Bangong Co-Nujiang Mineral Belt - Duolong Ore Cluster - Tiegelongnan, Naruo, Bolong, Duobuza, Dibaonamugang, Nating |
|
Xizang - Tibet, China |
Main commodities:
Cu Au W Fe Ni Cr
|
|
 |
|
 |
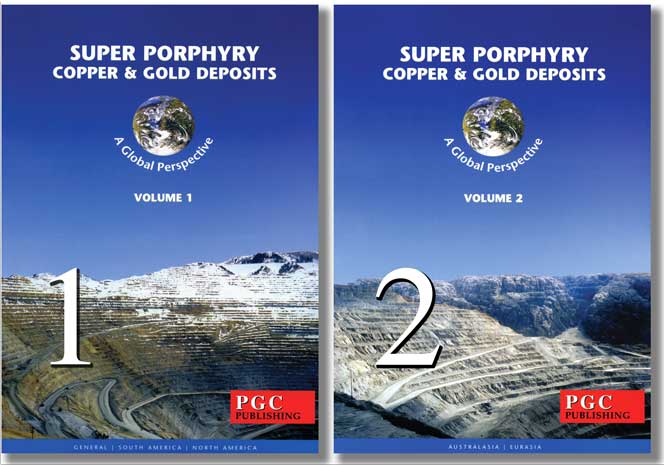 |
Super Porphyry Cu and Au

|
IOCG Deposits - 70 papers
|
All papers now Open Access.
Available as Full Text for direct download or on request. |
|
 |
The Bangong Co-Nujiang Mineral Belt straddles the Bangong-Nujiang Suture Zone that separates the South Qiangtang Sub-terrane and Lhasa Terrane in central Tibet (see image below) over an east-west interval of ~1000 km and width of up to ~200 km. The belt contains
ophiolitic hosted nickel-chromite,
porphyry copper,
skarn copper, skarn iron,
orogenic gold and
quartz-vein tungsten mineralisation.
In its western section, the belt also incorporates the more restricted, NE-SW trending, 50 x 22 km Duolong Ore Cluster that incorporates a string of Upper Cretaceous porphyry and epithermal Cu-(Au) mineralised systems. These are the most significant deposits within the mineral belt and include the large Tiegelongnan, Bolong, Naruo, Duobuza and Nating-Dibaonamugang deposits.
REGIONAL SETTING
The Bangong Co-Nujiang Suture Zone and bordering Lhasa and composite Qiangtang terranes, are part of the East Tethyan Orogenic Belt of central to southeast Asia. These are, in turn, within the eastern segment of the greater Tethyan Orogenic Belt that extends from Indochina in the SE to the Atlas Mountains of Morocco to the west. Other significant adjoining and nearby mineral belts that together constitute the East Tethyan Orogenic Belt include the
Gangdese,
Yulong,
Ailaoshan-Red River,
Yidun/Zhongdian and the
North Himalayan Mineral Belt which lies to the south of the eastern half of the Gangdese Belt.
To the NW, the Songpan Ganzi Complex is obliquely truncated and offset by the long-lived, multiple strand, continental scale, ENE-WSW trending, sinistral Altyn Tagh Fault. To the west again, the Altyn Tagh Fault zone curves to the west and then NW, where it merges with the dextral, NW-SE trending Karakoram Fault. This latter fault zone cuts the attenuated western extremities of the Songpan Ganzi, Qiangtang and Lhasa terranes, and displaces them to become the Pamirs to the west.
See the Regional Setting section of the Ailaoshan-Red River Belt record for the broad framework into which the suture is set and how the mineral belts listed above are disposed.
The generally east-west to WNW-ESE trending Bangong Co-Nujiang Mineral Belt incorporates deposits in magmatic arcs of the southern margin of the South Qiangtang Sub-terrane in the north, the Bangong Co-Nujiang Suture Zone in the centre, and the North and Central Lhasa sub-terranes to the south.
For an outline of the geology of the North and Central Lhasa sub-terranes, see the Regional Setting section of the Gangdese Belt record.
Where mapped in detail the South Qiangtang Sub-terrane stratigraphy is made up of (after Zhao, 2015 and others):
• Qiangtang basement comprising greenschist-facies pre-Ordovician strata, dominantly quartzite and phyllite, with pervasive folding and cleavage that indicate two periods of deformation. Detrital zircon ages (youngest of which peak at 591 Ma) and an ~470 Ma granite intrusion constrain the age of the Qiangtang Basement;
• Palaeozoic sedimentary rocks, that unconformably overlie the basement, and include Middle to Late Ordovician siltstone and slate, and Upper Carboniferous to Lower Permian flysch and sandstone, including glaciomarine deposits, all with a Gondwanan affinity (Zhao et al., 2014). Both the Ordovician and Carboniferous units are fossiliferous. These sedimentary rocks are not metamorphosed and only exhibit one deformation phase, expressed as folding, boudinage and cleavage development (Zhao et al., 2014). Consequently the first deformation phase seen in the basement must be pre-Ordovician, whilst the second is post-Carboniferous;
• tectonic/subduction mélange that forms the >>500 km long, east-west trending, Central Qiangtang Metamorphic Belt (Kapp et al., 2003; Zhang et al., 2006; Liu et al., 2011), also known as the Qiangtang Culmination or Anticlinorium. It is composed of strongly deformed lithologies, including high-pressure metamorphic rocks which include blueschists and eclogites, as well as subduction mélange containing ophiolitic fragments. These rocks are exposed in core-complex like structures in the footwall of Late Triassic to Early Jurassic, domal, low-angle, normal faults. The mélange is composed of a strongly deformed, schistose matrix of transposed metasedimentary and mafic schists that encloses lesser-deformed blocks of meta-mafic rocks, Carboniferous to Triassic meta-sedimentary rocks, and early Palaeozoic gneiss. Both the matrix and clasts have been subjected to greenschist, epidote-blue-schist, and locally, epidote-amphibolite facies metamorphism. This metamorphism took place at >10 kbar, whilst the maximum equilibration temperatures for mafic schists in the mélange matrix decrease from east to west, from 660°C at 89°E, to 425°C at 84°E. Structural, thermo-barometric, and 39Ar/40Ar age dating studies suggest the Qiangtang mélange was exhumed in an intra-continental setting from depths of >35 km to upper crustal levels in <12 Myr by Late Triassic to Early Jurassic crustal-scale normal faulting (Kapp et al., 2003). The hanging walls of the mélange capping faults is occupied by late Palaeozoic to early Mesozoic meta-sedimentary and crystalline rocks, similar to lithologies exposed in the footwall of the mélange.
Two hypotheses have been offered to explain the occurrence of the mélange, namely, that it is i). an accretionary wedge above the north-dipping subduction zone in which the oceanic crust, and then the following South Qinangtang continental block is subducted to the north below the North Qiangtang Block, followed by extension and exhumation (e.g., Li et al., 1995); or ii). an accretionary wedge and tectonic mélange overlying Triassic southward, flat subducting Songpan-Ganzi oceanic crust below the combined North and South Qiangtang terrane. This compressional event is interpreted to have been followed by exhumation to upper crustal levels during the Late Triassic to Early Jurassic as a core complex, resulting from local extension caused by roll-back of the subducting plate Kapp et al., 2000). The first option is consistent with the North and South Qinangtang terranes being of separate Cathaysian and Gondwanan affinity respectively, while the latter is more problematic with this observation. However, Pullen and Kapp (2016) note that 'recently', Carboniferous to Lower Permian strata have been found to the north of the Qiangtang Metamorphic Belt. Never-the-less, a detailed study of detrital zircons by Liu et al. (2022) indicated Palaeozoic to Triassic sedimentary successions rocks of the South Qiangtang Sub-terrane were derived from a Gondwanan source, whilst those from the North Qiangtang Sub-terrane were sourced from the Yangtze Block and from arc magmatic rocks, which were associated with the subduction of the intervening oceanic lithosphere below the North Qiangtang Sub-terrane. The same authors also note that the available data indicate that the northward subduction of this oceanic lithosphere was continuous, at least during the Late Devonian to Triassic, and that collision between the two subterranes was initiated at ∼225 Ma.
