% Na2O, and belongs to a high-K calcalkaline series (Yang et al., 2008)
► Early to Middle Jurassic Yeba Formation, which is up to 3000 m thick and covers ~60% of the surface area of the district, where it generally strikes east-west. Lavas of the formation have been dated at 174.4±1.7 Ma (Dong et al., 2006), and are geochemically similar to volcanic rocks in arc settings, with enrichment of Large Ion Lithophile Elements (LILE) and depletion of High Field Strong Elements (HFSE). Consequently they are interpreted to be the earliest products of northward subduction of the Neo-Tethyan Ocean (Dong et al., 2006). They can be divided into three lithologic members:
• 'Basal' Member - andesitic crystal tuff, ignimbrite and dacite with intense contact metamorphic hornfels, mainly restricted to the south-central part of the district where it hosts most of the intrusive rocks at Qulong.
• 'Second' Member - predominantly composed of medium to thinly laminated slate with minor interbeds of sandstone and limestone. It is mainly found to the north of the Qulong deposit, but also can be found to the south.
• 'Top' Member - andesitic lava and volcaniclastic rock, that is largely restricted to the area north of the deposit.
► Miocene intrusive rocks, including:
• Rongmucuola Pluton, outcropping in the central part of the Qulong deposit, where it strikes west-west and is the largest Miocene intrusion in the district. It varies in composition from granodiorite to monzogranite and intrudes the Basal member of the Yeba Formation, producing strong hornfelsing. It appears to comprises several phases, with the eastern segment of the pluton typically having a medium to coarse, hypidiomorphic-granular texture consisting mainly of medium to coarse-grained (3 to 8 mm) plagioclase (35 to 40 vol.%), alkali feldspar (25 to 30 vol.%), quartz (15 to 20 vol.%), minor biotite (5 to 10 vol.%) and hornblende (~5 vol.%). The western part is weakly porphyritic with 35 and 40 vol.% phenocrysts of abundant medium- to coarse-grained (3 to 7 mm) plagioclase (~20 vol.%), lesser medium-grained (0.4 to 3 mm) quartz (~10 vol.%), biotite (~5 vol.%) and hornblende (>5 vol.%), all set in a quartzo-feldspathic groundmass with micro-subhedral granite texture (0.2to 1 mm).
Despite the textural variations, all of these phases are mineralogically and compositionally similar and are characterised by a light grey colour.
Mafic phases or enclaves are common within the pluton, mainly composed of plagioclase, hornblende and lesser biotite, with accessory apatite, magnetite and chalcopyrite, and minor titanite, rutile and zircon and anomalous Cu levels. These enclaves mostly range from 1 to 20 cm across and have angular to ovoid shapes, with local 2 to 5 mm wide chilled borders. The chilled margin differs from the interior of the enclaves only in grain size. The Rongmucuola pluton is cut by all of the other Miocene porphyries, with intense superimposed hydrothermal alteration surrounding the later intrusions. The pluton hosts at least 70% of the 7 Mt Cu metal in the orebody.
• P Porphyry - The Rongmucula Pluton was intruded by monzogranite porphyries that are interpreted to be coeval with the main mineralisation. This monzogranite is divided into the early P Porphyry and later X Porphyry largely on the basis if crosscutting relationships. The former is the largest Miocene porphyry at Qulong, and outcrops in the core of the deposit over an area of 0.2 km2, occurring as a complex, steep-walled, mushroom-shaped stock that is ~500 m in diameter. It is composed of from 25 to 30 vol.% phenocrysts that comprise ~15 vol.% plagioclase, 5 to 7 vol.% quartz, and 3 to 5 vol.% K feldspar and <3 vol.% biotite, all set in a quartzo-feldspathic groundmass that has a fine-grained aplitic texture at shallow levels. Feldspar phenocrysts are generally tabular and ~1 to 5, up to 8 to 10 mm across with minor embayed shapes. Quartz phenocrysts are commonly rounded and embayed and from 2 to 4 mm in diameter. Biotite phenocrysts are euhedral brown flakes with distinct cleavage and <2 mm across.
The P Porphyry has been subjected to intense potassic and feldspar destructive alteration and is mostly strongly veined (5 to 10%, mainly quartz A veins) and is generally well mineralised.
Unidirectional solidification textures (UST) are observed in the P Porphyry, comprising 2 to 50 mm thick sinuous bands of crenulated, coarse-grained prismatic (comb) quartz interbanded with the porphyry. These quartz veins generally vary from 0.2 to 0.3 mm in width, and although the internal texture is not distinct, can be shown to have single-directional growth.
• X porphyry, is characterised by more biotite phenocrysts and sparser (<1 vol.%) quartz veins compared to the P Porphyry, and occurs as dykes that range from a few to 50 cms in thickness. Most are irregular and discontinuous, not being traceable between drill holes. Irregular quartz veins within the porphyry have K feldspar selvages and are truncated by marginally younger quartz, K feldspar and biotite veinlets. It has been subjected to intense potassic and weak feldspar-destructive alteration, and is only weakly mineralised. Relationships observed suggest emplacement between early K feldspar and later biotite alteration stages. The porphyry has 15 to 20 vol.% phenocrysts, comprising ~8 vol.% plagioclase, 3 to 5 vol.% biotite, ~3 vol.% K feldspar and ~3 vol.% quartz in a quartzofeldspathic groundmass. Unidirectional solidification textures are also evident, characterised by continuous veins of single-directional comb quartz occurring at the X Porphyry to Rongmucuola pluton contact.
• Aplite, which occurs as 1 to 50 cm wide, irregular fracture-controlled dykes composed of intergrowths of fine-grained anhedral alkali feldspar and quartz with disseminated magnetite and pyrite. Some of these dykes have thin (1 to 2 mm) selvages of barren prismatic quartz. Crosscutting relations suggest it is coeval or slightly older than the X Porphyry, but it is cut by irregular to planar quartz-K feldspar ±anhydrite veins that accompany K silicate alteration.
• Diorite porphyry is a post-ore intrusion and the youngest in the deposit. Its texture is preserved, and it occurs as 2 to 6 m thick dykes, restricted to the centre of the deposit. Phenocrysts comprise 7 to 8% of the rock and consist of ~5% tabular, 1 to 5 mm (locally to 20 mm), crystals of plagioclase, 2 to 3% quartz, <1% tabular hornblende and <1% biotite, set in a groundmass of plagioclase and hornblende, with lesser quartz and biotite.
• Igneous breccias - occurring as both continuous and discontinuous circular pipes that are commonly distributed along the western flank of, or above, the P Porphyry. These have been divided into two main types, namely: i). breccias cemented by monzogranite, containing angular fragments that are mainly <2 cm in size and comprise Rongmucuola Pluton, X Porphyry and quartz-K feldspar-molybdenite veins. The fragments, especially quartz-K feldspar veins, are commonly displaced, indicating displacement during brecciation. This group of breccias were apparently emplaced during K silicate alteration, as indicated by abundant irregular quartz-K feldspar veins; and ii). those cemented by aplite, containing subrounded fragments that are generally <10 cm across, are composed of Rongmucuola Pluton, some of which contain irregular quartz-K feldspar veins, but also planar quartz-anhydrite-molybdenite veins. These breccia were emplaced during the sericitic alterations stage, as implied by the presence of abundant sericite in the aplite cement. The breccias within the deposit average ~0.3% Cu , which is slightly less than the average grade of the deposit i.e., ~0.5% Cu.
Geochemical analyses indicate the residual Miocene intrusions at Qulong show a good correlation between SiO2 and other major oxides, indicating they have a similar origin and probably formed from fractionation in a common magma chamber.
Alteration and Mineralisation - began with early, dominantly potassic hydrothermal alteration with peripheral propylitic, and local calc-silicate assemblages, followed by later feldspar destructive alteration. The calc-silicate alteration is characterised by hydrothermal garnet, calcic plagioclase, diopsidic pyroxene, epidote and idocrase, mainly affecting the carbonate rocks of the second member of the Yeba Formation in the southern part of the deposit. The main alteration events and styles are as follows:
► Potassic alteration, represented by both pervasive and vein/veinlet development of potassic mineral assemblages in the deep and central sections of the Qulong intrusions, subdivided into:
• Early K feldspar Alteration - which formed after the intrusion of the P Porphyry, occurring as pervasive or selectively pervasive replacement, and emplacement of veins and veinlets, mainly in the P Porphyry and Rongmucuola Pluton, and less extensively in the Yeba Formation. Selectively pervasive alteration throughout much of the Rongmucuola Pluton and P Porphyry involved partial replacement of plagioclase by K feldspar, usually filling micro-veinlets and healing discontinuous, minute stockwork-like fractures, as well as replacing primary feldspar along twinning planes or rims. Pervasive alteration is best developed in the central core of the alteration system, involving the complete destruction of the original minerals and textures and the formation of an assemblage of a flesh-coloured K feldspar, quartz and minor biotite. Vein or veinlet K feldspar is mainly found within the Rongmucuola Pluton, and where well developed occurs as K feldspar ±quartz, quartz-K feldspar ±anhydrite, barren quartz, and quartz-K feldspar-molybdenite ±anhydrite. Of these, the K feldspar±quartz assemblage is the earliest, occurring as irregular and discontinuous, generally <1 mm thick veins. They are cut by irregular to planar, discontinuous, 1 to 4 mm thick barren, granular quartz veins, and by 1 to 5, up to 15 mm wide quartz-K feldspar±anhydrite veins. Two generations of the latter are recognised. The earlier has irregular walls and an obvious selvage of K feldspar, whereas the later is characterised by regular walls and white centre lines of K feldspar. These veins are all cut by planar, 4 to 10 mm thick quartz-K feldspar-molybdenite ±anhydrite veins, characterised by the presence of disseminated molybdenite.