• Permian to Jurassic shallow marine deposits. This sequence begins with the Longge Group which contains abundant Middle Permian fauna and is composed of massive, poorly bedded reef or platform limestones including micritic bioclastic and sparry oolitic limestone, dolomicrite, and brecciated dolostone. These strata occur as large fault-bounded rafts within the mélange, with a maximum thickness of ∼ 5000 m. Late Permian to Middle Triassic rocks are absent from the South Qinangtang Terrane. Upper Triassic strata commenced with conglomerates to sandstones unconformably overlying Permian sedimentary rocks, as well as mélange (Li and Wen, 2007), followed by medium- to thick-bedded sandstone and shale, as well as coal and thin bedded limestone (Yin et al., 1988; Zhang et al., 2002; Ding et al., 2013). The Lower to Middle Jurassic succession consists of thin-bedded limestone and fine-grained clastic rocks, whilst the Upper Jurassic is characterised by oolitic limestone and interbedded sandstone (Zhang et al., 2002). The Lower Cretaceous stratigraphy includes conglomerate, sandstone and non-marine clastic rocks, and locally marine limestone along its limbs (Pan et al., 2004; Kapp et al., 2005; K.J. Zhang et al., 2012).
• Late Cretaceous to Cenozoic Basins. Following a depositional hiatus during the Lower Cretaceous, Upper Cretaceous and Cenozoic deposition on the South Qiangtang Terrane primarily comprised terrestrial clastic rocks, mostly conglomerates, deposited in elongate, fault-bounded, intermontane basins (Wang and Pan, 2012). These basins were similar to the Paleogene and Neogene intra-continental strike-slip extensional basins of the northern Jinshajiang-Ailaoshan-Red River Metallogenic Belt.
• Magmatic activity within the South Qiangtang Terrane is interpreted to have been caused by northward subduction of oceanic lithosphere beneath the terrane during the Permian to Triassic, forming the Early Mesozoic South (or West) Qinling Arc Accretionary Complex (e.g., Li et al., 2014; Li et al., 2015; Luo et al., 2015). These are dominated by andesitic‐dacitic‐rhyolitic rocks which have abundant mafic magmatic enclaves, and mainly belong to the high‐K to shoshonitic series, with weakly metaluminous characteristics. However, some quartz diorites to granodiorites with tonalitic enclaves have adakite‐like signatures. Two main episodes are indicated:
i). Early Episode between 245 and 235 Ma in the Mid-Triassic, predominantly comprising diorite‐granodiorite‐monzogranite with abundant mafic enclaves that predominate to the west. Most granitoids include hornblende‐bearing I‐type granite (Guo et al., 2012; Li et al., 2014; Li,
Huang, et al., 2015; Luo et al.., 2015), whilst some have adakite‐like signatures (Luo et al., 2015).
ii). Late Episode between 234 and 216 Ma in the Mid- to Upper-Triassic, which predominate to the east, and are composed of diorite, monzogranite and granitic porphyry, but lack associated coeval volcanic rocks (Cao et al., 2011; Zhu et al., 2011; Guo et al., 2012; Zhu, Zhang, et al., 2013). These granitoids have I‐type or A‐type affinities (Cao et al., 2011; Guo et al., 2012; Wang et al.,
2013; Zhu et al., 2011; Zhu, Zhang, et al., 2013).
The Bangong Co-Nujiang Suture Zone extends for over 2000 km across the central Tibetan Plateau, and separates the South Qiangtang Sub-terrane and Lhasa Terrane. It is characterised by scattered ophiolite fragments associated with thick sequences of Jurassic flysch, mélange, and volcanic rocks (Kapp et al., 2003).
The oldest basement rocks within the Bangong Co-Nujiang Suture Zone are variously described by different authors as Palaeoproterozoic (e.g., Song et al., 2023) or Neoproterozoic (dated at ~820 Ma; Zhang et al., 2012) and comprise metamorphic rocks that include gneiss, granitic gneiss, amphibolite, migmatite, magnetite quartzite, schist and marble. These rocks are predominantly exposed towards the eastern extremity of the east-west section of the suture zone within the core core the ~150 x 50 km Amdo Dome. They have been reworked in the Palaeozoic to produce an assemblage of blueschist, amphibolite, marble, quartz schist, quartzite and muscovite schist restricted to the Amdo Dome and as sporadic outcrops in the northern part of the neighbouring South Qiangtang Terrane (Zhang et al., 2012). Guynn et al. (2012) suggested that the intermediate-felsic orthogneisses from the Amdo basement represent two magmatic crystallisation events, namely Neoproterozoic, 920 to 820 Ma and Cambro-Ordovician 540 to 460 Ma. The Neoproterozoic and Palaeozoic orthogneisses are observed to typically be interlayered, with abundant veins parallel to the gneissic foliation (Zhang et al., 2012). The Early Palaeozoic gneisses are bimodal. In contrast, detrital zircon dating of the quartzite within the complex suggest the maximum depositional age of its protoliths to be Ordovician, although to be consistent with populations elsewhere in the region, they are most likely Carboniferous to Permian continental margin strata. Metamorphic overgrowth rims of zircons from all the meta-igneous rocks and meta-sedimentary rocks in the Amdo Dome examined by Zhang et al. (2012) and in other studies, yielded similar ages of 190 to 170 Ma, indicating all underwent the same Early Jurassic metamorphism. Zhang et al. (2012), interpreted these data to represent peak metamorphism to high-pressure granulite facies, followed by a retrogression to amphibolite facies, all during the Jurassic. The Amdo basement is also characterised by extensive Lower Jurassic 185 to 170 Ma granitoids, emplaced coeval with the metamorphism (Guynn et al., 2006, 2012; Zhang et al., 2012).
The Bangong Co-Nujiang Suture Zone is characterised by a broad belt of widely scattered, discontinuous, ophiolitic fragments that are associated with thick Jurassic to Early Cretaceous deep-marine flysch sequences, melange and volcanic rocks (e.g., Wang et al., 1983; Cheng and Xu, 1987; Yin et al., 1988). The width of this belt varies to as much as 200 km, prompting speculation as to whether it represents i). a single ophiolite sheet that was obducted southward from a north dipping subduction zone along the southern margin of the Qiangtang Terrane (e.g., Girardeau et al., 1984, 1985; Coward et al., 1988); ii). two or more oceanic plates separated by one or more intra-oceanic arc terranes (e.g., Mei et al., 1981; Srimal, 1986; Pearce and Deng, 1988; Hsu et al., 1995; Matte et al., 1996; Dunlap and Wysoczanski, 2002); or iii). alternatively, Song et al. (2023) and Zhou (2015) suggest closure of the Bangong-Nujiang Sea involved temporally overlapping double subduction, southward below the Lhasa Terrane during the Early Triassic to Early Cretaceous (Pan et al., 2006; Zhu et al., 2009; Zhu et al., 2009; Zhu et al., 2011), and northward beneath the South Qiangtang Sub-terrane from the Middle Triassic (Kapp et al., 2003; 2007; Guynn et al., 2006, Liu et al., 2022), respectively, with hard collision involving development of a complex mélange of folding, faulting and thrusting that juxtaposed fragments of both the ocean floor and overlying volcanic and sedimentary rocks.