• Later Biotite Alteration - which was formed after the intrusion of X Porphyry and is dominantly found in the Rongmucuola Pluton and X Porphyry. It is characteristically composed of biotite, anhydrite, quartz and carbonate and occurs in three main forms, i). continuous vein fill, or discontinuous hairline veinlet fill of medium-coarse grained (0.1 to 0.4 mm) yellow-brown biotite, accompanied by chalcopyrite; ii). halos enclosing irregular quartz or quartz-anhydrite veins; and iii). selective replacement of primary biotite and hornblende phenocrysts, or groundmass feldspars. In the second and third modes, alteration biotite is fine-grained and bright brown, characterised by its shreddy shape. Other fine-grained secondary biotite alteration is difficult to recognize in hand specimen.
Veining associated with biotite alteration comprise a). chalcopyrite ±biotite, which are <0.1 mm thick and occur throughout the Rongmucuola Pluton. accompanied by disseminations of similar composition; b). quartz-anhydrite ±K feldspar ±chalcopyrite ±pyrite, which are 3 to 6 mm thick irregular to planar veins characterised by abundant anhydrite and a broad biotite selvage; c). biotite veinlets, the most significant biotite veining, that are 0.5 to 1 mm thick and are predominantly found within the Rongmucuola Pluton and X Porphyry, and truncate early-stage K feldspar associated veining.
► Propylitic alteration is very well developed, and affects the periphery of the deposit. It has been subdivided into two types depending on the wallrocks, as follows:
• within the Yeba Formation, propylitic assemblage is characterised by intense epidote alteration of plagioclase, but only poor chlorite development, and mainly occurs as veining and selectively replacement. Veins are generally 1 to 2, and locally up to 6 mm thick, and irregular to planar. They mainly comprise 60 to 80% epidote and 15 to 30% quartz, with lesser chlorite and K feldspar. Selectively replacement is characterised by strong overprinting of plagioclase phenocrysts and broken crystals by assemblages of epidote, quartz and lesser chlorite. Epidote also locally replaces plagioclase in the volcanic groundmass. Sulphides are dominated by euhedral pyrite, which it also occurs in the absence of alteration, suggesting it may not entirely be an alteration product.
• propylitic alteration the Rongmucuola Pluton is characterised by selective replacement of biotite, hornblende and plagioclase by a chlorite-epidote ±carbonate assemblage. Biotite is progressively replaced along flake margins or cleavage planes by both chlorite and carbonate, whilst plagioclase and hornblende are partially and only weakly converted to epidote and calcite. No sulphide mineralisation is observed. Compared to the Yeba Formation, propylitic alteration in the Rongmucuola Pluton is very weak.
► Phyllic ± argillic alteration is pervasive, or occurs as selvages to planar pyrite-quartz and chalcopyrite-pyrite veins. It overprints both fresh rocks and the potassic alteration and part of the propylitic halo, and affects all of the porphyry types, including the latest diorite porphyry. Alteration assemblages are dominated by quartz, sericite and pyrite, but also include chlorite and calcite, locally with lesser anhydrite, rutile, apatite and clay minerals. The intensity of this alteration varies from incipient replacement of mafic minerals to almost complete obliteration of the igneous texture. Intense alteration of this type mainly occurs in the P Porphyry and surrounding Rongmucuola Pluton. It is characterised by complete destruction of all silicate minerals, except quartz. Feldspars are pervasively replaced by fine-grained sericite and abundant ultra-fine granular quartz, with lesser anhydrite, calcite, rutile and clay minerals, or alternatively, mainly by sericite and kaolinite. Biotite is replaced by chlorite, sericite, quartz and rutile. Some quartz in the matrix may be secondary, resulting from the breakdown of K feldspar to muscovite and quartz. Pyrite is ubiquitous, occurring as disseminated grains and in veinlets. Strong phyllic alteration also occurs as selvages to pyrite-quartz and chalcopyrite-pyrite veins and may affect large volumes where the veins are closely spaced. Strongly alteration of this type is surrounded weak development, largely in the form of sericite and calcite occurring along hairline fractures. Veining that is genetically associated with phyllic ±argillic alteration include planar D veins of molybdenite-quartz ±pyrite ±chalcopyrite, chalcopyrite-pyrite ±anhydrite and pyrite-quartz ±anhydrite.
► Hypogene Mineralisation - The bulk of the mineralisation at Qulong is closely associated with potassic alteration. The dominant copper mineral is chalcopyrite, with lesser bornite, occurring as disseminations, as hairline fracture fillings or as centreline fillings in veins. Vein and veinlet types include discontinuous chalcopyrite±biotite which are associated with biotite alteration; and planar quartz-anhydrite-chalcopyrite±molybdenite±pyrite and chalcopyrite-pyrite ±anhydrite that generally have chlorite-sericite selvages. All of these veins are generally 1 to 3 mm thick, but may be up to 10 mm. Chalcopyrite ±biotite micro-veinlets are by far the most common, especially in Rongmucuola Pluton away from the P Porphyry, and account for ~60% of the Cu in the deposit. The presence of chalcopyrite-pyrite ±anhydrite veins suggests an economically significant amount of Cu also formed during chlorite-sericite alteration.
Molybdenum was deposited during the transition from potassic to phyllic alteration, slightly later than Cu mineralisation. The principal molybdenum mineral is molybdenite, with lesser molybdine (or ferrimolybdite; Fe2(MoO4)3•nH2O) at surface. Molybdenite is predominantly found as centreline fillings in planar quartz-molybdenit ±anhydrite ±chalcopyrite ±pyrite veins that are generally 4 to 20 mm thick, but may be up to 40 mm; and 2 to 10 mm thick quartz-anhydrite-chalcopyrite ±molybdenite ±pyrite veins which together account for ~60% of the contained Mo metal in the deposit. These veins are predominantly found within Rongmucuola Pluton and Yeba Formation. Although they generally lack alteration selvages, they usually have weak and irregular bleached halos. These halos may include biotite and occassionally sericite, suggesting the veins formed at the transition from potassic to phyllic alteration, and are probably B or C veins. Rare, continuous, planar, 1 to 2 mm thick molybdenite-quartz ±pyrite ±chalcopyrite veins with sericite-chlorite selvages suggest a small proportion of the molybdenite formed during phyllic alteration.
The information in this Qulong / Julong summary is drawn from Selby et al., 2013; Xiao et al., 2012; and Yang et al., 2009.
Yang et al. (2009) quoted a resource at Qulong / Julong of 1420 Mt @ 0.5% Cu;
The Zijin Mining website (viewed February 2024) quoted total Mineral Resources (including Ore Reserves) as 18.92 Mt of contained copper from ore with an average grade of 0.33% Cu; 1.16 Mt of contained Mo at an average grade of 0.02% Mo and 13 145 tonnes of contained silver at a grade of 2.51 g/t Ag. These figures equate to an ore tonnage and grade of 5.73 Gt ore @ 0.33% Cu, 0.02% Mo, 2.51 g/t Ag.
Jiama Cu-Mo
The Jiama porphyry/skarn Cu-polymetallic system is located in Metrorkongka County, in the Tibet Autonomous Region of China, ~68 km east of Lhasa and ~25 km NE of the Qulong deposit described above.
Small-scale lead mining had historically taken place in the immediate Jiama district prior to the 1950s. Geological work between 1951 and 1990, mainly surface trenching, delineated a 3000 m long belt of Cu-Pb-Zn mineralization. Subsequent investigations identified potential for skarn-type Cu-Pb-Zn mineralisation in the district, and the target was drilled, a resource estimated in 2000 and mining commenced soon after. Further work revealed a 10 km2 area of hornfelsing, and skarn and silicate alteration immediately to the east of Lead Mountain, which was regarded as prospective for porphyry and skarn copper mineralisation. Subsequent exploration and evaluation drill programs outlined a composite deposit of Cu-polymetallic skarn, Cu-Mo hornfels and porphyry Mo ±Cu orebodies (Tang et al., 2010, 2013; Zheng et al., 2010, 2012). The established resource comprises ~60% skarn, ~35% hornfels and ~5% porphyry hosted mineralisation in the resource. Six elements (Cu,Mo, Au, Ag, Pb, and Zn) can be extracted, and the deposit is regarded as economic in China.