Ophiolitic fragments include MORB-type, supra-subduction zone (Shi, 2007; Shi et al., 2005) and oceanic island-sea mountain basalt (Wang et al., 2005) and oceanic ridge plagiogranite (Zhang and Chen, 2007), with ages that range from 221.6 ±2.1 Ma in the Late Triassic to 167.0 ±1.4 Ma in the Early Triassic (Wang et al., 2008; Shi, 2007). To the SW of the Amdo Dome, they can be shown to have formed prior to deposition of the uppermost Jurassic and Lower Cretaceous sequences, whilst on the western extremity of the suture zone, such fragments are overlain by Lower Cretaceous, Aptian to Albian volcanic rocks and shallow marine limestones (Girardeau et al., 1985; Cheng and Xu, 1987; Matte et al., 1996). Overall, the ophiolitic rocks of the Bangong Suture are interpreted as having formed during the Late Triassic to Early Jurassic, and been obducted in the Middle to Late Jurassic, along with the overlying sequence deposited in the closing ocean (Girardeau et al., 1985; Bao et al., 1996; Matte et al., 1996; Wang et al., 2002; Lu et al., 2003, Pullen and Kapp, 2016). However, deep-marine deposition appears to have continued, at least locally, to the Middle Cretaceous (Smith and Xu, 1988; Baxter et al., 2009). During the Early Cretaceous, the large obducted mélange sheet was being shortened and progressively structurally stacked during the Lhasa-South Qiangtang collision. The collision resulted in the uplift and deposition of Middle Cretaceous post-collisional molasse, largely composed of terrestrial red-bed conglomerates from ~90 Ma, followed by older sequences being exhumed and ramped over those red beds, as well as Triassic sequences concordantly overlying Jurassic suites. Similarly in the western section of the suture zone, Late Cretaceous thrusting displaced Permian strata >20 km to structurally overlie Jurassic ophiolitic mélange and Cretaceous strata (Kapp et al., 2003).
Intrusive rocks within the Bangong Co-Nujiang Suture Zone were sparse during the Palaeozoic, with the bulk being emplaced in the Mesozoic and Cenozoic. These included a wide variety of granitoids, from quartz porphyry to diorite, dolerite, gabbro, plagiogranite and syenite porphyry. The Triassic intrusive rocks are confined to the northern part of the Bangong Co-Nujiang Mineral Belt, whilst Late Jurassic to Early Cretaceous magmatism is widespread in the belt, concentrated along the southern margin of the South Qiangtang Terrane, as well as in the central and western sections of the Lhasa Block. Three main pulses of Cretaceous magmatism are recognised: i). from 140 to 130 Ma; ii). 120 to 110 Ma, occurring on both sides of the suture zone, accompanied by the eruption of a wide range of volcanic rocks; and iii). ~90 Ma in the Late Cretaceous, mainly within the North Lhasa Belt (Wang et al., 2019; Lei et al., 2019). Other less intense magmatic events include Cenozoic intrusive and volcanic rocks at various times between 65 and 15 Ma, mainly distributed along the Central Lhasa Belt.
As with the intrusives, Palaeozoic volcanic rocks are less common in the Bangong Co-Nujiang Mineral Belt, whilst Mesozoic and Cenozoic volcanic rocks dominate. These mainly comprise relatively minor Jurassic dacite, rhyolite, andesite, basalt and tuff that are restricted to the southern part of the belt, whilst abundant Cretaceous andesite, basalt, rhyolite, dacite, trachyte, volcanic breccia, and tuff, prevail in the entire southern part of the belt.
The observations outlined above have been interpreted to support the following framework:
• The South Qiangtang Sub-terrane, closely followed by the Lhasa Terrane (together referred to as the Cimmerian Superterranes) were progressively detached and separated from the northern margin of Gondwana and began to migrate to the NNE across the Meso-Tethys Ocean towards Asia. This was accommodated, firstly, from ~240 to 165 Ma (e.g., Song et al., 2023) by the detachment of the South Qiangtang Sub-terrane from the Lhasa Terrane in the south. At that stage, the latter was still attached to, or was undergoing incipient rifting from, Gondwana. The separation of the two terranes led to the formation of the Bangong-Nujiang Ocean. Secondly, to the north, this migration was also accommodated by the northward subduction of the Meso-Tethys Ocean below the North Qiangtang Sub-terrane. This migration culminated in the collision and amalgamation of the North and South Qiangtang sub-terranes, separated by the Longmu Co-Shuanghu Suture Zone. Zhao (2015) suggests this collision occurred at ~220 to 215 Ma and initially involved the northward subduction of the Meso-Tethys Ocean below the North Qiangtang Sub-terrane, followed by a brief period of simultaneous south dipping subduction below the South Qiangtang Sub-terrane before a hard collision and sinking of the two detached slabs by 210 Ma.
• Following the closure, collision and amalgamation of the of the Longmu Co-Shuanghu Suture Zone, the Lhasa Terrane also began northward migration from Gondwana, closing the Bangong-Nujiang Ocean, with northward subduction below the South Qiangtang Sub-terrane as early as 220 Ma (Liu et al. 2022). A minor micro-continental block, represented by the basement core of the Amdo Dome was caught between the now amalgamated Qiangtang Terrane to the north and the approaching Lhasa Block. According to Li et al. (2019), the oceanic crust on the northern leading edge of the Amdo Dome was being subducted below the Qiangtang Terrane prior to the collision of those two blocks at ~190 Ma. This was followed by deep continental subduction of the Amdo basement beneath the Qiangtang Terrane to a depth of ~50 km and the development of high-pressure granulite-facies metamorphism at ~190 Ma (Zhang et al., 2012; 2014). Subsequent oceanic slab break-off is interpreted to have led to rebound and exhumation of the Amdo basement to mid-crustal levels of ~20 km, accompanied by retrograde amphibolite facies metamorphism by ~180 Ma. The trailing edge of the metamorphosed Amdo Basement was exposed at this stage and contributed detritus to late Early to early Middle Jurassic sedimentation. This subduction event was also responsible for the development of a continental magmatic arc on the southern margin of the Qiangtang Terrane, emplacing extensive ~185 to 170 Ma granitoids into that terrane and the Amdo basement Guynn et al. (2006).
• Song et al. (2023) interpret the Bangong-Nujiang Ocean, an arm of the Meso-Tethys Ocean, to have undergone north directed oceanic subduction below the Amdo and amalgamated Qiangtang Terrane between 165 and 145 Ma, that produced widespread Middle to Late Jurassic granitoids on the southern South Qiangtang Terrane, accompanied by deposition of a thick accretionary wedge and a sequence of Jurassic flysch, mélange and volcanic rocks within the ocean (Kapp et al., 2003). This persisted until the diachronous closure of the ocean from east to west between 145 and 100 Ma. This was accompanied by temporally overlapping southward subduction of the same ocean below the northern Lhasa Terrane where another magmatic arc formed. Subduction was followed by continent-continent collisional orogenesis, including continental subduction, slab and SCLM detachment from 100 to 65 Ma, and final post-orogenesis rebound and uplift from 65 Ma.
REGIONAL MINERALISATION
Song et al. (2023) interpret the spatial and temporal development and distribution of mineralisation within the Bangong Co-Nujiang Mineral Belt to have occurred as follows, relative to it's tectonic evolution:
• During the opening, between 240 and 165 Ma, of the Bangong-Nujiang Ocean that separated the South Qiangtang and Lhasa terranes, Cr-Ni mineralisation was deposited, mainly hosted in the ultramafic sections of the ophiolite complex preserved within the Bangong-Nujiang Suture Zone. These include the Dongqiao and Qielihu chromite; Bangonghu and Yugula Ni, and Yilashan Cr-Ni deposits. Dongqiao and Qielihu were mined during the 1950s while the others had defined resources in 2023. The Cr deposit are mainly composed of massive, disseminated, nodular and podiform chromite, with variable disseminated millerite, which coexists with magnetite, and occurs as layered and strip-shaped bodies. More than 130 such bodies that are >20 m long with >12% chromite have been delineated. The Dongqiao ore bodies, for example are hosted within dunite and harzburgite. The host sequence also includes lesser basaltic lava and mafic-ultramafic cumulate. The Yugula nickel deposit occurs within the ultramafic rock of the ophiolite suite on the southern margin of the Bangong-Nujiang Suture Zone.
• During the subduction of the Bangong-Nujiang oceanic plate beneath both the South Qiangtang and Lhasa terranes and the later continent-continent collisional orogenesis, between ~240 and 100 Ma, porphyry-epithermal Cu-Au, porphyry-skarn Cu, skarn hosted Fe and minor orogenic Au deposits were formed. The bulk of the mineralisation was emplaced between 165 and 100 Ma, with a younger pulse of a few porphyry-skarn Cu ±Mo and orogenic Au deposits from 100 to 65 Ma, as follows:
- Porphyry copper systems ± associated epithermal gold - mostly located on the southern margin of the South Qiangtang Terrane, including the Qingcaoshan, Jilong and Duolong ore clusters which are distributed over a 200 km NW-SE aligned interval. Some porphyry systems are located within the Bangong-Nujiang Suture Zone, e.g., the Saideng, Daneri and Wugacuo deposits, whilst others occur within the northern Lahsa Terrane, e.g., the Kenbale, Yazhuo, Yare and Xiongmei deposits. Copper is the dominant metal in these deposits, with subordinate to trace Au, Ag and Mo.
There is one giant deposit Tiegelongnan within the Duolong cluster, with >10 Mt of contained Cu at an average grade of 0.53% Cu (~1.8 Gt of ore), as well as four large deposits containing between 1 and 10 Mt of Cu, but with lower Cu grades i.e., Bolong (2.7 Mt Cu @ 0.33% Cu), Duobuza (2.5 Mt Cu @ 0.46% Cu), Nating-Dibaonamugang (1.5 Mt Ca @ 0.32% Cu) and Naruo (2.5 Mt Cu @ 0.38% Cu). These five large to giant porphyry Cu (Au, Ag) deposits, as well as the smaller Gaerqin, Sena and Nadun are all located within the generally SW-NE aligned 50 x 22 km Duolong cluster within the southern South Qiangtang Terrane.
In addition to these large to giant deposits, there are also four smaller porphyry style deposits with between 1 and 0.1 Mt of contained metal, namely Yare, Saideng, Emenri and Qingcaoshan, and 12 occurrence with <100 000 tonnes of Cu e.g., XiongmeiGaerqin. Several of the smaller occurrences have high grades of >1% Cu, e.g., Saideng (1.3% Cu), Emenri (1.25% Cu), Nagedingri (2.3% Cu) and Daneri (1.8% Cu). Some of the larger porphyry systems also contain significant gold, as at Tiegelongnan (~37 t), Bolong (~126 t), Nating (~118 t), Naruo (~80 t) and Duobuza (~70 t), with grades that vary from ~0.13 to 0.41 g/t Au at Tiegelongnan and Duobuza respectively. Gold is only found in restricted zones, presumably as epithermal overprints, in most of the porphyry systems, e.g., the resource at Tiegelongnan infers the gold resource is confined to 285 Mt of ore, whilst the porphyry resource comprises ~1.8 Gt of ore. The gold bearing systems are usually accompanied by elevated silver. Molybdenum is not significant in almost all deposits. In addition, several porphyry and epithermal base metal deposits within the Bangong Co-Nujiang Mineral Belt host Pb and Zn resources, e.g., the Bagong, Xianglong, Balong, Baobude and Yare deposits which are found in the Lhasa and Qingtiang terranes and the Bangong-Nujiang Suture Zone.
The porphyry style deposits of the Bangong Co-Nujiang Mineral Belt are predominantly associated with Cretaceous porphyry intrusions. The major syn-mineral intrusions at Xiongmei, Duobuza, Bolong, Naruo and Tiegelongnan are granodiorite and diorite porphyries. Yazhuo is related to diorite porphyry. Some of the deposits listed above do not have any associated intrusion, although mineralisation is hosted by porphyry-style alteration and veining. The alteration style varies from deposit to deposit, even within the same district, e.g., with the Duolong cluster, where the lower grade Duobuza and Bolong deposits are mainly only accompanied by potassic alteration (Zhu et al., 2012; Zhang et al., 2015), whereas the hosts to the higher-grade ores at Tiegelongnan are overprinted by advanced argillic and phyllic alteration. In contrast the low grade ores at the Nating deposit are hosted in propylitic altered rocks.
The Tiegelongnan, Nadun and Dibaonamugang deposits are the only three known to have undergone advanced argillic alteration and host high sulphidation epithermal mineralisation (Tang et al., 2014; Tang et al., 2016; Song et al., 2018; Yang et al., 2020).
The principal copper sulphide in these porphyry systems is chalcopyrite, although a few deposits have subordinate bornite. Hypogene high-sulphidation minerals such as digenite, covellite, enargite and tennantite are found in several deposits, such as Tiegelongnan and Nadun which have been subjected to advanced argillic alteration and host and high sulphidation epithermal mineralisation. Supergene Cu-sulphides, comprising covellite, digenite and chalcocite, as well as oxide assemblages which include malachite and azurite, are found in some deposits.
- Skarn altered copper and iron mineralisation - within the Bangong Co-Nujiang Mineral Belt, at least nine skarn altered copper resources have been defined (as of 2023), five of which including Gaobaojiayue, Kuga, Galale, Shelama and Balazha, individually contain >0.1 to ~0.3 Mt of Cu metal with grades of that vary from ~0.6% to 2.96% Cu at Balazha and Shesuo respectively. Some have associated gold mineralisation, e.g., Galale, Ga'erqiong and Shelama with grades at 2.8, 2.0 and 2.61 g/t Au, and 40, ~20 and ~4 tonnes of contained gold respectively. Sulphides within these skarn Cu deposits mainly comprise pyrite and chalcopyrite with minor bornite, sphalerite, molybdenite and pyrrhotite, with supergene covellite, chalcocite and the Cu-oxides azurite and malachite in some.
Iron skarns include Nixiong on the northern margin of the South Lhasa Belt, and Lunggar on the southern margin of the Central Lhasa Belt with resources of 150 Mt @ 55 to 66% Fe2O3 Total and 37 Mt @ 56% Fe2O3 Total respectively. The Caima and Fuye deposits on the southwestern margins of the South Qiangtang Terrane contain 11.5 Mt @ 43% to 68%, Fe2O3 Total and 9.1 Mt @ 57% to 63%,Fe2O3 Total, respectively. Magnetite and hematite are the major iron minerals in these deposits, which also contain minor sulphides, such as chalcopyrite, pyrite, bornite, galena and pyrrhotite.
Most Cu and Fe skarn alteration systems are accompanied by porphyritic intrusions with predominantly granodiorite, diorite and monzonite compositions intruding carbonate hosts.
• Orogenic gold mineralisation - resources have been estimated for three occurrences distributed over an ~1000 km interval from the western, central and eastern sections of the Bangong-Nujiang Suture Zone. These are, respectively, Dacha (or Wusuola) with 6.73 tonnes of contained gold in ore @ 3.54 g/t Au, Shangxu with 4.12 t @ 7.36 g/t Au and Zhagela with 23 t @ 8.9 g/t Au. These occurrences are predominantly hosted by Lower to Middle Jurassic sedimentary rocks, with gold occurring in massive to laminated quartz saddle and spur veins and as disseminations, usually as native gold. Associated hydrothermal alteration is characterised by muscovite, carbonate, sulphides and chlorite. Sulphides include pyrite, chalcopyrite, sphalerite, galena and marcasite, with minor arsenopyrite, pyrrhotite, tetrahedrite, digenite, bornite, millerite, gersdorffite and cobaltite (Fang et al., 2020).
• During the post-orogenic stage after 65 Ma, W-(Mo) mineralisation was generated. The more significant mineralised systems known as at 2023 are:
- Jiagang, with a resource of 18 900 tonnes of contained WO3 @ 1.12% WO3, hosted near the southern margin of the North Lhasa Sub-terrane. It is a quartz-vein wolframite deposit with associate, widely developed, greisen alteration (Ge et al., 2004; Wang et al., 2006).
- Jiaoxi with a resource of 39 100 t of contained WO3 @ 1.12% WO3. It is hosted within the western Bangong-Nujiang Suture Zone, and is a quartz-vein type tungsten deposit (Wang et al., 2018; Wang et al., 2019).
Both deposits hosted within monzonitic granite and surrounding country rocks. Quartz and white mica constitute the typical hydrothermal alteration assemblage, and the quartz veins also contain a minor molybdenite and bismuthinite.