Measure + Indicated + Inferred Resources are as follows (Zheng et al., 2016):
Skarn ore - 621.3 Mt @ 0.64% Cu, 0.04% Mo, 0.15% Pb, 0.09% Zn, 0.25 g/t Au, 14.69 g/t Ag;
Hornfels ore - 1184.4 Mt @ 0.27% Cu, 0.03% Mo, 0.01% Pb, 0.01% Zn, 0.04 g/t Au, 1.55 g/t Ag;
Porphyry ore - 86.5 Mt @ 0.30% Cu, 0.04% Mo, 0.01% Pb, 0.01% Zn, 0.07 g/t Au, 2.91 g/t Ag;
TOTAL RESOURCE - 1892.2 Mt @ 0.39% Cu, 0.03% Mo, 0.06% Pb, 0.04% Zn, 0.11 g/t Au, 5.92 g/t Ag;
Contained metal - 7.4 Mt Cu, 0.6 Mt Mo, 1.8 Mt Pb+Zn, 205 t Au, and 11200t Ag.
Note: Zheng et al. (2016) and Yao et al. (2018) use the term 'hornfels ore' to describe the outer shell of the porphyry style mineralisation developed within hornfelsed country rock. The hornfels is significantly more extensive than the porphyry system and is the result of pre-mineral thermal contact metamorphism of sedimentary and volcanic country rocks by a concealed granitic pluton.
Geology. The stratigraphy of the sequence hosting the intrusive complex that accompanied mineralisation and Jiama is as follows, from the base:
• Lower to Middle Jurassic Yeba Formation - up to 7700 m of massive andesites, tuffaceous dacite, and lens of thin-bedded limestones;
• Upper Jurassic Duodigou Formation - 200 to 1700 m of limestones;
• Lower Cretaceous Linbuzong Formation - 110 to 1500 m of sandstones and sandy slates;
• Lower Cretaceous Chumulong Formation - 370 to 900 m of quartz sandstones, siltstones, and black shales;
• Quaternary colluvium and alluvium.
Few of the intrusive igneous rocks associated with the deposit are exposed at surface, most occurring as dykes and stocks and are only encountered in drill core. Together they form an irregular, composite, forked intrusive mass with a plan area of ~800 x <100 to ~350 m, surrounded by other separate dykes and smaller stocks. The main intrusive phases include:
• Granite porphyry - which contains ~30% to 35% phenocrysts, including 15 to 20% plagioclase and 10% K feldspar, with 1 to 5% quartz, <3% biotite and <2% amphibole. These phenocrysts are unevenly distributed and show intense corrosion. They are set in an aphanitic felsic matrix, dominated by ~30% K feldspar, >20%quartz and ~10% plagioclase, with <3% biotite and <2%amphibole, with accessory zircon, titanite, magnetite ±ilmenite ±apatite.
• Monzonitic granite porphyry - containing ~30% phenocrysts, mainly ~15% K feldspar and (<10% plagioclase , with ~5% quartz and <2% biotite, set in a fine-grained, holocrystalline, felsic matrix which has a granular texture, and is dominated by ~ 20% K feldspar, ~20% plagioclase and quartz, ~5% biotite, <2%amphibole and accessory zircon, titanite, apatite, magnetite ± ilmenite.
• Granodiorite porphyry - comprising 20 to 25% phenocrysts of mainly >15% plagioclase and <5% amphibole, with <3% quartz in a microgranular, felsic matrix that is dominated by >45% plagioclase, ~20% quartz and <15% amphibole, with minor <3% biotite and accessory zircon, titanite, apatite and magnetite ±ilmenite.
• Quartz diorite porphyry - containing <5 to 20%, widely variable phenocrysts of mainly plagioclase and amphibole, with minor quartz and biotite in a fine-grained, hypidiomorphic-granular dioritic matrix dominated by plagioclase and amphibole, with lesser quartz, biotite and K feldspar and accessory zircon, titanite, apatite and ilmenite ±magnetite.
The Quartz diorite, Granodiorite and Monzonitic granite porphyries are calc-alkaline to high-K calcalkaline in composition and are quasi-aluminous. They have a systematic enrichment in LILE (Large Ion Lithophile Elements) and depletion of HFSE (High Field Strong Elements), weak negative Eu anomalies, and characteristics consistent with I-type granites. In contrast, the Granite porphyry has a high-K, alkaline, peraluminous composition, with negative Eu anomalies and Rb/Sr ratios similar to those of S-type granites.
Structure, principally a thrust nappe and associated gliding nappe, were important controls on the development of the Jiama mineralised system. The thrust nappe at Jiama is characterised by limbs of overturned clastic and carbonate rocks of the Upper Jurassic and Lower Cretaceous sequence. This deformation apparently took place at ~50 Ma (Zhong et al., 2012), close to commencement of India-Asia continental collision (Mo et al., 2002; 2007; Yin and Harrison, 2000). The Yeba Formation in the footwall of the nappe is dominated by a series of overturned folds with north-dipping axial planes, indicating south vergence. The Jiama deposit lies in the front and intermediate zones of the thrust nappe structure. This structure led to the development of an interlayer detachment zone between the folded upper nappe limb in sandstones and slates of the Linbuzong Formation and the footwall carbonates of the Duodigou Formation which, in turn, overlies the Yeba Formation. Intense deformation at the base of the preserved Linbuzong Formation is taken to be evidence of this detachment. The detachmment is the focus of skarn alteration and mineralisation, as described below (Duan et al., 2014; Zhong et al., 2012). The Niumatang overturned anticline in the thrust nappe structure was the primary control on porphyry intrusion, responsible for the formation of the #1 skarn, hornfels and porphyry orebodies.
A single 4 x 1 km, ESE-WNW elongated gravity induced gliding nappe was developed in the Copper Mountain section of the district, covering an area of 4 km2. This structure was formed as a result of northward slippage of the unstable uplifted nose zone of the thrust nappe (Zhong et al., 2012). The gliding nappe is only discernible at the peak of Copper Mountain, where only Duodigou Formation limestone and Linbuzong Formation slate (<100 m thick) are exposed. The gliding nappe can be divided into three zones from north to south: the front, intermediate and rear zones (Zhong et al., 2012). The front zone has many Z-folds and cleavage structures, which provided sufficient space for the transport and deposition of ore-forming fluids, and controlled the formation of the #2 skarn orebody.
Ore Styles and Characteristics - Three main styles of mineralisation are recognised at Jiama:
• Skarn Cu-polymetallic, comprising the #1 and #2 skarn orebodies. The #1 skarn orebody is localised at the contact between porphyry and marble, and in the interlayer detachment zone between the hornfels and marbles of the Linbuzong and Duodigou formations respectively. It is located at elevations of 4000 to 5000 masl and occurs as a layer, or thick tabular plate that strikes WNW and dips to the NE. The upper part of the orebody dips steeply at 60 to 80°, while the base is flatter at 10 to 30°. It has a strike length of ~3000 m and down dip extent of 2500 m. The thickness of the orebody where it follows the interlayer detachment is generally 10 to 50 m, although it reaches 50 to 100 m in the anticlinal core. Where it follows the contact between the intrusion and marble, it is typically >100 m thick, with a maximum of 328 m.
The #2 skarn orebody is lens-shaped and occurs in the front zone of the Copper Mountain gliding nappe, at elevations of 4600 to 5100 masl. It strikes east-west, dips 50 to 80°S, and is generally >100 m thick. The Cu resource is at ~50 Mt @ 0.98% Cu and is suitable for open-pit mining. In the middle to rear of the gliding nappe, a small, <10 m lens-shaped skarn has been intersected.
A number of other smaller, lenticular shaped skarn-type mineralised bodies which have also been identified below and to the SE of the #1 body, are generally discontinuous and of limited strike (<200 m) and thickness.
It is uncertain from the literature studied, whether the prograde skarn is related to the mineralised porphyry system or the larger concealed pluton that produced the extensive hornfels described below.
• Porphyry Cu ±Mo, which is porphyry style mineralisation predominantly hosted within and marginal to intrusions. It occurs at elevations of <4800 m in the deeper core of the mineralised system, centred on a cone shaped swarm of Quartz-diorite, Granodiorite and Monzonitic-Granite and Granite porphyry dykes. The orebody is ~900 x 200 m, elongated north-south (although the actual width varies from 14 to 361 m), and extends for >600 m vertically.
• Hornfels Cu-Mo, which is porphyry style mineralisation hosted by hornfelsed sedimentary and volcanic wall rocks, and is located at elevations of 4300 to 5200 masl, surrounding the porphyry centre. It occurs as a massive, irregular and vertically-orientated 'cylindrical' body, above and flanking the composite porphyry intrusion swarm. It has a 1220 m NW-SE strike length, width of ~800 m, subvertical dip, and has been intersected over a vertical interval of up to 940 m. This mineralisation occurs within a larger district wide thermal metamorphic aureole covering a maximum area of about 40 km2, induced by a concealed pluton (Wang et al., 2011).
Alteration - The following alterations styles have been differentiated within the mineralised system:
• Potassic alteration - is predominantly found in ore-bearing porphyry dykes and in adjacent country rock. It generally does not persist for >500 m from an altered porphyry. Skarn in contact with mineralised dykes is also subjected to potassic alteration, but generally for only <100 m from a dyke. The dominant potassic mineral is hydrothermal biotite. Two main styles of potassic alteration are recognised: i). quartz-biotite-chalcopyrite ±plagioclase ±albite ±pyrrhotite ±pyrite ±magnetite ±molybdenite veins, which occur in porphyry, hornfels and skarn; and ii). fine-grained hydrothermal biotite, typically occurring as <0.5 mm aggregates with chalcopyrite, pseudomorphing replaced amphibole and plagioclase. A-type veins are rare, with the bulk of the quartz veins being B-type veins.
• Propylitic alteration - which occurs peripheral to the potassic and phyllic zones, and is characterized by abundant hydrous minerals. Three styles are delineated: i). quartz-epidote-chlorite-pyrite ±actinolite ±chalcopyrite veins, which commonly occur in the hornfels and porphyry; ii). quartz-chlorite-biotite-chalcopyrite-pyrite that are disseminated in the countryrock where chalcopyrite is relatively abundant; and iii). chlorite and epidote derived from partial metasomatism of plagioclase phenocrysts in porphyry dykes.
• Phyllic alteration, which occurs in the altered country rock/hornfels above the potassic zone and in some porphyry dykes. Two variations are recognised: i). quartz-sericite-pyrite ±chalcopyrite ±calcite ±anhydrite veins in the hornfels/country rock; and ii). partial alteration of plagioclase phenocrysts in dykes to a sericite-quartz-pyrite assemblage. D veins dominate in the phyllic alteration zone, with lesser B veins. Sulphide minerals in the D veins have a strong spatial zonation, from central chalcopyrite-rich veins overprinting potassic alteration, grading vertically to pyrite-chalcopyrite veins, and finally to pyrite-dominated veins containing variable amounts of galena, sphalerite, tetrahedrite and rhodochrosite.
• Argillic alteration - occurs above the phyllic zone in the upper section of the country rock/hornfels mineralisation and in some porphyry dykes. More intense alteration in the country rocks produces an assemblage of kaolinite and illite, whilst in the porphyry dykes, feldspar phenocrysts are ultimately altered to kaolinite. At the top of the argillic zone, ~50 m from the surface, a small lithocap covering an area of ~0.01 km2 has been formed, characterised by porous secondary quartz and locally containing alunite.
• Skarn alteration - occurs in the form of both exo- and endoskarn.
Endoskarn mainly occurs as epidote alteration in the contact between carbonate rocks and Granite or Quartz-Diorite porphyries. In the contact zone of Granite Porphyry dykes, the epidote alteration is cut by massive andradrite veins, whereas the Quartz-Diorite Porphyry contact skarn is dominated by the epidote alteration.
Exoskarn is laterally most extensively developed where it follows the detachment between the carbonate rocks of the Duodigou Formation and the structurally overlying Linbuzong Formation outward from the intrusive contact. It occurs as both prograde and retrograde phases. Prograde exoskarn is predominantly composed of garnet, pyroxene and wollastonite. It has been subdivided into three transitional zones outward from the intrusive contact, namely proximal, intermediate and distal garnet-pyroxene zones, whilst wollastonite occurs as a fourth zone below all three zones in the contact transition to the underlying marble. The composition of each of these four zones is as follows (From: Zheng et al., 2016):
Pyroxene is not as variable as garnet, mainly occurring as light green to white diopside with a maximum hedenbergite content of ~20% and an average composition of Diopside 88.6; Hedenbergite 8.9; Johannsenite 2.5, although at the margin of the skarn there may be up to ~60% hedenbergite.
Retrograde skarn results in an overprinting assemblage of amphibole, epidote and chlorite, with a late sulphidation stage with calcite, anhydrite and sulphides.
Mineralisation within skarn occurs as disseminated sulphides enveloping thin (<10 cm) thick high grade vein sets of massive sulphides. Disseminated gold and silver share a similar distribution to that of copper, while molybdenum has a negative correlation.
Veining and Mineralisation in each of the ore styles differentiated, is as follows:
• Skarn Cu-polymetallic - The main economic ore minerals are molybdenite, chalcopyrite, bornite, tetrahedrite, chalcocite, galena and sphalerite. Gangue minerals include garnet, wollastonite, diopside, tremolite, chlorite, epidote, quartz and calcite. Four types of veins are found in the skarns: Type I - quartz + sulphide; Type II - wollastonite + quartz + sulphide; Type III - quartz + garnet ±wollastonite ±epidote ±sulphide and Type IV - quartz ±garnet ±sulphide. Type I veins have clear wall rock contacts, mainly dip at <40°, and are widespread throughout the skarn. Type II, III and IV veins are primarily found close to the porphyry centre and have regular morphologies. Some veins dip steeply. Garnet, wollastonite, chlorite and epidote are observed in the outer edges of type II, III and IV veins. Type I veins in the skarns are cut by all of the other types (Yao et al., 2018).
• Porphyry Cu ±Mo mineralisation primarily occurs as veins or stockworks, with the main ore minerals being chalcopyrite and molybdenite, with a gangue of quartz, feldspar, biotite, sericite and chlorite. Vein types, as described by Yao et al. (2018) at Jiama include EB veins (early barren, irregular quartz veins with biotite), A veins (irregular quartz veins with diffuse margins containing albite, K feldspar and quartz-sericite selvages ±chalcopyrite ±pyrite), B veins (veins with sharp boundaries and selvages of biotite and chlorite, with a variety of associated assemblages, including quartz-biotite ±chalcopyrite ±pyrite; quartz-chlorite ±chalcopyrite ±pyrite; quartz ±anhydrite-chalcopyrite-molybdenite; and quartz-molybdenite) and D veins (regular, tabular veins that contain quartz-molybdenite and barren quartz) veins. However, these veins, as described by Yao et al. (2018) appear to represent a number of pulses of hydrothermal activity, and reopening and overprinting of earlier veins as they include both potassic and phyllic alteration assemblages.
• Hornfels Cu-Mo ores typically occur in veins or stockworks, similar to the that of the underlying porphyry orebody. Sulphide minerals include chalcopyrite and molybdenite, in a gangue that includes quartz, feldspar, biotite and chlorite. Three types of veins have been identified: Type a) quartz with biotite, chlorite or siliceous selvage ±pyrite ±pyrrhotite ±chalcopyrite; Type b) quartz quartz with biotite, chlorite or siliceous selvage +pyrite ±chalcopyrite ±molybdenite; and Type c) quartz ±pyrite ±chalcopyrite ±molybdenite. Type a) veins, which are widespread in the deposit area, are typically follow bedding and are parallel to each other, with irregular morphology and diffuse wall rock contacts, but possess selvages. Type b) veins transect bedding and have regular morphology and diffuse wall rock contacts. Alteration halos are commonly found along the vein walls. These veins are most prevalent close to the intrusion centre. Type c) veins have regular morphology and distinct wall rock contacts with no selvages. Type c) veins truncate types a) and b) veins, and are most abundant near the intrusion centre.
Crosscutting relationships between vein types in the different ore styles, as well as fluid inclusion studies of veins, allow correlation and a sequence of deposition to be established. Yao et al. (2018) concluded that two hydrothermal stages and two corresponding episodes of fluid boiling took place at Jiama. The first boiling event occurred during the early hydrothermal stage, as recorded by fluid inclusions from A veins in the porphyries, type a) veins in the hornfels, and wollastonite in the prograde skarns. This fluid boiling event was only accompanied by relatively weak
mineralisation and only occurred in the central part of the deposit. The second boiling event occurred in the late hydrothermal stage, as determined from
fluid inclusions hosted in B and D veins in the porphyries, type I to IV veins in the skarns, and type
b) and c) veins in the hornfels. Both boiling stages took place at from 450 to 220°C in vapour and liquid phases with variable salinities from low (<1 wt.%) to high (>40 wt.% NaCl equiv.). H-O isotope data show a trend of groundwater mixing with magmatic fluid in the late hydrothermal stage, which is interpreted to have led to a temperature and salinity decrease, and an increase in pH, resulting in precipitation of ore minerals. The late boiling event was responsible for >90% of the metal accumulation in the deposit and took place across an entire interlayered structural zone between hornfels and marble. The two hydrothermal stages are interpreted to be separated by a period of brecciation which mainly affect the skarn and Granite Porphyries, and contain clasts of garnet and wollastonite skarns and marble cemented by quartz (Yao et al., 2018). The principal mineralised veins in each of the ore types comprise B and D veins in the porphyry ore; type c) and d) in the hornfels and types II, III and IV in the skarns, all of which are correlated with the late hydrothermal stage. The three veining stages in the skarn mineralisation correspond to the late sulphide stage of retrograde skarn development. Information in this paragraph is summarised from Yao et al., 2018).