MINERAL DEPOSIT DESCRIPTIONS
Tiegelongnan Porphyry Cu-Au Deposit (#Location: 32° 52' 17"N, 83° 29' 57"E)
The Tiegelongnan porphyry-epithermal Cu-(Au) deposit is located in central Tibet, ~80 km NW of Gêrzê and ~850 km NW to WNW of Lhasa. It was previously known as the Rongna or South Tiegelong deposit, and is situated towards the southern margin of the South Qiangtang Sub-terrane, within the Duolong Ore Cluster in the western section of the Bangong Co-Nujiang metallogenic belt. Field observations and and geochronology indicate that the porphyry mineralisation is closely related to ~123 to 120 Ma Early Cretaceous intermediate-felsic intrusions, whilst the epithermal ore and gangue mineral assemblage in the shallow central part of the deposit indicate the presence of a shallow high sulphide epithermal phase superimposed upon the deeper porphyry mineralisation.
Regional Setting - The Tiegelongnan deposit is situated towards the northeastern extremity of the generally NE-SW trending, ~50 x 22 km Duolong ore cluster (Songet al., 2014) and is on the northern side of the Bangong Co-Nujiang Suture Zone. The cluster has been interpreted to lie within a continental arc setting, on the NW margin of a large circular structure and within a large east-west uplifted block. This ore cluster is defined by at least eight zones of alteration and mineralisation that define a linear trend, near normal to the alignment of the Bangong-Nujiang Suture Zone, influenced by the intersection of east-west and NE-SW faulting. The most significant of these structures along which most of the deposit are centred, is a deep fault which trends NE-SW and is known as the F10 Fault. The northern extremity of the cluster contains a broader, ~4 km diameter knot formed by several more such altered and mineralised zones. The country rock is predominantly composed of Jurassic pelagic sedimentary rocks of the Yanshiping Group and lesser Cretaceous and Cenozoic continental volcanic rocks, conglomerate and sandstone, all of which overlie a Triassic carbonate sequence that is exposed to the east. The Triassic Riganpeicuo Group, dominated by marble and limestone, is the oldest exposed unit in the Duolong Belt. It is overlain by members of the Yanshiping Group, beginning with the Lower Jurassic Quse Formation which consists of interbedded sandstone and siltstone, with some units of chert and pillow basalt; and then by the Lower to Middle Jurassic Sewa Formation pelagic siltstone and sandstone. These rocks, which generally dip at 50 to 80°NW, are interpreted to constitute a metamorphosed accretionary complex that was formed by the north-dipping subduction of the Bangong Co-Nujiang oceanic crust below the Qiangtang Terrane (Li et al., 2011a; Yang Chao et al., 2014; Fang Xiang et al., 2015). The Sewa Formation is overlain by the NE-trending, fault-controlled, Lower Cretaceous Meiriqiecuo Formation dated at 111 Ma (Li, et al., 2011) island-arc volcanics with minor volcaniclastic rocks (Wang Qin et al., 2015; Sun Jia, 2015). This formation is, in turn, overlain by the Upper Cretaceous Abushan Formation, a post-collisional molasse sequence of conglomerate and sandstone.
The emplacement of igneous rocks is controlled by well developed regional structures, with mineralisation mainly associated with NE, east-west and NW trending structures (Li et al., 2012, 2013; Geng Quanru et al., 2011; Pan et al., 2012; Duan Zhiming et al., 2013). With the exception of minor gabbro, dolerite and pillow basalt in the Jurassic sedimentary succession, regional magmatism is mainly restricted to intermediate to felsic granitoids, which include Cu ore-hosting granodiorite/diorite/granite porphyries (She Hongquan et al.., 2009; Zhu et al., 2015b; Zhou et al., 2015; Duan Jilin et al., 2015). Lower Cretaceous continental arc andesite, andesite porphyry and dacite form the immediate post-mineral cover, and as such have been important to the preservation of the Cu deposits (Wang Qin et al., 2015; Lin et al., 2017).
Geology - Within the immediate deposit area, the oldest exposed rocks are the Jurassic Sewa Formation siltstone and sandstone, which are overlain by the post-mineral Meiriqiecuo Formation andesite and dacite, and then by the Abushan Formation molasse. Syn-mineral Cu-(Au) hosting intrusions at Tiegelongnan include diorite porphyry and granodiorite porphyry (Lin et al., 2017). The diorite porphyry has only been intersected in drill core as a small dyke, with no observed contact with the granodiorite porphyry. The latter is composed of 45 to 55% plagioclase, 25 to 30% quartz, 5 to 8% biotite and 15 to 20% amphibole, while the diorite porphyry contains 55 to 75% plagioclase and 25 to 30% amphibole, with <5% quartz. In addition, well-mineralised breccias containing sandstone clasts have been found deep in the granodiorite porphyry.
Mineralisation and Alteration - The largest Cu-(Au) mineralised zone at Tiegelongnan is generally tabular grading to funnel-shaped at depth, and is hosted by granodiorite porphyry. Metallic minerals include mainly pyrite, chalcopyrite and molybdenite, with digenite, bornite, covellite, enargite and hematite, as well as minor azurite, malachite, limonite, sphalerite and galena. Non-metallic rock forming and alteration minerals are predominately quartz, feldspar, biotite, sericite, chlorite, epidote, alunite, dickite and kaolinite, with minor calcite and anhydrite. Mineralisation occurs as disseminated, veinlet, breccia and stockwork styles, exhibiting euhedral-subhedral and metasomatic-residual corrosion textures. Chalcopyrite is commonly xenomorphic, molybdenite frequently occurs as rosettes or flakes, whilst pyrite is mainly present as metasomatic-residual grains and is replaced by Cu sulphides.
The hydrothermal alteration pattern includes potassic, propylitic, phyllic and advanced argillic zones. Potassic alteration is the earliest phase, represented by abundant hydrothermal biotite, deep in the granodiorite porphyry and surrounding rocks. Within the granodiorite porphyry, some amphibole phenocrysts are replaced by hydrothermal biotite, whilst some hydrothermal biotite or biotite-quartz veins intrude the wall rocks. This alteration is accompanied by disseminated and veinlet chalcopyrite, pyrite and molybdenite-quartz. The potassic phase was followed by propylitic alteration, characterised by abundant chlorite and epidote. Whilst much of the propylitic alteration has been obliterated by the later phyllic stage, traces can still be found in the diorite porphyry, distal to the main mineralised centre. Where preserved within the diorite porphyry, chlorite and epidote occur as veinlets or as pseudomorphs after plagioclase and amphibole, and are accompanied by disseminated and veinlet chalcopyrite, pyrite and hematite. Phyllic alteration is extensive, and is characterised by disseminated secondary quartz and sericite after feldspars. Advanced argillic alteration comprises abundant kaolinite, dickite, alunite and vuggy quartz. Both phyllic and advanced argillic alterations are spatially associated with the epithermal Cu- (Au) mineralisation, dominated by disseminated bornite, digenite and enargite.
In summary, five main hydrothermal/mineralisation stages have been recognised, as follows: Stage I: Potassic alteration with chalcopyrite-pyrite-molybdenite; Stage II: Propylitic alteration and chalcopyrite-pyrite-hematite; Stage III: Phyllic and subsequent advanced argillic alteration, accompanied by a bornite-digenite-enargite assemblage; Stage IV: Pyrite and minor sphalerite-galena veining; Stage V: Supergene limonite-azurite-malachite mineralisation. Stages I (porphyry) and III (epithermal), are the principal hypogene stages.
The Tiegelongnan diorite and granodiorite porphyries have been dated at ~123 Ma and 121 Ma respectively (Fang et al., 2015; Lin et al., 2017). The hydrothermal biotite (potassic alteration) yielded a well-defined plateau age of 121.1 ±0.6 Ma (Ar/Ar and Rb-Sr ages; Lin et al, 2017).