The occurrence of each of the different metal associations, is as follows:
• Copper mineralisation occurs in the porphyry, 'hornfels' and skarn orebodies. Porphyry and 'hornfels' mineralisation occurs above elevation 4700 m, and is dominated by disseminated and veinlet chalcopyrite. In contrast, in the skarn altered zone, Cu occurs as massive, disseminated and vein chalcopyrite with bornite and minor vein tetrahedrite, and is distributed throughout the middle to upper section of the #1 skarn orebody, and is dominant throughout the #2 skarn orebody.
Three main stages of Cu mineralisation have been differentiated at Jiama: i). the major stage, which produced disseminated and veinlet chalcopyrite in the upper section of the porphyry and 'hornfels' zones, and disseminated, vein and massive copper mineralisation in the skarn; ii). an intermediate stage, which deposited stockwork Cu and Mo mineralisation in the 'hornfels' and porphyry; and iii). late-stage, near-vertical vein chalcopyrite in the 'hornfels', 'porphyry' and skarn zones.
• Molybdenum mineralisation which occurs as veined molybdenite in the porphyry and 'hornfels' ores, whilst skarns host disseminated molybdenite, all of which are below an elevation of 4700 m. Two stages of Mo mineralisation are recognised: i). disseminated and stockwork veining in the 'hornfels', porphyry and skarn, and occurred later than the first stage of Cu mineralisation; and ii). vein Cu and Mo mineralisation, which was coeval with the second stage of Cu mineralisation.
• Lead-zinc mineralisation, which is largely restricted to the skarn zone, and is dominantly composed of banded and disseminated galena-sphalerite. It occurs above 4800 m elevation, throughout the distal zone of the #1 skarn, and small amounts in the upper parts of the #2 skarn. Galena, sphalerite and chalcopyrite exhibit a paragenetic sequence discernible in solid solution textures between sphalerite and chalcopyrite. As a consequence, Pb-Zn mineralisation is regarded to be deposited during the main stage of Cu mineralisation event.
• Gold and silver mineralisation generally occur within chalcopyrite, bornite and other Cu minerals, and are a by-products retrievable from Cu concentrates. Au appears to be of economic significance in the distal zone of the #1 skarn, where it occurs in three modes: i). In the NW part of the deposit, the skarn is dominated by hedenbergite, has been intensely silicified, and has a sulphide association of pyrite+native gold; ii). In the Quartz-Diorite Porphyry, also in the NW part of the deposit, gold is found in veins with a mineral association is quartz-calcite-pyrite-sericite-native gold; and iii). In distal skarn, in the SE part of the deposit, gold mineralisation is associated with intense actinolite, chlorite and silica alteration, with associated massive pyrrhotite, chalcopyrite, pyrite and native gold. The latter is not spatially connected to either the #1 or #2 skarn bodies. This distal Au mineralisation post-dates the Cu-Mo mineralization throughout the deposit, and is the product of late-stage hydrothermal alteration (Zheng et al., 2012; Zhong et al., 2011).
Mineralisation is zoned, related to different migration pathways and precipitation temperatures, to produce upper Cu and lower Mo zones in the 'hornfels' and porphyry orebodies, while the skarn orebodies show an outwards gradation from the porphyry contact of: Mo → Cu-Mo → Cu ±Mo → Cu ±Pb-Zn → Pb-Zn and finally → Au mineralisation.
The information in this summary is drawn from Zheng et al., 2016 and Yao et al., 2018. Some information has also been drawn from Cameron, A.R. and Guo, B., 2014 - Jiama Phase 2 Extension Project, Mineral Resources and Reserves; An NI 43-101 Technical Report prepared by Mining One Consultants for China Gold International, 250p.
Lakang'e Mo-Cu
Lakang'e is located ~20 km SW of Qulong. The country rocks in the Lakang'e district include the Middle to Lower Jurassic Yeba Formation, the Upper Jurassic Duodigou Formation and Quaternary rocks. The first segment of the Yeba Formation within the central part of the district strikes almost east-west and dips steeply to the north. It is dominated by schistose andesite, intermediate to basic volcanic breccia, sericite-quartz schist, chlorite-quartz schist and tuff units. The second segment crops out in the northern part of the district, and is in conformable contact with the first segment, also striking nearly east-west. It is dominated by volcanic breccia and crystal-rich tuff units that are intercalated with metamorphosed fine-grained sandstone and slate. The Duodigou Formation is exposed in the southern section of the district. It is in faulted contact with the Yeba Formation, and is dominated by fine crystalline limestone, bioclastic limestone and limestone-marble units interbedded with sandstone. Quaternary rocks generally occur along river beds and terraces and are dominated by Holocene pluvial to alluvial gravels and sandy gravels which also contain silty and sandy loam.
The older successions are cut by Miocene intermediate to acidic intrusive rocks related to the Lakang'e deposit, exposed in the central and northwestern sections of the district, occupying ~20% of the area. This multiphase intrusive complex can be divided into two different intrusive phases, on the basis of petrographic and cross-cutting relationships. The Granodiorite Porphyry is the main ore-bearing porphyry, whilst the volumetrically minor Quartz Diorite porphyry was post-mineral, as follows:
• Granodiorite Porphyry - which is the ore-bearing intrusion, the largest at Lakang'e, with an outcrop area of 3 km2. It trends NW, is ~3 km in length and has a width varying from 0.2 to 1.0 km. Phenocrysts range from 25 to 40% of the total volume and include plagioclase (0.2 to 1 cm), quartz (0.2 to 0.5 cm), biotite (0.1 to 0.5 cm) and minor K feldspar (0.2 to 0.5 cm). The groundmass is quartz-feldspathic and consists of fine-grained quartz and plagioclase. Accessory minerals include apatite, zircon, rutile, titanite and magnetite. Strong contact metamorphism in the surrounding Jurassic Yeba Formation accompanied its intrusion. Some microgranular mafic enclaves can be found in the main mass, mainly composed of plagioclase and biotite. Local volumetrically minor mineralised breccias are found at shallow levels and are related to well developed fissure structures. The Granodiorite Porphyry is cut by quartz-sulphide veins and post-mineralization Quartz Diorite porphyry dykes, and has undergone moderate to strong potassic, phyllic and chlorite alteration as detailed below.
• Quartz Diorite porphyry - which is post-mineral, occurring as dykes and the youngest intrusions associated with the Lakang'e deposit. It conytains no mineralisation. Individual dykes are generally 2 to 5 m wide. Phenocrysts of plagioclase, hornblende and minor quartz range from 0.05 to 0.5 cm in size, and account for 20 to 25% of the rock volume. Accessory minerals include apatite, zircon and titanite. Although regarded as post-mineral, the Quartz Diorite porphyry has undergone strong phyllic alteration. Plagioclase and hornblende grains have metasomatic pseudotextures, with a large number of fine-grained cubic pyrite grains.
Mineralisation and alteration is spatially related to the Granodiorite Porphyry. Hydrothermal alteration assemblages recognised at Lakang'e are mainly potassic and phyllic styles, with lesser weak to moderate argillic, chlorite, carbonate, skarn and hornfels alteration. High-grade Mo mineralization mainly occurs in areas with potassic and phyllic alteration, whilst high-grade Cu mineralisation is mainly found in areas with argillic and phyllic alteration. The potassic alteration is characterized by the formation of biotite and quartz, which occur mainly within the Granodiorite Porphyry. The phyllic alteration, as the product of feldspar or hornblende replacement, is represented by fine-grained sericite and pyrite. The chlorite alteration is characterised by the replacement of biotite and plagioclase by chlorite or disseminated chlorite. The carbonation alteration is represented by calcite veins or patchy calcite..
Whilst the deposit is understood to be significant, no resource figures have been encountered to December, 2021.
The information in this summary is drawn from Tang et al., 2021.
Bangpu Mo-Cu
The Bangpu porphyry Mo-Cu deposit is located in the eastern section of the northern Gangdese Porphyry Copper Belt in a zone of well developed fracturing and ductile shearing (Wen et al., 2009). The country rock in the area includes some Quaternary sedimentary rocks, but mainly tuffs of the Paleocene Dianzhong Formation, as well as volcanic breccias, tuffs, marbles and limestones of the Lower Permian Luobadui Formation.
The mineralisation related intrusive rocks comprise adamellite/quartz monzonite and diorite porphyries (Zhou et al., 2010b). The former contains phenocrysts quartz, plagioclase, K feldspar and minor biotite set in a matrix is quartz and feldspar. The phenocrysts in the diorite are predominantly of feldspar and amphibole within a matrix almost entirely composed of feldspar.
The deposit has undergone widespread silicification and sericitisation characteristically comprising anhedral granular quartz and flakey sericite, overprinting a potassic assemblage of K feldspar, biotite with lesser quartz and albite, passing out into widespread propylitisation that produced chlorite and carbonatisation. The principal metallic minerals are pyrite, chalcopyrite, sphalerite, molybdenite, galena, pyrrhotite, accompanied by tennantite, enargite and bismuthinite.
The alteration assemblage differs between the ore-bearing adamellites and diorites. The diorite is characterised by multi-stage potassic alteration, with sericite, propylitic minerals and carbonates, with most of the primary minerals having been replaced by secondary biotite, sericite, chlorite and calcite, along with pyrite, chalcopyrite and bornite. Both the alteration and mineralisation is very strong. In contrast the quartz monzonite porphyry is only locally altered, and has only undergone potassic alteration and sericitisation. The 15.30 Ma diorite porphyry is interpreted to have been derived from the asthenosphere, and has been contaminated by the crust (Hou et al., 2009). The 15 to 14 Ma quartz monzonite porphyry is interpreted to have strong affinities with adakite, and formed later than the diorite porphyrite from a source region of thickened lower crust below South Tibet. The main ore-forming period is ca. 16 to 14 Ma at Bangpu (Wang et al., 2012).
The Bangpu deposit is quoted as having reserves ~0.80 Mt of Mo at an average grade of 0.089% Mo in ~900 Mt of ore (Zhao et al., 2015), and of ~0.2 Mt of Cu at grades of 0.32% Cu in ~ 62.5 Mt of ore (Ma et al. 2016).
The information in this summary is drawn from Wang et al., 2012.
Zhunuo Cu-Mo-Au
The Zhunuo porphyry copper deposit is located within the Gangdese belt of southern Tibet. Country rocks include 51.6 ±1.0 Ma Eocene rhyolite and 49.1 ±0.6 Ma quartz porphyry. These are intruded by mineralised Miocene 14.7 ±0.3 Ma monzogranite and 14.5 ±0.2 Ma monzogranite porphyry which commonly host 14.9 ±0.3 Ma microgranular mafic enclaves. The Miocene intrusions are cross-cut by late-mineral 14.2 ±0.2 Ma diorite porphyry, post-mineral 12.2 ±0.1 Ma lamprophyre dykes and 12.0 ±0.2 Ma granite porphyry. Quartz-molybdenite veins have been dated at 14.8 ±0.1 Ma and 14.4 ±0.1 Ma (Re-Os molybdenite model ages) whilst quartz-muscovite-llite-pyrite-molybdenite veins returned ages of 14.2 ±0.1 Ma and 13.5 ±0.1 Ma (Re-Os molybdenite model ages).
Mineralisation at Zhunuo is characterized by early potassic alteration with associated quartz A veins centered on the monzogranite porphyry and adjacent monzogranite. These are surrounded by distal propylitic alteration within the rhyolite country rocks, and late-stage phyllic alteration with associated quartz B veins, followed by D veins that overprinted the quartz porphyry and potassic-altered rocks. Copper mineralisation is predominantly within the potassic alteration zone that was overprinted by phyllic alteration.
The deposit has resources of 2.37 Mt of contained copper @ 0.57% Cu, which would equate to an ore tonnage of ~400 Mt. The deposit also contains economically extractable gold.
The information in this Zhunuo summary is drawn from Sun et al., 2021.
Beimulang Cu-Mo-Ag
The Beimulang deposit, which is located within Xigaze City, lies immediately to the SW, and within a few hundred metres of the Zhunuo porphyry copper deposit. The exposed country rocks surrounding the Zhunuo-Beimulang deposit pair include the tuffs and rhyolites of the Eocene Pana Formation, and the Eocene tuffaceous sedimentary rocks of the Nianbo Formation.
The intrusive suite at Beimulang is very similar to that at Zhunuo, predominantly comprising Eocene quartz porphyry and Miocene monzogranite porphyry as well as monzogranite, granite porphyry and diorite dykes. At Beimulang, the Eocene quartz porphyry is peripheral to mineralisation and has predominantly been subjected to sericite alteration, as manifested by the replacement of plagioclase, and the development of disseminated pyrite aggregates and quartz-pyrite veins. It has also been intruded by Miocene monzogranite, followed by syn-mineral Miocene monzogranite porphyry. Whilst the monzogranite porphyry remains concealed at Beimulang, it is exposed at Zhunuo. Subsequently, Miocene granite porphyry was intruded as stocks or dyke, cutting both the Miocene monzogranite and monzogranite porphyry.
Two main ore bodies have been outlined at Beimulang, namely:
• Orebody I to the north, which occurs as an irregular tabular body dipping to the SW, being wider in the north and narrowing towards the south. The long axis of the deposit has a length of 1778 m and trends NE, whilst the short axis trends NW and ranges from 765 to 1655 m. The thickness varies between 15 and 50 m. Copper grades range from 0.2% to 0.7%, with an average of 0.38%, accompanied by a substantial silver and a minor molybdenum.
• Orebody II, which is ~2 km to the SE, and is much smaller, has Cu grades of generally between 0.2 and 0.4%, and associated high grade silver.
Hydrothermal alteration at Beimulang includes potassic, phyllic and propylitic assemblages. Potassic alteration includes both K feldspar and biotite replacement as as swell as K feldspar veinlets. The K feldspar alteration is characterised by pervasive or selective replacement of magmatic feldspar in monzogranite and monzogranite porphyry, imparting a strong pink colouration. Biotite alteration is characterised by hydrothermal biotite selectively replacing primary mafic minerals. Phyllic alteration occurs in monzogranite, monzogranite porphyry and quartz porphyry, and is characterised by pervasive sericite and quartz replacing the original textures or earlier alteration assemblages. Phyllic alteration is also manifested as vein halos. Propylitic alteration is found as pervasive disseminations or as veins of chlorite, epidote and calcite, replacing original textures and overprinting earlier phyllic alteration. Propylitic alteration mainly affects the monzogranite and quartz porphyry.
The deposit is reported to contain 1.3 Mt of Cu at a grade of 0.38% Cu, which would equate to ~340 Mt of ore.
The information in this Beimulang summary is drawn from Du et al., 2021, which is cited below.
The most recent source geological information used to prepare this decription was dated: 2023.
Record last updated: 26/7/2024
This description is a summary from published sources, the chief of which are listed below. © Copyright Porter GeoConsultancy Pty Ltd. Unauthorised copying, reproduction, storage or dissemination prohibited.
|
|
Beaudoin, G., Hebert, R., Wang, C.S. and Tang, J., 2005 - Epithermal Au-Ag-Cu, porphyry Cu-(Au-Mo) and Cu-Au-Ag-Zn-Pb skarn deposits of the Gangdese Arc, Tibet: in Mao, J. and Bierlein, F.P., (Eds.), 2005 Mineral Deposit Research: Meeting the Global Challenge , Eighth Biennial SGA Meeting, Beijing, China, 18-21 August 2005, Proceedings, Chapter 11-1, pp. 1219-1222.
|
Cao, H.‐W., Zhang, Y.‐H., Santosh, M., Li, G.‐M., Hollis, S.P., Zhang, L.‐K., Pei, Q.‐M., Tang, L. and Duan, Z.‐M., 2018 - Petrogenesis and metallogenic implications of Cretaceous magmatism in Central Lhasa, Tibetan Plateau: A case study from the Lunggar Fe skarn deposit and perspective review: in Geological Journal v.53, pp. 1-24.
|
Chen, J.-L., Xu, J.-F., Zhao, W.-X., Dong, Y.-H., Wang, B.-D. and Kang, Z.-Q., 2011 - Geochemical variations in Miocene adakitic rocks from the western and eastern Lhasa terrane: Implications for lower crustal flow beneath the Southern Tibetan Plateau: in Lithos v.125, pp. 928-939.
|
Chen, X., Richards, J.P., Liang, H., Zou, Y., Zhang, J., Huang, W., Ren, L. and Wang, F., 2019 - Contrasting arc magma fertilities in the Gangdese belt, Southern Tibet: Evidence from geochemical variations of Jurassic volcanic rocks: in Lithos v. 324-325, pp. 789-802.
|
Deng, J. and Wang, Q., 2016 - Gold mineralization in China: Metallogenic provinces, deposit types and tectonic framework: in Gondwana Research v.36, pp. 219-274.
|
Du, Z., Cheng, Z., Yu, X., Liu, X. and Zhao, Y., 2023 - Geochronology and petrogeochemistry of Miocene porphyries from the Beimulang deposit, western Gangdese copper belt: in Ore Geology Reviews v.162, 27p. doi.org/10.1016/j.oregeorev.2023.105682.
|
Ge, Y., Li, Y., Wang, X., Qian, X., Zhang, J., Zhou, A. and Liu-Zeng, J., 2018 - Oligocene-Miocene burial and exhumation of the southernmost Gangdese mountains from sedimentary and thermochronological evidence: in Tectonophysics v.723, pp. 68-80.
|
Guan, Q., Zhu, D.-C., Zhao, Z.-D., Dong, G.-C., Zhang, L.-L., Li, X.-W., Liu, M., Mo, X.-X., Liu, Y.-S. and Yuan, H.-L., 2012 - Crustal thickening prior to 38 Ma in southern Tibet: Evidence from lower crust-derived adakitic magmatism in the Gangdese Batholith: in Gondwana Research v.21, pp. 88-99.
|
Hou, Z. and Cook, N., 2009 - Metallogenesis of the Tibetan collisional orogen: A review and introduction to the special issue: in Ore Geology Reviews v.36, pp. 2-24.