The total Cu ore resource is estimated to be more than 2 Gt @ 0.53% Cu (Lin, et al., 2017) and contains >10 Mt of copper metal (Song et al., 2023). The epithermal section of the deposit contains a resource of 37 t of gold at a grade of 0.13 g/t Au. Lin et al., 2017 quote a total Measured + Indicated + Inferred Mineral Resources of >2.089 Gt @ 0.53% Cu, 0.13 g/t Au.
This Tiegelongnan description is drawn from "Lin, B., Chen, Y., Tang, J., Wang, Q., Song, Y., Yang, C., Wang, W., He, W. and Zhang, L., 2017 - 40Ar/39Ar and Rb-Sr Ages of the Tiegelongnan Porphyry Cu-(Au) Deposit in the Bangong Co-Nujiang Metallogenic Belt of Tibet, China: Implication for Generation of Super-Large Deposit, 2017 - Acta Geologica Sinica (English Edition), v91, pp. 602-616."
Bolong Porphyry Cu-Au Deposit
The Bolong porphyry Cu-Au deposit is located ~12 km to the SW of the Tiegelongnan deposit, along the main Duolong ore cluster trend.
Regional Setting - See the Tiegelongnan description above.
Geology - The Bolong porphyry Cu-Au deposit is hosted by alternating beds of sandstone and siltstone of the Lower Jurassic Quse Formation and Lower Cretaceous, ~120 Ma, granodiorite porphyry stocks. WithIn the Bolong deposit, three stages of granodiorite porphyry intrusions are recognised. The first and second, GDP1 and GDP2 granodiorite porphyries respectively, have only been encountered in drill holes, while the third, GDP3, is exposed at surface within the Bolong deposit. GDP1 is truncated by GDP2, with some xenoliths of strongly potassic alteration GDP1 being evident within GDP2. All of these porphyries contain similar phenocrysts of plagioclase, hornblende, biotite and quartz. GDP1 contains ~40% phenocrysts set in a microcrystalline groundmass with pseudomorphs after ~5 to 10 mm euhedral plagioclase and up to 10 mm amphibole. GDP2 contains lesser phenocrysts, averaging ~20 to 30 vol.%, with smaller, ~3 to 5 mm pseudomorphs of plagioclase and 5 to 8 mm amphibole. The contents of biotite and amphibole are <5 vol.%. Plagioclase phenocrysts are euhedral and completely or partially replaced by sericite. Euhedral hornblende phenocrysts were first replaced by biotite that was subsequently chloritised with the separation of magnetite and some sulphide minerals, including chalcopyrite and pyrite. Primary biotite phenocrysts are mainly euhedral and only partly replaced by chlorite, whilst quartz phenocrysts are anhedral. GDP1 and GDP2are syn-mineral having undergone potassic alteration with associated Cu-Au mineralisation, and are partially overprinted by phyllic alteration. GDP3 stocks, contain 10% to 15 vol.% phenocrysts, mainly plagioclase. GDP3 is associated with strong phyllic, but not potassic alteration and pyrite mineralization, suggesting later emplacement.
The Bolong porphyry Cu-Au deposit has been eroded to expose phyllic alteration at surface, subdivided into:
• Weak phyllic alteration, characterised by sericite, quartz, chlorite and pyrite which is replaced by limonite near surface; and
• Strong phyllic alteration, which is also accompanied by quartz-pyrite (or limonite) and sericite ±chalcopyrite veins and coincides with to the centre of alteration within the Bolong porphyry system.
From the surface downwards in the centre of the alteration system at Bolong, the host rocks change from quartz sandstone of the Quse Formation, into GDP1 or GDP2, whilst the alteration type changes gradually from strong phyllic to potassic alteration, which is characterised by secondary biotite and K feldspar with some magnetite. Quartz-chalcopyrite ±magnetite veins with pink K feldspar alteration selvages are commonly associated with the potassic alteration zone. Along the contact between the quartz sandstone and the granodiorite porphyries, there are abundant quartz-pyrite ±chalcopyrite veins with sercite selvages, and sulphides mainly concentrated along the centrelines or walls of the veins. Propylitic alteration, with an assemblage characterised by chlorite, epidote, and calcite, is locally found at surface within the deposit.
Copper and gold are both closely associated with, and homogeneously distributed within, GDP1 and GDP2, although a large, continuous mineralised shell is developed primarily in the outer and upper parts of these intrusions. The main metallic mineral is chalcopyrite, which occurs i). along the centreline or contacts of quartz-pyrite-chalcopyrite ±sericite veins, ii). as patches in quartz-chalcopyrite ±magnetite veins, or iii). is disseminated in GDP1 and GDP2. Gold exhibits a close relationship with chalcopyrite and has a positive correlation with copper content. Molybdenite is scarce, mainly occurring as quartz-molybdenite ±pyrite ±sericite veinlets, suggesting the Mo and Cu mineralising events were simultaneous. The molybdenite content of the quartz-molybdenite veinlets is usually very low.
The total mineral resource is estimated to be more than 800 Mt @ 0.33% Cu, including ~650 Mt @ 0.2 g/t Au, based on a resource of ~2.7 Mt of contained copper metal at a grade of 0.33% Cu and 126 t of contained gold at a grade of 0.2 g/t Au (Song et al., 2023). However, according to Zhu et al. (2015), the deposit had reserves of 3.8 Mt of copper with an average grade of 0.5% Cu, and 150 tons of gold with an average grade of 0.2 g/t Au.
This Bolong description is drawn from "Zhu, X., Li, G., Chen, H., Ma, D. and Huang, H., 2015 - Zircon U-Pb, Molybdenite Re-Os and K-feldspar 40Ar/39Ar Dating of the Bolong Porphyry Cu-Au Deposit, Tibet, China; Resource Geology, v.65, pp. 122-135.
Naruo Porphyry Cu-Au Deposit
The Naruo porphyry Cu-Au deposit is located ~3 km to the NE of the Tiegelongnan deposit, along the main Duolong ore cluster trend.
Regional Setting - See the Tiegelongnan description above.
Geology - The geology of the deposit area is relatively simple and dominated by feldspar quartz sandstone and slate of the Middle to Late Jurassic Sewa Group and dacite and andesite of the Late Cretaceous Meiriqiecuo Group as detailed above in the Tiegelongnan description. Small areas of granodiorite porphyry is exposed in the NE and SW sections of the deposit, occurring as 'branch-like' or 'vein-like' shapes and intrude the feldspar-quartz sandstone of the Sewa Group. The granodiorite porphyry has been divided into two phases based on the presence or absence of mineralisation. The early porphyry, which is exposed in the centre of the deposit, is the main mineralised and altered phase with a zonal distribution surrounding the intrusion. The late phase is exposed in the south-western and north-western parts of the deposit and does not exhibit significant mineralisation or alteration. The NE-SW trending F10 and F11 faults pass through this area, with the F10 being the main ore-controlling structure at the deposit and Duolong ore cluster.
The mineralisation within the Naruo deposit has been grouped into three styles:
• veinlet-disseminated which are hosted in the centre of the deposit by granodiorite porphyry in which the disseminated sulphides form star-like patterns, accompanied by quartz sulphide veinlets;
• stockwork veining , distributed peripheral to the porphyry within feldspar-quartz sandstone countryrocks, the product of metasomatic fracture filling. Individual veins vary from 0.3 to 3 cm in thickness and are filled with a variety of sulphides, including pyrite, molybdenite and chalcopyrite;
• a crypto-explosion breccia which has the form of an upright, 200 m vertical by 150 m wide and 30 to 150 m thick cylinder, located in the southwestern part of the deposit, distal to and only partially overlapping the stockwork vein mineralisation. Clast within the breccia accounts for 50 to 70% of the total volume of the breccia and range from 0.5 to 10 cm across. They are composed of both feldspar-quartz sandstone and granodiorite porphyry with 'triangular, platy, elliptical or irregular shapes' (Ding et al., 2017) set in a matrix mainly composed of quartz, calcite, chlorite, epidote and sericite, with pyrite chalcopyrite and hematite, and minor galena and sphalerite. Barren granodiorite porphyry cuts the core of the breccia.