|
Hou, Z., Yang, Z., Qu, X., Meng, X., Li, Z., Beaudion, G., Rui, Z., Gao, Y. and Zaw, K., 2009 - The Miocene Gangdese porphyry copper belt generated during post-collisional extension in the Tibetan orogen: in Ore Geology Reviews v.36, pp. 25-51.
|
Hou, Z., Zhang, H., Pan, X. and Yang, Z., 2011 - Porphyry Cu (-Mo-Au) deposits related to melting of thickened mafic lower crust: Examples from the eastern Tethyan metallogenic domain: in Ore Geology Reviews v.39, pp. 21-45.
|
Hu, Y.-B., Liu, J.-Q., Ling, M.-X., Ding, W., Liu, Y., Zartman, R.E., Ma, X.-F., Liu, D.Y., Zhang, C.-C., Sun, S.-J., Zhang, L.-P., Wu, K, and Sun, W.-D., 2015 - The formation of Qulong adakites and their relationship with porphyry copper deposit: Geochemical constraints: in Lithos v.220-223, pp. 60-80.
|
Jiang, J.-S., Zheng, Y.-Y., Gao, S.-B., Zhang, Y.-C., Huang, J., Liu, J., Wu, S., Xu, J. and Huang, L.-L., 2018 - The newly-discovered Late Cretaceous igneous rocks in the Nuocang district: Products of ancient crust melting trigged by Neo-Tethyan slab rollback in the western Gangdese: in Lithos v. 308-309, pp. 294-315.
|
Lang, X., Tang, J., Li, Z., Huang, Y., Ding, F., Yang, H., Xie, F., Zhang, L., Wang, Q. and Zhou, Y., 2014 - U-Pb and Re-Os geochronological evidence for the Jurassic porphyry metallogenic event of the Xiongcun district in the Gangdese porphyry copper belt, southern Tibet, PRC: in J. of Asian Earth Sciences v.79, pp. 608-622.
|
Li, Y., McCoy-West, A.J., Zhang, S., Selby, D., Burton, K.W. and Horan, K., 2019 - Controlling Mechanisms for Molybdenum Isotope Fractionation in Porphyry Deposits: The Qulong Example: in Econ. Geol. v.114, pp. 981-992.
|
Li, Y., Selby, D., Condon, D. and Tapster, S., 2017 - Cyclic Magmatic-Hydrothermal Evolution in Porphyry Systems: High-Precision U-Pb and Re-Os Geochronology Constraints on the Tibetan Qulong Porphyry Cu-Mo Deposit: in Econ. Geol. v.112, pp. 1419-1440.
|
Liu, D., Zhao , Z., DePaolo, D.J., Zhu, D.-C., Meng, F.-Y., Shi, Q. and Wang, Q., 2017 - Potassic volcanic rocks and adakitic intrusions in southern Tibet: Insights into mantle-crust interaction and mass transfer from Indian plate: in Lithos v.268-271, pp. 48-64.
|
Ma, L., Wang, Q., Wyman, D.A., Jiang, Z.-Q., Yang, J.-H., Li, Q.-L., Gou, G.N. and Guo, H.-F., 2013 - Late Cretaceous crustal growth in the Gangdese area, southern Tibet: Petrological and Sr-Nd-Hf-O isotopic evidence from Zhengga diorite-gabbro: in Chemical Geology, v.349-350, pp. 54-70.
|
Qu, X., Hou, Z. and Li, Y., 2004 - Melt components derived from a subducted slab in late orogenic ore-bearing porphyries in the Gangdese copper belt, southern Tibetan plateau: in Lithos v.74 pp. 131-148.
|
Qu, X., Hou, Z., Zaw, K., Mo, X., Xu, W. and Xin, H., 2009 - A large-scale copper ore-forming event accompanying rapid uplift of the southern Tibetan Plateau: Evidence from zircon SHRIMP U-Pb dating and LA ICP-MS analysis: in Ore Geology Reviews v.36, pp. 52-64.
|
Sun, X., Leng, C.-B., Hollings, P., Song, Q.-S., Li, R.-X. and Wan, X.Q., 2021 - New 40Ar/39Ar and (U-Th)/He dating for the Zhunuo porphyry Cu deposit, Gangdese belt, southern Tibet: implications for pulsed magmatic-hydrothermal processes and ore exhumation and preservation: in Mineralium Deposita v.56, pp. 917-934.
|
Sun, X., Li, R., Si, X.,Xiao, K. and Dung, J., 2024 - Timing and Mechanism of Ore Precipitation in Porphyry Cu Systems: Insight from LA-ICP-MS Analysis of Fluid Inclusions and In Situ Oxygen Isotope Analysis of Hydrothermal Quartz at Zhunuo Porphyry Cu Deposit, China: in Econ. Geol. v.119, pp. 593-616. doi: 10.5382/econgeo.5064.
|
Sun, X., Zheng, Y., Xu, J., Huang, L., Guo, F. and Gao, S., 2017 - Metallogenesis and ore controls of Cenozoic porphyry Mo deposits in the Gangdese belt of southern Tibet: in Ore Geology Reviews v.81, pp. 996-1014.
|
Tafti, R., Lang, J.R., Mortensen, J.K., Oliver, J.L. and Rebagliati, C.M, 2014 - Geology and Geochronology of the Xietongmen (Xiongcun) Cu-Au Porphyry District, Southern Tibet, China: in Econ. Geol. v.109, pp. 1967-2001.
|
Tang, P., Tang, J., Wang, Y., Lin, B., Leng, Q., Zhang, Q., He, L., Zhang, Z., Sun, M., Wu, C., Qi, J., Li, Y. and Dai, S., 2021 - Genesis of the Lakange porphyry Mo (Cu) deposit, Tibet: Constraints from geochemistry, geochronology, Sr-Nd-Pb-Hf isotopes, zircon and apatite: in Lithos v.380-381, 16p. doi.org/10.1016/j.lithos.2020.105834.
|
Tang, P., Tang, J.-X., Lin, B., Wang, L.-Q., Zheng, W.-B.,Leng, Q.-F., Gao, X., Zhang, Z.-B. and Tang, X.-Q., 2019 - Mineral chemistry of magmatic and hydrothermal biotites from the Bangpu porphyry Mo (Cu) deposit, Tibet: in Ore Geology Reviews v.115, 16p. doi.org/10.1016/j.oregeorev.2019.103122.
|
Wang, C., Bagas, L., Lu, Y., Santosh, M., Dua, B. and McCuaig, C., 2016 - Terrane boundary and spatio-temporal distribution of ore deposits in the Sanjiang Tethyan Orogen: Insights from zircon Hf-isotopic mapping: in Earth Science Reviews v.156, pp. 39-65.
|
Wang, Q., Zhu, D.-C., Liu, A.-L., Cawood, P.A., Liu, S.-A., Xia, Y., Chen, Y., Wang, H., Zhang, L.-L. and Zhao, Z.-D., 2018 - Survival of the Lhasa Terrane during its collision with Asia due to crust-mantle coupling revealed by ca. 114 Ma intrusive rocks in western Tibet: in Lithos v.304-307, pp. 200-210.
|
Wang, R., Richards, J.P., Hou, Z. and Yang, Z., 2014 - Extent of underthrusting of the Indian plate beneath Tibet controlled the distribution of Miocene porphyry Cu-Mo ± Au deposits: in Mineralium Deposita v.49, pp. 165-173.
|
Wang, R., Richards, J.P., Zhou, L.-M., Hou, Z.-Q., Stern, R.A., Creaser, R.A. and Zhu, J.-J., 2015 - The role of Indian and Tibetan lithosphere in spatial distribution ofCenozoic magmatism and porphyry Cu-Mo deposits in the Gangdesebelt, southern Tibet: in Earth Science Reviews v.150, pp. 68-94.
|
Wang, R., Weinberg, R.F., Collins, W.J., Richards, J.P. and Zhu, D.-C., 2018 - Origin of postcollisional magmas and formation of porphyry Cu deposits in southern Tibet: in Earth Science Reviews v.181, pp. 122-143.
|
Wang, X., Lang, X., Turlin, F., Deng, Y., Xie, F., He, Q. and Moritz, R., 2024 - Copper behavior in arc-back-arc systems: Insights into the porphyry Cu metallogeny of the Gangdese belt, southern Tibet: in Mineralium Deposita v.59, pp. 133-154.
|
Wang, Y.-F., Zeng, L., Gao, J., Zhao, L., Gao, L.-E. and Shang, Z., 2019 - Along-arc variations in isotope and trace element compositions of Paleogene gabbroic rocks in the Gangdese batholith, southern Tibet: in Lithos v.324-325, pp. 877-892.
|
Wang, Z., Liu, Y., Liu, H., Guo, L., Zhang, J. and Xu, K., 2012 - Geochronology and geochemistry of the Bangpu Mo-Cu porphyry ore deposit, Tibet: in Ore Geology Reviews v.46, pp. 95-105.
|
Wu, S., Zheng, Y., Geng, R., Jin, L., Bao, B., Tan, M. and Guo, F., 2017 - Geology, fluid inclusion and isotope constraints on ore genesis of the post-collisional Dabu porphyry Cu-Mo deposit, southern Tibet: in Ore Geology Reviews v.89, pp. 421-440
|
Wu, S., Zheng, Y., Wang, D., Chang, H. and Tan, M., 2017 - Variation of copper isotopes in chalcopyrite from Dabu porphyry Cu-Mo deposit in Tibet and implications for mineral exploration: in Ore Geology Reviews v.90, pp. 14-24.
|
Xiao, B., Qin, K., Li, G., Li., J., Xia, D., Chen, L. and Zhao, J., 2012 - Highly Oxidized Magma and Fluid Evolution of Miocene Qulong Giant Porphyry Cu-Mo Deposit, Southern Tibet, China: in Resource Geology v.62, pp. 4-18.
|
Xie, F., Tang, J., Chen, Y. and Lang, X., 2018 - Apatite and zircon geochemistry of Jurassic porphyries in the Xiongcun district, southern Gangdese porphyry copper belt: Implications for petrogenesis and mineralization: in Ore Geology Reviews v.96, pp. 98-114.
|
Xu, J., Zheng, Y., Sun, X. and Shen, Y., 2016 - Alteration and mineralization at the Zhibula Cu skarn deposit, Gangdese belt, Tibet: in Ore Geology Reviews v.75, pp. 304-326.
|
Xu, J., Zheng, Y.-Y., Sun, X. and Shen, Y.-H., 2016 - Geochronology and petrogenesis of Miocene granitic intrusions related to the Zhibula Cu skarn deposit in the Gangdese belt, southern Tibet: in J. of Asian Earth Sciences v.120, pp. 100-116.
|
Yang, Y.-F. and Wang, P., 2017 - Geology, geochemistry and tectonic settings of molybdenum deposits in Southwest China: A review: in Ore Geology Reviews v.81, pp. 965-995.
|
Yang, Z., Hou, Z., Chang, Z., Li, Q., Liu, Y. and Qu, H., 2016 - Cospatial Eocene and Miocene granitoids from the Jiru Cu deposit in Tibet: Petrogenesis and implications for the formation of collisional and postcollisional porphyry Cu systems in continental collision zones: in Lithos v.245, pp. 243-257.
|
Yang, Z., Hou, Z., White, N.C., Chang, Z., Li, Z. and Song, Y., 2009 - Geology of the post-collisional porphyry copper-molybdenum deposit at Qulong, Tibet: in Ore Geology Reviews v.36, pp. 133-159.
|
Yang, Z.-M., Lu, Y.-J., Hou, Z.-Q. and Chang, Z.-S., 2015 - High-Mg Diorite from Qulong in Southern Tibet: Implications for the Genesis of Adakite-like Intrusions and Associated Porphyry Cu Deposits in Collisional Orogens: in J. of Petrology v.56, pp. 227-254.
|
Yao, X., Liu, J., Tang, J., Zheng, W. and Zhang, Z., 2018 - Fluid Evolution and Ore-forming Processes of the Jiama Cu Deposit, Tibet: Evidence from Fluid Inclusions: in Acta Geologica Sinica v.92, pp. 127-143.
|
Yu, K., Li, G., Zhao, J., Evans, N.J., Li, J., Jiang, G., Zou, X., Qin, K. and Hu, G., 2022 - Biotite composition as a tracer of fluid evolution and mineralization center: a case study at the Qulong porphyry Cu-Mo deposit, Tibet: in Mineralium Deposita v.57, pp. 1047-1069.
|
Zeng, Y.-C., Chen, J.-L., Xu, J.-F., Lei, M. and Xiong, Q.-W., 2017 - Origin of Miocene Cu-bearing porphyries in the Zhunuo region of the southern Lhasa subterrane: Constraints from geochronology and geochemistry: in Gondwana Research v.41, pp. 51-64.
|
Zhang, K.-J., Zhang, Y.-X., Tang, X.-C. and Xia B., 2012 - Late Mesozoic tectonic evolution and growth of the Tibetan plateau prior to the Indo-Asian collision: in Earth Science Reviews v.114, pp. 236-249.
|
Zhang, Z., Yao, X.-F., Tang, J.-X., Li, Z.-J., Wang, L.-Q., Yang, Y., Duan, J.,-L., Song, J.-L. and Lin, X., 2015 - Lithogeochemical, Re-Os and U-Pb Geochronological, Hf-Lu and S-Pb Isotope Data of the Ga erqiong-Galale Cu-Au Ore-Concentrated Area: Evidence for the Late Cretaceous Magmatism and Metallogenic Event in the Bangong-Nujiang Suture Zone, NW Tibet, China: in Resource Geology v.65, pp. 76-102.
|
Zhao, J., Qin, K., Li, G., Cao, M., Evans, N.J., McInnes, B.I.A., Li, J., Xiao, B. and Chen, L., 2015 - The exhumation history of collision-related mineralizing systems in Tibet: Insights from thermal studies of the Sharang and Yaguila deposits, central Lhasa: in Ore Geology Reviews v. 65, pp. 1043-1061.
|
Zhao, J., Qin, K., Li, G., Li, J., Xiao, B. and Chen, L., Yang, Y., Li, C. and Liu, Y., 2014 - Collision-related genesis of the Sharang porphyry molybdenum deposit, Tibet: Evidence from zircon U-Pb ages, Re-Os ages and Lu-Hf isotopes: in Ore Geology Reviews v.56, pp. 312-326.
|
Zhao, J., Qin, K., Li, G., Li., J., Xiao, B. and Chen, L., 2012 - Geochemistry and Petrogenesis of Granitoids at Sharang Eocene Porphyry Mo Deposit in the Main-Stage of India-Asia Continental Collision, Northern Gangdese, Tibet: in Resource Geology v.62, pp. 84-98.
|
Zheng, S.-J., Zhong, H., Bai, Z.-J., Zhang, Z.-K. and Wu, C.-Q., 2021 - High-sulfidation veins in the Jiama porphyry system, South Tibet: in Mineralium Deposita v.56, pp. 205-214
|
Zheng, W., Tang, J., Zhong, K., Ying, L., Leng, Q., Ding, S. and Lin, B., 2016 - Geology of the Jiama porphyry copper-polymetallic system, Lhasa Region, China: in Ore Geology Reviews v.74, pp. 151-169.
|
Zheng, Y., Chen, X., Palmer, M.R., Zhao, K., Hernandez-Uribe, D., Gao, S. and Wu, S., 2024 - Magma mixing and magmatic-to-hydrothermal fluid evolution revealed by chemical and boron isotopic signatures in tourmaline from the Zhunuo-Beimulang porphyry Cu-Mo deposits: in Mineralium Deposita v.59, pp. 1133-1153.
|
Zheng, Y., Sun, X., Gao, S., Wu, S., Xu, J,m Jiang, J., Chen, X., Zhao, Z. and Liu, Y., 2015 - Metallogenesis and the minerogenetic series in the Gangdese polymetallic copper belt: in J. of Asian Earth Sciences v.103, pp. 23-39.
|
Zheng, Y.Y., Sun, X., Gao, S.B., Zhao, Z.D., Zhang, G.Y., Wu, S., You, Z.M. and Li, J.D., 2014 - Multiple mineralization events at the Jiru porphyry copper deposit, southern Tibet: implications for Eocene and Miocene magma sources and resource potential: in J. of Asian Earth Sciences v.79, pp. 842-857.
|
Zhou, L.-M., Wang, R., Hou, Z.-Q., Li, C., Zhao, H., Li, X.-W. and Qu, W.-J., 2018 - Hot Paleocene-Eocene Gangdese arc: Growth of continental crust in southern Tibet: in Gondwana Research v.62, pp. 178-197.
|
Zhu, D.-C., Zhao, Z.-D., Niu, Y., Mo, X.-X., Chung, S.-L. and Hou, Z.-Q., 2011 - The Lhasa Terrane: Record of a microcontinent and its histories of drift and growth: in Earth and Planetary Science Letters v.301, pp. 241-255.
|
Zou, Y., Chen, X., Huang, W., Zhang, J., Liang, H., Xu, J. and Chen, L., 2017 - Identification of an Early-Middle Jurassic oxidized magmatic belt, south Gangdese, Tibet, and geological implications: in Science Bulletin v.62, pp. 888-898
|
Porter GeoConsultancy Pty Ltd (PorterGeo) provides access to this database at no charge. It is largely based on scientific papers and reports in the public domain, and was current when the sources consulted were published. While PorterGeo endeavour to ensure the information was accurate at the time of compilation and subsequent updating, PorterGeo, its employees and servants: i). do not warrant, or make any representation regarding the use, or results of the use of the information contained herein as to its correctness, accuracy, currency, or otherwise; and ii). expressly disclaim all liability or responsibility to any person using the information or conclusions contained herein.
|
Top | Search Again | PGC Home | Terms & Conditions
|
|