The principal metallic minerals of the deposit are chalcopyrite, pyrite, magnetite and hematite, with minor molybdenite, galena, sphalerite, covellite, and associated gold. Alteration associated with this mineralisation includes potassic, silicic, sericitic, chloritic and epidote rich assemblages. The central potassic core of the deposit has been overprinted by a phyllic phase and grades into a shallow propylitic periphery. No argillic alteration has been recorded. Four phases of mineralisation have been identified: i). quartz-feldspar-biotitechalcopyrite-pyrite-magnetite; ii). quartz-pyrite-sericitechalcopyrite; iii). quartz-chlorite-epidote-pyrite-hematite; and iv). quartz-molybdenite.
The total mineral resource is variously estimated to be 660 Mt @ 0.38% Cu (Lin et al., 2019; Ding et al., 2017), or ~2.51 Mt of contained copper metal at a grade of 0.38% Cu and 82 t of contained gold at a grade of 0.19 g/t Au (Song et al., 2023).
The information in this Naruo description is drawn from Ding et al., 2017 and Lin et al., 2019, as cited below
Duobuza Porphyry Cu-Au Deposit
Duobuza was the first of the porphyry Cu-Au deposits to be found in the Duolong ore cluster, when it was discovered in 2000 by the No.5 Geological Team, of the Tibet Bureau of Geology and Exploration. It is located ~8 km SW and ~4 km NE of the Tiegelongnan and Bolong porphyry Cu-Au deposits respectively, as described above.
Geology - In the immediate deposit area, the stratigraphy mainly comprises Middle Jurassic rocks of the Yanshiping Group, overlain by the Late Cretaceous Meiriqie and Cenozoic Kangtuo groups, partially masked by Quaternary cover. The Yanshiping Group is composed of interbedded volcaniclastic to littoral facies rocks, mainly arkose and siltstone with interbedded basalt and dacite, that are distributed over an east-west elongated area, and dip at 50 to 80°NW. The Meiriqie group contains volcaniclastic andesite rich in plagioclase and amphibole phenocrysts. The Yanshiping Group strata is intruded by mafic to intermediate hypabyssal rocks, mainly gabbro, dolerite porphyry, diorite, quartz-diorite, quartz-diorite porphyry and granodiorite porphyry, mostly as stocks and dykes.
A granodiorite porphyry stock is the principal mineralised intrusion. This intrusion is partially exposed over a 200 x 1000 m area in the northeastern section of the deposit area, and as an elliptical 300 x 200 m area to the SW, both of which are surrounded by a 1400 x 500 m zone of mineralisation. The degree and style of alteration, divide the volcanic sequence into pre- syn- and post-mineral basaltic andesites that are intruded by the mineralised granodiorite porphyry and was responsible for their propylitic alteration. The granodiorite porphyries and all of the volcanic rocks have geochemical characteristics of arc magmas (Li et al., 20O8); specifically a high-K, calc-alkaline series depleted in HFSE (i.e., Nb, Ta, Zr and Hf) and enriched in large ion lithophile (LILE) elements (e.g., Rb and Ba), ranging from basaltic rocks, with 49 to 53% SiO2, to granodioritic composition with 65 to 68% SiO2. The SHRIMP zircon U-Pb age of the mineralised granodiorite porphyry is 121.6 ±1.9 Ma (Li et al., 20O8) in the Early Cretaceous.
Alteration surrounding the deposit covers and area of ~10 km2, zoned outwards from the centre of the mineralised porphyry from potassic biotite, with a partially superimposed argillic overprint and adjacent propylitic zone. Phyllic alteration is not well developed, with sericite-quartz veins only found locally. Hydrothermal magnetite is abundant and is consistently associated with copper sulphides and gold. Dense micro-fracturing is developed within the deposit with a frequency as high as 60 and 500 per metre, with a mean ranging from 95 to 350 per metre, proportional to the grade of mineralisation. A series of thicker 0.5 to 1.5 cm thick veins developed concurrently with these high density fractures (Li et al. 2007; Li 2008). Based on the mineralogy and cross-cutting relationships, three main stages of veining have been proposed. Stage I: biotite (EB veins; Gustafson and Quiroga. 1995), K feldspar-biotite-chalcopyrite-quartz, quartz-chalcopyrite-magnetite (A veins; Gustafson and Hunt 1975), and quartz-magnetite-biotite-K feldspar veins, all of which fall within the 'potassic' alteration zone; Stage II: quartz-chalcopyrite veins, where the sulphide is concentrated in the vein centre (B veins; Gustafson and Hunt 1975), quartz-chalcopyrite-pyrite, quartz-pyrite and quartz-molybdenite-chalcopyrite veins in the 'moderate argillic' alteration zone; and Stage III: gypsum, quartz-pyrite, gypsum-chalcopyrite and calcite veins in the wall rock.
The northern section of the Duobuza mineralised system falls within the granodiorite porphyry, its contact zone with the wall rocks, and in the potassic and superimposed moderate argillic alteration zones. The deposit has been defined over a north-south width of 100 to 400 m, an east-west strike length of ~1400 m, and a vertical extent of ~500 m below surface. It dips at between 65 and 80° toward a direction of 200° (SSW).
Microscopic petrography indicates copper and gold mineralisation was predominantly deposited during the early potassic alteration stage. The principal hypogene minerals are chalcopyrite and magnetite, followed by pyrite, hematite, rutile, minor chalcocite, bornite and native gold. The amount of chalcopyrite is greater than bornite, whilst both exceed pyrite. Gangue minerals are K feldspar, albite, quartz, scricite, chlorite, carbonate, illite and gypsum. Only a single small molybdenite-quartz vein had been encountered in 2011 (Li et al., 2011). There is vertical mineralisation gradation from stockwork veinlets and disseminated copper that are more prevalent in the the upper part of the deposit, but decrease with depth. There appears to be a positive correlation between gold and copper (Li et al., 2007), without any systematic variation in the Cu/Au ratio with depth. A 70 to 100 m thick supergene enrichment zone has been defined surrounding the southeastern part of the deposit with an average grade of >1.17% Cu, and > 0.28 g/t Au, and a mineralogy that includes malachite, azurite, limonite, chalcocite, naive copper and cuprite.
The proven mineral resource at Duobuza is of 2.7 Mt of copper with a grade of 0.94% Cu, and 13 t of gold with a grade of 0.21 g/t Au, although it is inferred that the deposit could contain as much as 4 to 5 Mt Cu and 30 to 50 t Au (unpublished report by No.5 Geological Team, Tibet Bureau of Geology and Exploration in 2003).
The information in this Duobuza description is largely drawn from Li et al., 2011 as cited below
Nating and Dibaonamugang Porphyry Cu-Au Deposits
The Nating and adjacent Dibaonamugang deposits are both located within the large, southwestern most of the alteration zones of the Duolong ore cluster and are ~12 km SW of the Bolong deposit. Intrusive rocks include 121 to 118 Ma granodiorite porphyry and monzonitic granite porphyry intruding a sequence of Mid-Jurassic feldspar-quartz sandstone and silty slate. Dibaonamugang is the third of the trio of deposits within the Duolong ore cluster that have undergone advanced argillic alteration and high sulphidation epithermal mineralisation, the other two being Tiegelongnan and Nadun.
Nating has a resource of 1.5 Mt of contained copper and a grade of 0.32% Cu, and contains 118 tonnes of gold with a grade of 0.41 g/t Au (Song et al., 2023).
Little information has been encountered on the Nating and Dibaonamugang deposite, but what has been entered is largely drawn from Song et al., 2023 and Tang et al., 2021, as cited below
The most recent source geological information used to prepare this decription was dated: 2023.
This description is a summary from published sources, the chief of which are listed below. © Copyright Porter GeoConsultancy Pty Ltd. Unauthorised copying, reproduction, storage or dissemination prohibited.
Tiegelongnan
|
|
Ding, S., Chen, Y., Tang, J., Zheng, W., Lin, B. and Yang, C., 2017 - Petrogenesis and Tectonics of the Naruo Porphyry Cu(Au) Deposit Related Intrusion in the Duolong Area, Central Tibet: in Acta Geologica Sinica (English edition) v.91, pp. 581-601.
|
Hu, W.-L., Wang, Q., Tang, G.-J., Zhang, X.-Z., Qi, Y., Wang, J., Ma, Y.-M., Yang, Z.-Y.,Sun. P. and Hao, L.L., 2022 - Late Early Cretaceous magmatic constraints on the timing of closure of the Bangong-Nujiang Tethyan Ocean, Central Tibet: in Lithos v.461-417, doi.org/10.1016/j.lithos.2022.106648.
|
Kapp, P., Murphy, M.A., Yin, A., Harrison, T.M., Ding, L. and Guo, J., 2003 - Mesozoic and Cenozoic tectonic evolution of the Shiquanhe area of western Tibet: in Tectonics v.22, pp. 3-1 - 3-25. doi:10.1029/2001TC001332.
|
Kapp, P., Yin, A., Manning, C.E., Harrison, T.M. and Taylor, M.H., 2003 - Tectonic evolution of the early Mesozoic blueschist-bearing Qiangtang metamorphic belt, central Tibet: in Tectonics v.22, pp. 17-01 - 17-25. doi:10.1029/2002TC001383.
|
Li, C., Zeng, M., Chen, S., Jin, X. and Cheng, W., 2021 - Provenance evolution during passive- to active-margin transition unraveled from an accretionary complex from the Bangong-Nujiang suture zone: Insights into Early Mesozoic Meso-Tethys subduction and source-area tectonics: in Gondwana Research v.98, pp. 191-211. https://doi.org/10.1016/j.gr.2021.06.010.
|
Li, L., Garzione, C.N., Fan, M., Li, X. and Li, X., 2019 - Jurassic sedimentation in the south-central Qiangtang terrane reveals successive terrane collisions in central Tibet: in Geosphere v.15, pp. 433-449. doi.org/10.1130/GES01649.1
|
Li, S., Yin, C., Guilmette, C., Ding, L. and Zhang, J., 2019 - Birth and demise of the Bangong-Nujiang Tethyan Ocean: A review from the Gerze area of central Tibet: in Earth Science Reviews v.198, doi.org/10.1016/j.earscirev.2019.102907.
|
Lin, B., Chen, Y., Tang, J.-X., Wang, Q., Song, Y., Yang, C., Wang, W., He, W. and Zhang, L., 2017 - 40Ar/39Ar and Rb-Sr Ages of the Tiegelongnan Porphyry Cu-(Au) Deposit in the Bangong Co-Nujiang Metallogenic Belt of Tibet, China: Implication for Generation of Super-Large Deposit: in Acta Geologica Sinica (English edition) v.91, pp. 602-616.
|
Lin, B., Tang, J.-X., Chen, Y., Baker, M., Song, Y., Yang, H.-H., Wang, Q., He, W. and Liu, Z.-B., 2019 - Geology and geochronology of Naruo large porphyry-breccia Cu deposit in the Duolong district, Tibet: in Gondwana Research v.66, pp. 168-182. doi.org/10.1016/j.gr.2018.07.009.
|
Lin, B., Tang, J.-X., Chen, Y., Song, Y., Hall, G., Wang, Q., Yang, C., Fang, X., Duan, J.L., Yang, H.-H., Liu, Z.-B., J., Wang, Y.-Y. and Feng, J., 2016 - Geochronology and Genesis of the Tiegelongnan Porphyry Cu(Au) Deposit in Tibet: Evidence from U-Pb, Re-Os Dating and Hf, S, and H-O Isotopes: in Resource Geology v.67, pp. 1-21. doi:10.1111/rge.12113.
|
Pullen, A. and Kapp. P., 2014 - Mesozoic tectonic history and lithospheric structure of the Qiangtang terrane: Insights from the Qiangtang metamorphic belt, central Tibet: in Nie, J., Horton, B.K. and Hoke, G.D., (Eds.), 2014 Toward an Improved Understanding of Uplift Mechanisms and the Elevation History of the Tibetan Plateau: The Geological Society of America, Special Paper 507, pp. 7187, doi:10.1130/2014.2507(04).
|
Song, Y., Tang, J., Lin, B., Yang, C. and Sunm H., 2023 - Metallogeny in the Bangong-Nujiang belt, central Tibet, China: A review: in Frontiers in Earth Science, 19 April, 2023, 18p. doi: 10.3389/feart.2023.1139941.
|
Song, Y., Yang, C., Wei, S., Yang, H., Fang, X. and Lu, H., 2018 - Tectonic Control, Reconstruction and Preservation of the Tiegelongnan Porphyry and Epithermal Overprinting Cu (Au) Deposit, Central Tibet, China: in Minerals (MDPI) v.8, 17p. doi:10.3390/min8090398.
|
Tang, J., Yang, H., Song, Y., Wang, L., Liu, Z., Li, B., Lin, B., Peng, B., Wang, G., Zeng, Q.,Wang, Q., Chen, W., Wang, N., Li, Z., Li, Y., Li, Y., Li, H. and Lei, C., 2021 - The copper polymetallic deposits and resource potential in the Tibet Plateau: in China Geology v.1, pp. 1-16. doi:10.31035/cg2021016.
|
Xue, W., Hu, X., Garzanti, E., Ma, A., Lai, W. and Li, C., 2023 - Discriminating Qiangtang, Lhasa, and Himalayan sediment sources in the Tibetan Plateau by detrital-zircon U-Pb age and Hf isotope facies: in Earth Science Reviews v.236, 21p. doi.org/10.1016/j.earscirev.2022.104271.
|
Zhang, X.-N., Li, G.-M., Qin, K.-Z., Lehmann, B., Li, J.-X. and Zhao, J.-X., 2020 - Porphyry to epithermal transition at the Rongna Cu-(Au) deposit, Tibet: Insights from H-O isotopes and fluid inclusion analysis: in Ore Geology Reviews v.123, doi.org/10.1016/j.oregeorev.2020.103585.
|
Zhang, Z.-H., Dong, X., Liu, F., Lin, Y.-H., Yan, R. and Santosh, M., 2012 - Tectonic Evolution of the Amdo Terrane, Central Tibet: Petrochemistry and Zircon U-Pb Geochronology: in J. of Geol. v.120, pp. 431-451. DOI: 10.1086/665799.
|
Zhao, Z., 2015 - Tectonic evolution of the Qiangtang terrane, Central Tibetan Plateau: in Dissertation der Mathematisch-Naturwissenschaftlichen Fakultat der Eberhard Karls Universitat Tubingen zur Erlangung des Grades eines Doktors der Naturwissenschaften 172p.
|
Zhu, X., Li, G., Chen, H., Ma, D. and Huang, H., 2015 - Zircon U-Pb, Molybdenite Re-Os and K-feldspar 40Ar/39Ar Dating of the Bolong Porphyry Cu-Au Deposit, Tibet, China: in Resource Geology v.65, pp. 122-135. doi:10.1111/rge.12059.
|
Zhu, Z., Zhai, Q., Hu, P., Tang, Y., Wang, H., Wang, W. and Wu, H., 2022 - Resolving the timing of Lhasa-Qiangtang block collision: Evidence from the Lower Cretaceous Duoni Formation in the Baingoin foreland basin: in Palaeogeography, Palaeoclimatology, Palaeoecology, v.595, doi.org/10.1016/j.palaeo.2022.110956.
|
Porter GeoConsultancy Pty Ltd (PorterGeo) provides access to this database at no charge. It is largely based on scientific papers and reports in the public domain, and was current when the sources consulted were published. While PorterGeo endeavour to ensure the information was accurate at the time of compilation and subsequent updating, PorterGeo, its employees and servants: i). do not warrant, or make any representation regarding the use, or results of the use of the information contained herein as to its correctness, accuracy, currency, or otherwise; and ii). expressly disclaim all liability or responsibility to any person using the information or conclusions contained herein.
|
Top | Search Again | PGC Home | Terms & Conditions
|
|